Chapter 32 Biologic Response Modifiers
Interferons, Interleukins, Chemokines, and Hematopoietic Growth Factors∗
Biological Response Modifiers and Cytokines
Natural or synthetic preparations given with the intent of altering the response of the host to a pathogen, neoplasm, or inflammatory process have been termed biological response modifiers. The goal of therapy may be to increase the effectiveness of the immune response directed toward a pathogen or neoplasm, to stimulate the proliferation of hematopoietic progenitor cells (e.g., in the treatment of chemotherapy-associated neutropenia), or to decrease a chronic inflammatory response (e.g., chronic inflammatory bowel disease). Biological response modifiers are not usually specific in an immunologic sense; rather, they alter physiologic systems through changes in regulatory pathways. They range from bacterial cell wall extracts to molecularly cloned cytokines. As mediators of physiologic processes, interferons, interleukins, and chemokines are candidates for therapeutic manipulation or even for use as drugs. Several recombinant human, feline, and canine cytokines are commercially available in pharmacologic quantities and are available for veterinary use (Box 32-1). Some generalizations about cytokine biology follow. See the reviews by Oppenheim and Feldman,1 Nicola,2 and Oppenheim and coworkers3 for general discussions of cytokine, growth factor, and chemokine biology and the article by Gangur and coworkers4 for a review of chemokines of veterinary relevance.
Box 32-1 Commercially Available Recombinant Canine and Feline Cytokines, Chemokines, and Growth Factors
Cytokines are polypeptides that regulate cell growth and differentiation, apoptosis, inflammation, immunity, and repair. They are of fundamental importance in the pathogenesis and treatment of disease. They transmit information to target cells regarding the physiologic status of the animal, resulting in a biologic response in the target tissue. More than 50 distinct human cytokines and their receptors have been described. Cytokine nomenclature can be bewildering, with several historical names attached to the same molecule (e.g., stem cell factor, c-kit ligand, and mast cell growth factor are all the same cytokine). Cytokines Online Pathfinder Encyclopaedia (COPE) is a useful Internet resource with a large amount of information concerning cytokine, chemokine, and growth factor biology (www.copewithcytokines.de/cope.cgi). Cytokines can be categorized into families on the basis of homology in primary amino acid sequence, three-dimensional structure, induction mechanisms, chromosomal location, similarities in receptor type, and functional homology. Families that are currently clinically relevant in companion animal medicine include interferons (IFNs), which have antiviral and immunoregulatory functions; interleukins (ILs), which have a wide variety of functions; chemokines, which have to do with chemotaxis and organ development; and hematopoietic growth factors.
Cytokines typically have pleiotropic and redundant actions. The same activity may be induced by several structurally distinct cytokines acting at unrelated cell surface receptors. For example, IL-1, tumor necrosis factor-alpha (TNF-alpha),∗ and IL-6 are all mediators of the acute inflammatory response; induce fever and the synthesis of acute-phase proteins in the liver; increase vascular permeability; and induce adhesion molecules, fibroblast proliferation, platelet production, IL-6 and IL-8, and T and B cell activation. Gene deletion experiments reveal that few individual cytokines are absolutely essential to life or even to individual cell function, with notable exceptions such as TNF-alpha and transforming growth factor-β (TGF-β). Also, the cellular response of most cytokines is modulated by other cytokines, with synergistic, additive, and antagonistic interactions described. Cytokines form a complex feedback network by either inducing or suppressing the expression of other cytokines, forming cascades similar to the blood coagulation cascades.
It is tempting to administer cytokines in pharmacologic doses; however, several points should be considered. Perhaps most important, systemic levels of a given cytokine will perturb many cytokine cascades, with resultant side effects and toxicity. The use of IL-12 as a therapeutic agent, as discussed later, has been limited by potentially severe hematopoietic and hepatic toxicities in animals and man and was associated with two deaths in a phase 1 trial in humans.5 Dosing may be critical; for example, low doses of IFN appear to be immunostimulatory, whereas higher doses are immunosuppressive, and the specific type of IFN influences these effects. This concept of increasing doses of immunostimulatory cytokines leading to depression of the immune response has been demonstrated with IL-12 as well and appears to be due to the magnitiude of downstream IFN-γ and nitric oxide that is produced.6 Currently, recommended cytokine doses are empirically derived. With the exceptions of rfeIFN-α (in cats) and rhIL-1 (in dogs), the pharmacokinetics of cytokines in dogs and cats have not been well characterized.
Interferons
Interferon Biology
Two distinct classes of IFNs have been described. Class I interferons are subdivided into alpha, omega, and beta interferons (a subclass of omega interferons, the tau interferons, has been described in ruminant embryos and is important in maintaining pregnancy). Class II interferons are composed of a single protein, IFN-γ. IFN-α and IFN-ω were originally described as being secreted by leukocytes, but they are likely produced by all nucleated cells. Humans have at least 24 different alpha and omega genes, dogs have two alpha genes and no omega genes, and the genetic complement of cats has not been reported. Feline IFN-α cDNA has been cloned and the cytokine produced in a silkworm-recombinant baculovirus system, and its pharmacokinetic properties have been studied.7–10 The pharmacokinetic data suggest that rfeIFN has similar pharmacokinetic properties to human IFNs and that it is distributed primarily to the liver and kidneys, is rapidly catabolized mainly in the kidneys, and is excreted in the urine without residual accumulation in the body. Since feline IFN-α was initially cloned, 14 additional subtypes have been described.11,12 IFN-β is classically described as being produced by fibroblasts; however, many other cells can be stimulated to secrete the cytokine. Humans and dogs have one IFN-β gene. Synthesis of IFN-α and IFN-β can be induced by live or inactivated viruses, bacterial cell walls, synthetic oligonucleotides, IL-1, IL-2, and TNF-alpha. IFN-α and IFN-β compete for similar receptors. IFN-γ is produced by activated T and natural killer (NK) cells and is structurally and functionally distinct from IFN-α/β. All mammals investigated have only one IFN-γ gene. IFN-γ binds to a receptor distinct from the α/β receptor. Canine IFN-γ has been characterized, the cDNA and chromosomal gene have been cloned, and production and characterization of recombinant canine from Escherichia coli has been described.13–15 Feline IFN-γ cDNA has also been cloned.16,17 After receptor binding, IFNs induce the transcription of a set of genes called IFN-stimulated genes (ISGs). Nearly 30 different ISGs have been identified. Induction of ISGs by IFN-α/β is rapid and transient, lasting for 3 to 4 hours. IFN-γ requires several hours of exposure before gene induction occurs. After the initial induction, ISG transcription declines and returns to basal levels.
IFNs inhibit the growth of almost all known viruses by interfering with viral RNA and protein synthesis. IFNs are induced by viral nucleic acid and bind to the receptors of nearby cells. The development of resistance to virus infections occurs within a few minutes and peaks within a few hours. Several proteins are induced, including RNase L (which cleaves viral RNA); nitric oxide synthetase (nitric oxide has antiviral activity); a protein kinase that phosphorylates an initiation factor called elL-2 (which inhibits viral protein synthesis by preventing the elongation of viral double-stranded RNA); and the Mx protein, which inhibits translation of viral mRN.18 IFN-γ inhibits viral replication by stimulating the release of other IFNs. IFNs are also induced by bacteria, fungi, and some protozoa, and the activation of phagocytic cells is important in the host response to these pathogens.
Large-scale production of IFNs is accomplished by culturing stimulated cells, leading to the production of “natural” or “native” IFN (denoted by N) products. Alternatively, IFNs can be produced by recombinant methods. Human IFNs from both sources are commercially available, and recombinant feline and canine IFN-α and IFN-γ are commercially available (see Box 32-1). Natural IFNs are less concentrated and may contain a mixture of IFN types with other cytokines.
Interferons as Therapeutic Agents
In humans IFN-α has been approved for the treatment of hairy cell leukemia, melanoma, chronic myelogenous leukemia, Kaposi sarcoma, basal cell carcinoma, renal cell carcinoma, and genital warts. Effects on metastatic melanoma, endocrine–pancreatic tumors, metastatic colorectal and ovarian carcinoma, and bladder cancer have also been reported. IFN-β is also licensed for the treatment of chronic hepatitis B and hepatitis C infections. IFN-γ bound to polyethylene glycol (peg-IFN) has an increased circulating half-life and has shown improved efficacy in treating hepatitis C infections.19 IFN-β has been approved for the treatment of multiple sclerosis and IFN-γ for the treatment of chronic granulomatous disease. Experience using IFN therapy has generally shown that these products are most effective when combined with other antiviral or cytotoxic treatment modalities.20
Using a feline in vitro system, Weiss and Oostrom-Ram21 showed that low levels of rhIFN-α had no effect on lymphocyte blastogenesis, whereas higher levels significantly suppressed blastogenic responses. In vivo, cats given 102 or 104 IU/kg had significantly enhanced blastogenesis, whereas cats given 1 × 106 IU/kg had depressed lymphocyte stimulation. In cats the immunomodulating effects of rhIFN-α appeared to be dose dependent.
Five feline IFN-α subtypes have been recently cloned, expressed, and characterized in feline cell lines.11,22 All subtypes were shown to have antiviral effects on feline calicivirus and vesicular stomatitis virus in vitro. Additionally, they showed a species-specific antiproliferative effect on a feline tumor cell line. The IFN-α inducible Mx gene was upregulated 24 hours after administration to cats, demonstrating in vivo efficacy. In vitro work has also demonstrated the antiviral activity of IFN-α against feline herpesvirus-1 in the absence of any cytopathic effect on the cultured corneal cells.23 Activity of rfeIFN-ω, a closely related cytokine to rfeIFN-α against rotavirus, feline panleukopenia virus, feline calicivirus, and feline infectious peritonitis coronavirus, was documented in cell cultures of feline origin. The antiviral effect was more pronounced when the cell cultures were treated continuously than when they were pretreated only before challenge. RfeIFN-ω did not have activity in canine cells challenged with vesicular stomatitis virus, implying species specificity of action,24 yet de Mari and coworkers25 described clinical benefits of another rfeIFN-ω preparation in dogs with parvoviral infection (discussed later). Recombinant feline IFN-ω is marketed in Japan as IntercatR (Toray Industries, Tokyo, Japan), and a second rfeIFN-ω product is licensed in Europe as Virbagen omega (Laboratory Virbac, Carros, France).
Feline Infectious Peritonitis
Parenterally administered rhIFN-α and IFN-β, with or without Propionibacterium acnes (which enhances IFN responses and augments T and NK cell activities) given prophylactically or therapeutically, did not significantly reduce the mortality rate of experimentally induced feline infectious peritonitis virus (FIPV) infection. Cats treated with high-dose IFN, 10 U/kg daily for 8 days and then on alternate days for an additional 2 to 3 weeks, however, had temporary suppression of clinical signs and decreased serum antibody responses to FIPV, and the mean survival time of cats treated with high-dose rhIFN-α was increased by a few weeks over that of untreated cats. IFN-related toxicities were not reported.26 It is possible that the benefits of the high-dose protocol were due to the dose-dependent immunosuppressive effects of IFN. The increase in survival times in this study using experimental challenge was only 2 to 3 weeks, and there are few data documenting the response of cats with naturally occurring disease. There are anecdotal reports of orally administered low-dose rhIFN-α therapy (as described later) inducing remissions from clinical FIP, but there is currently no published data. A study of subcutaneous rfeIFN-ω administered to 12 cats clinically diagnosed with FIP demonstrated long-term survival in 4 animals.27 As the authors acknowledge, this was not a case-controlled study and histologic confirmation of the disease was available only for those animals that died during the study. A recent placebo-controlled, double-blind trial in which rfeIFN-ω was administered subcutaneously to cats naturally infected with FIPV did not demonstrate any clinical, hematopoietic, or survival benefits to the therapy; conventional therapy with cytotoxic drugs and corticosteroids remains the treatment of choice.28,29
Feline Leukemia Virus and Feline Immunodeficiency Virus
Recombinant hIFN-α has been shown to inhibit the production or release (or both) of feline leukemia virus (FeLV) in tissue culture systems.30 The parenteral administration of rhIFN-α alone or in combination with zidovudine (AZT) beginning 12 weeks after exposure to FeLV resulted in significant and sustained decreases in circulating virus levels. The anti-FeLV effect was limited by the production of anti–rhIFN-α antibodies detected 7 weeks after the start of therapy. IFN-associated toxicity was not observed.31 In a subsequent study, treatment of FeLV-infected cats with AZT, IFN-α, and adoptive transfer of lectin/IL-2–activated lymphocytes resulted in clearance of circulating virus in four of nine cats despite the appearance of anti-rhIFN-α antibodies. Combination therapy appeared to reconstitute antiviral humoral immunity, counteracted immunosuppression, and induced the reversal of retroviremia.32 Unfortunately, because of the problem of antibody induction, parenteral rhIFN-α appears to have little clinical utility as a monotherapy for FeLV infections. In vitro work supports the antiviral effects of rfeIFN-α and rhIFN-α but not rfeIFN-γ on feline immunodeficiency virus (FIV) replication.33 Ovine IFN-τ has demonstrable effects on the in vitro replication of both FIV and human immunodeficiency virus (HIV).34 A study of subcutaneous rfeIFN-α therapy in cats naturally infected with FeLV or co-infected with FeLV and FIV showed significant decreases in clinical scores and mortality rate during the 12 months of follow-up in the treated cats.35 Recently, low-dose oral IFN-α (as described later) was shown to improve clinical condition and survival in cats naturally infected with FIV.36
Canine Parvovirus
Two studies, one with experimental infection and one with naturally occurring infection, have examined the efficacy on rfeIFN-ω (Virbagen, Laboratory Virbac, Carros, France) on the outcome of canine parvoviral enteritis. Ten 8- to 9-week-old dogs inoculated with parvovirus were divided into rfeIFN-ω or placebo treatment groups and received three daily injections. All five of the placebo-treated dogs succumbed to fulminant enteritis, whereas four of five rfeIFN-ω–treated dogs survived and eventually recovered fully.37 In the second study of naturally occurring disease, 43 dogs were assigned to receive three daily intravenous injections of rfeIFN-ω, and 49 dogs received placebo treatment. There was a significant improvement in clinical signs and a significant decrease in mortality in the dogs receiving IFN therapy.25
Low-Dose Oral Recombinant Human Interferon-α
In 1988 Cummins and coworkers 38 reported that after experimental infection with the Rickard strain of FeLV, the administration of 0.5 or 5 U of natural hIFN-α orally once daily for 7 consecutive days on alternate weeks for 1 month was associated with survival in 70% of IFN-treated cats, whereas 100% of placebo-treated control cats died. However, 12 of 13 cats treated with IFN did develop persistent viremia. In another report, four cats with FeLV-associated nonregenerative anemia were treated with 100,000 U bovine IFN-β orally for 5 consecutive days on alternate weeks. General clinical improvement, reduction in circulating antigen levels, and normalization of hematocrit levels were reported in all cats,39 and one cat cleared its viremia. Similar findings were reported by Steed,40 who treated four FeLV-infected cats with low-dose natural hIFN-α or bovine IFN-β. Weiss and coworkers41 reported on 69 FeLV-infected cats with clinical signs treated orally with either low-dose rhIFN-α or bovine IFN-β. Cats treated with rhIFN had significantly higher survival rates than did cats given bovine IFN; both groups had increased survival rates compared with historical controls. In general, clinical responses were observed within the first or second week of oral IFN administration, with increased appetite, greater activity, weight gain, resolution of fever, improved hemogram and leukocyte counts, and quicker recovery from secondary bacterial infections when antibiotics were administered. Most cats remained viremic.
In contrast to the aforementioned reports, Kociba and coworkers42 reported that low-dose oral hIFN-α had no significant effects on viremia, course of the disease, or differential leukocyte counts in experimental FeLV infection. In their system the Kawakami–Theilen strain (A, B, and C subgroups, which consistently induce fatal erythroid aplasia) was administered to 12-week-old kittens. Methylprednisolone acetate was also given the day of inoculation. Neither rhIFN-α nor human natural IFN-α induced any significant benefit compared with placebo. A second study administering low-dose oral rhIFN-α showed a similar lack of benefit clinically or biochemically in naturally infected, clinically ill FeLV-positive cats.43
Pedretti and coworkers36 recently reported that FIV-infected cats given a daily oral dose of 10 IU/kg rhIFN-α had a significantly prolonged survival rate compared with placebo-treated cats. In addition, clinical remission of many of the typical immunopathologic lesions occurred despite no significant reduction in circulating viral levels. This study selected FIV-infected cats that presented with evidence of immunosuppression and clinical signs of acquired immunodeficiency syndrome (AIDS) and utilized a mixture of multiple human IFN-α subtypes. Two large, double-blind, placebo-controlled trials of low-dose oral IFN-α failed to show any efficacy in ameliorating clinical signs, increasing CD4+ T cell counts, or decreasing mortality rates in HIV-infected humans.44,45
Interferons as Therapy for Cancer
IFNs inhibit cell proliferation of both normal and malignant cells and have numerous immunomodulating effects. Several human cancers respond to IFN therapy. At present, there are only preclinical data suggesting that IFNs may have some utility in the treatment of cancer in companion animals. When canine mammary tumor and melanoma cell lines were incubated with canine IFN-γ, significantly increased expression of MHC antigen class I and II antigens and tumor-associated antigens were observed.46 Increased expression of these antigens may be of benefit in tumor cell recognition and rejection by the immune system. Pretreatment of canine macrophages with rcIFN-γ resulted in increased in vitro tumor cell killing in canine models of melanoma and osteosarcoma.47,48 In another study growth of canine and feline tumors was inhibited by rhIFN-α and rhIFN-γ in vitro. Sensitivity to IFN varied according to the type of neoplasm, with round cell tumors being most sensitive.49 Recombinant rfeIFN was found to have a dose-dependent inhibitory effect on cell growth and colony formation on cell lines derived from canine acanthomatous epulis, benign mixed mammary tumor, squamous cell carcinoma, and malignant melanoma.50 Recent work has demonstrated that intratumoral injection of rfeIFN-ω in cats with fibrosarcomas is safe and feasible; subsequent studies will be necessary to determine efficacy.51
Interleukins
Examples Of Interleukin Therapy in Dogs and Cats
Interleukin-1
Human rIL-1α was shown to be chemokinetic and chemotactic for canine neutrophils in vitro and to cause dose-dependent and selective neutrophil infiltration after intradermal administration.52 The pharmacokinetics of a single dose of human IL-1 has been studied in the dog. IL-1 was rapidly distributed, with a volume of distribution approximately twice that of the total body water of a lean dog. The terminal half-life was less than 30 minutes. Within approximately 1 hour after dosing,53 IL levels were below the quantifiable limit of the enzyme-linked immunosorbent assay (ELISA). As IL-1 is a central mediator of inflammation, these data are useful for studying the physiology of IL-1; however, pharmacologic efforts will focus on inhibition of IL-1 activity, with applications in the therapy of acute and chronic inflammatory disorders and septic shock. The canine IL-1 receptor antagonist, which functions as a competitive inhibitor of IL-1, has been cloned.54
Interleukin-2
IL-2 synthesis is triggered by antigen-induced activation of T lymphocytes, and its most important activity is the promotion of clonal expansion of antigen-specific T cells. In NK cells and macrophages, IL-2 also promotes proliferation, production of IFN-γ, and cytolytic activity. It also induces growth of B cells as well as immunoglobulin secretion. An important clinical consideration is the observation that NK cells cultured in the presence of IL-2 have enhanced cytotoxic activity, with increased capability for lysis of neoplastic cells. These activated cells are called lymphokine-activated killer (LAK) cells. IL-2 is thus an attractive agent for the therapy of neoplastic disorders; however, parenteral administration of IL-2 is associated with significant hepatic, hematopoietic, and vascular toxicity, which appears to be largely due to the secondary release of TNF-alpha and nitric oxide.55–57
Several in vitro studies have demonstrated that rhIL-2 has activity in companion animals similar to the activity recognized in humans. Feline lymphocytes responded appropriately to the cytokinetic action of systemically administered rhIL-2,58 and adoptive immunotherapy of FeLV-infected cats with lectin/rhIL-2–activated lymphocytes, IFN-α, and AZT led to reconstitution of antiviral humoral immunity, counteracted immunosuppression, and induced the reversal of retroviremia in a subset of treated cats.32 Feline IL-2 cDNA was cloned, and the recombinant protein was shown to promote proliferation of feline, but not human, cells.59,60 Helfand and coworkers61 demonstrated that the immunobiology of IL-2 in the dog is similar to that of humans. Tumor cytotoxicity was induced in vitro in canine lymphocytes with rhIL-2, demonstrating that functional and morphologic changes compatible with LAK cells could be obtained in dogs.62–64
Infusion of rhIL-2 into normal dogs resulted in lymphocytosis and enhanced in vitro lysis of a canine tumor cell line. Side effects included vomiting, diarrhea, and inactivity.65 Recombinant human TNF and rhIL-2 were administered in a sequential schedule to 30 dogs with a variety of spontaneous neoplasms. Objective tumor responses were seen in dogs with oral melanomas and cutaneous mast cell tumors. Dose-limiting toxicities were primarily gastrointestinal.66
In an effort to develop a delivery system that would be associated with less toxicity, Khanna and coworkers67 nebulized free rhIL-2 and rhIL-2–containing liposomes into normal dogs. Free IL-2 resulted in increased peripheral blood mononuclear cell activation compared with saline-treated control dogs. IL-2 liposomes resulted in significantly increased bronchoalveolar lavage (BAL) effector leukocyte numbers and activation compared with empty liposomes. In dogs with primary and metastatic lung cancer, nebulized IL-2 increased the total number of BAL macrophages, eosinophils, and lymphocytes compared with pretreatment levels, and there was increased expression of CD3 on BAL lymphocytes. Mean BAL cytolytic activity increased compared with pretreatment activity during therapy to a maximum at day 15, and then it decreased (despite continued aerosol therapy) to pretreatment levels at day 30. Antibodies reacting with hIL-2 developed in the serum of all treated dogs, possibly accounting for the decrease in BAL cytolytic activity at day 30 compared with day 15. Toxicity was not recognized with either IL-2 preparation. Complete regression of pulmonary metastases in two of five dogs with metastatic osteosarcoma was maintained at greater than 370 and 700 at day 68. A phase I trial of liposome-complexed canine IL-2 administered parenterally to dogs with chemotherapy-resistant, metastatic osteosarcoma was recently completed.69 The liposome formulation resulted in preferential expression of the IL-2 within the lung. The weekly injections were well tolerated, and 3 of the 14 dogs completing the 12-week therapy had partial or complete radiographic regression of metastases, with an additional four dogs showing stable disease. Despite the limitations in interpreting efficacy in a phase I pilot study, there was a significant increase in overall survival times in the treated dogs compared with historic controls with similar tumor staging.
Quintin-Colonna and coworkers70 reported that dogs with oral melanoma treated with local resection, 45-Gy radiation therapy, and repeated local injections of xenogeneic Vero cells transfected with an hIL-2–expressing plasmid had longer median survival times than did dogs treated with resection and radiation therapy alone; dogs treated with surgery, radiation therapy, and nonengineered Vero cells; or dogs treated with surgery, radiation therapy, and injection of rhIL-2 into the tumor bed. Similar results were seen in cats with fibrosarcomas. An unexpected observation was the development of metastatic fibrosarcoma in three of five cats that relapsed in the group treated with engineered cells. Complications seen in some dogs and cats included anaphylaxis associated with injection of Vero cells and local inflammatory reactions.70 Canarypox virus and attenuated vaccinia virus vectors have been used to deliver rfIL-2 and rhIL-2, respectively, to cats with soft tissue sarcomas.71 The injections were well tolerated, and there was a significant reduction in tumor recurrence rate with either of the virus delivery systems compared with controls 12 months after treatment.
IL-2 therapy appears to have a place in the immunotherapy of feline and canine cancers, particularly when administered in a manner that concentrates expression within the tumor, thereby limiting systemic toxicity. Adenoviral delivery and incorporation into liposomes appear to be particularly promising modes of therapy. Canine and feline IL-2 cDNA have been cloned59,72,73 and are commercially available (see Box 32-1).
Interleukin-12
IL-12 is a proinflammatory cytokine that has shown great promise as a therapeutic agent in experimental models of infectious disease and cancer. Multiple lines of evidence indicated that IL-12, a heterodimer produced in response to endotoxins, intracellular parasites, and CD40 ligation, is pivotal in initiating a cell-mediated immune response.74–76 Direct contact with microbial products or activated T cells can prompt antigen-presenting cells (e.g., macrophages, DCs) to release IL-12. In turn, IL-12 induces the synthesis of IFN-γ by T and NK cells. This early IL-12 generation is critical to early host containment of many intracellular pathogens, including Toxoplasma gondii,77 Listeria monocytogenes,78 Leishmania major,79 and Mycobacterium tuberculosis. 80 To date, most veterinary studies using IL-12 have been designed to characterize the adjuvant effect of IL-12 in boosting the cell-mediated immune response to FeLV, FIV, and FIPV vaccination. Two studies administering intradermal FIV DNA showed protection from FIV infection in three of four cats when IL-12 was included in the vaccination, whereas all four cats that received the DNA vaccine alone became infected.81,82 Similar DNA vaccinations protocols against FeLV challenge showed increased protection only when IL-12 was co-administered with IL-18, another cytokine known to enhance cell-mediated immunity.83 Vaccination of cats against FIPV in combination with co-delivery of feline IL-12 encoding plasmids did not enhance protection and may have actually potentiated the infection.84 There is precedence for the inhibition of cell-mediated immunity when IL-12 is administered at high doses,6,85 and this may have played a role in the outcome of this study. Lasarte and coworkers6 demonstrated that the depression of cell-mediated immunity at high IL-12 doses was related to the generation of large amounts of nitric oxide.
IL-12 is one of the most widely studied cytokines in cancer immunotherapy and has been found to eradicate experimental tumors, elicit long-term antitumor immunity,86–88 and possess antiangiogenic properties.89,90 The role of IL-12 as chemotherapeutic agent has been limited because of its toxicities. In mice, daily administration of 0.1 to 10 μg of recombinant murine IL-12 for up to 2 weeks resulted in liver function abnormalities; gastrointestinal toxicity; and hematopoietic changes, including anemia, neutropenia, lymphopenia, and thrombocytopenia.91–93 In humans the toxic effects include fever, anemia, neutropenia, lymphocytopenia, thrombocytopenia, liver function test abnormalities, rhinitis, stomatitis, and colitis.94 In a phase II clinical trial in renal carcinoma using rhIL-12, two deaths were reported.5 The authors have seen dose-dependent hematopoietic toxicities (anemia, neutropenia, and thrombocytopenia) in preclinical trials of systemic administration of a replication-defective adenovirus encoding murine IL-12 in cats.94a
One approach that can be used to avoid the systemic toxicity of IL-12 is to use local intratumoral IL-12 gene therapy. A replication-defective adenovirus containing feline IL-12 under the control of the heat-shock promoter has been developed and characterized in vitro.95 An added advantage of employing the heat-shock promoter is that IL-12 expression can be temporally induced by providing external hyperthermia to the tumor. A phase I dose escalation trial with feline soft tissue sarcomas was recently completed. A maximum tolerable dose was reached, although all cats completed the treatment regimen. At the highest dose of adenovirus, fever, anemia, and thrombocytopenia were reported.96 Further follow-up and expanded studies will be required to determine clinical efficacy of this cytokine treatment. Intramuscular injection of a plasmid DNA expression vector of canine IL-12 induced enhanced peripheral blood mononuclear cell production of IFN-γ in treated dogs.97 Recombinant mIL-12 has been shown to significantly inhibit the growth of a canine hemangiosarcoma cell line transplanted into mice.98 It is unclear whether the growth inhibition was mediated mainly through antiangiogenic mechanisms, NK cell activation, or a combination of both.
IL-12 is a potent immunostimulant that holds tremendous promise as a vaccine adjuvant or cancer immunotherapeutic. The very potency of this cytokine contributes to its limitations, and continued studies designed to diminish systemic toxicities will be necessary before it can be commonly employed. Both feline and canine IL-12 have been cloned and expressed,99,100 and the recombinant proteins are currently commercially available (see Box 32-1).
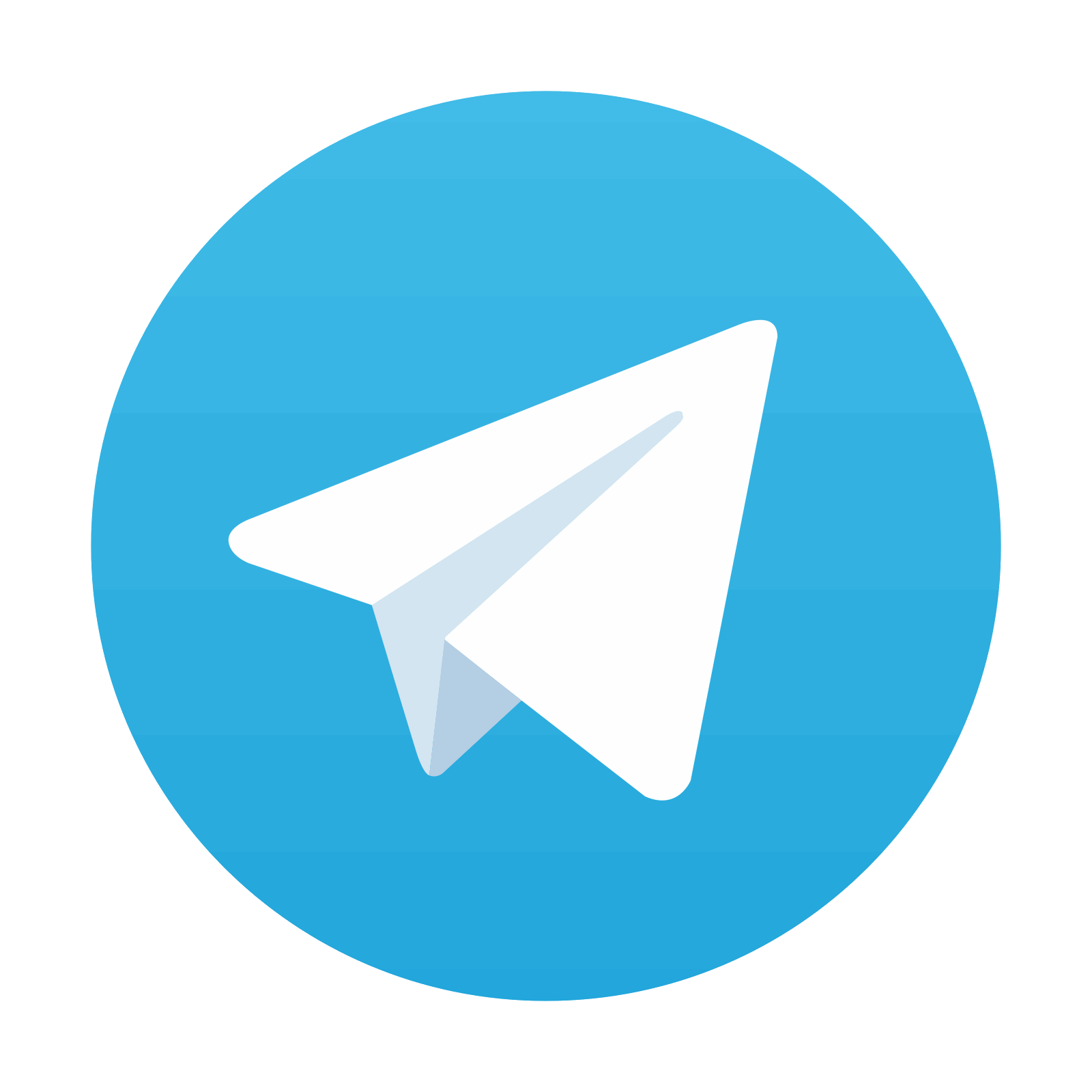
Stay updated, free articles. Join our Telegram channel

Full access? Get Clinical Tree
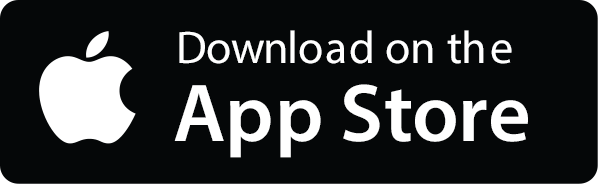
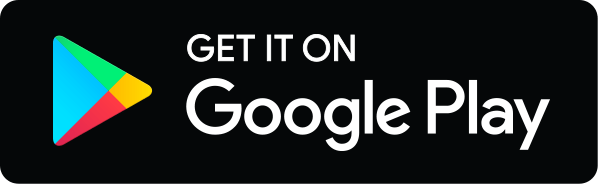