Hilary M. Clayton
Assessing English Saddle Fit in Performance Horses
The saddle acts as an interface between the rider and the horse, improving comfort and security during mounted equestrian sports. The underside of the saddle should conform to the shape of the horse’s back while the top of the saddle accommodates the very different shape of the rider’s pelvis and thighs. A saddle that fits the horse poorly is potentially a source of pain or discomfort that may be manifest in a variety of signs, ranging from aversion to being saddled to dangerous behavior when being ridden.
Anatomy of a Saddle
English saddles are built on a somewhat rigid tree (Figure 27-1) that distributes the rider’s weight over a large area of the horse’s back. However, the inherent rigidity of the tree can cause localized pressure points if it is not correctly fitted to the individual horse. The arch, also known as the gullet plate or head plate, supports the pommel, and the points extend downward from the arch to stabilize the front of the saddle across the withers. The angle of divergence of the points should match the outward slope of the horse’s shoulders, and the points should be long enough to stabilize the front of the saddle (Figure 27-2; see Figure 27-1). The bars are the support mechanism for the stirrup leathers, and the cantle underlies the seat.
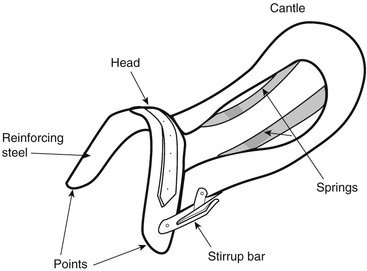
The tree gives attachment to the leather exterior, which varies greatly in shape and size depending on horse and rider morphology and the sport they participate in. Dressage saddles typically have a straight front edge of the flaps to accommodate the relatively extended hip angle and vertical thigh of the dressage rider (Figure 27-3). Jumping saddles have a more rounded flap to accommodate the flexed hip angle and forward position of the rider’s knee for jumping. The panels, which are attached to the underside of the saddle (see Figure 27-3), provide large and symmetric weight-bearing surfaces over the epaxial musculature on each side of the horse’s back. The left and right panels are separated on the midline by a gullet that should be high and wide enough to ensure that there is no direct pressure on the vertebral spinous processes (see Figure 27-3).
Saddle Force and Pressure
The horse’s vertebrae are united by ligaments, tendons, and muscles that provide passive and active support to the intervertebral joints. Forces generated by the soft tissues load the vertebrae in compression, even in quadrupeds that have a horizontally oriented vertebral column. The thoracolumbar spine acts as a beam suspended at each end by the forelimbs and hindlimbs. When the weight of a rider is placed on the horse’s back, it causes some extension of the intervertebral joints, which has the effect of hollowing the back. The degree of hollowing is correlated with the weight of the rider. During locomotion, the thoracolumbar spine moves in a predictable manner within each gait. In walking, there is considerable lateral bending of the back, with the spine bending away from the protracting hindlimb. In the trot and canter, the back flexes in the suspension phases and is extended in the stance phases as a consequence of gravitational and inertial effects on the large visceral mass. The presence of a rider is associated with greater extension of the intervertebral joints throughout the entire movement cycle, but the range of spinal motion remains unchanged. The fact that the intervertebral joints are more extended brings the spinous processes closer together, and in horses that have narrow interspinous spaces, increases the likelihood of impingement (kissing spines) when the horse is ridden.
When the horse is standing still, the combined weight of the rider and saddle determines the total force on the horse’s back. During locomotion, the total force changes through the stride in a cyclic, gait-specific pattern as a consequence of the movements of the horse’s limbs and trunk, the effect of muscular contractions causing localized changes in shape of the horse’s back, and the movements of the rider.
The availability of electronic pressure mats has provided information about the force on the horse’s back and the pressure distribution patterns in each gait. In walking and trotting, which are symmetric gaits, force and pressure are similar on the left and right sides of the horse’s back. In the walk, a graph of total force reveals small oscillations around the weight of the rider plus saddle weight (Figure 27-4) with six peaks and troughs per stride. Maximal force during walking is only slightly greater than the rider plus saddle weight. In trot, the total force shows two large oscillations per stride, with the horse’s back being unloaded in the suspension phases when the rider is projected upward and unloads the saddle and maximally loaded during the diagonal stance phases when the rider descends into the saddle. The maximal force is approximately double the weight of the rider plus saddle, and it is applied twice per stride. In the canter, the total force has a single large oscillation in each stride, with the horse’s back being unweighted in the suspension phase and maximally weighted in the middle of the stance phase. The maximal force at the canter is two and a half to three times the weight of the rider plus saddle and is applied once per stride. Thus the force on the horse’s back is considerably higher at the trot and the canter than at the walk, and the weight of the rider determines both the maximal force on the horse’s back and the amount of extension of the intervertebral joints. The pressure distribution, which can be measured using an electronic pressure mat (see Color Plate 27-1), also changes in a characteristic cyclic fashion during each gait, and evaluation of pressure scans may be helpful in localizing high-pressure areas (see Color Plate 27-1).
Effects of High Pressure
The saddle applies pressure to the skin and underlying tissues. Tissue ischemia may develop if saddle pressure exceeds capillary pressure, which is of the order of 4.7 kPa, with muscle tissue being particularly susceptible to pressure-induced ischemic damage. There is an inverse relationship between magnitude of pressure and the duration for which that pressure must be exerted in order to cause a pressure sore. Although pressures in excess of capillary pressure are frequently present on the horse’s back during riding, there is a cyclic pattern of loading and unloading so that the capillaries are not under continuous compression. Thus pressures considerably higher than capillary pressure may be tolerated as long as they are applied intermittently. Pressure-induced tissue damage is highly specific to body region, the nature of the interface, and the magnitude and duration of the pressure applied. For example, lower pressures are needed to cause clinical signs of back pain or saddle sores on the dorsum of the back compared with over the withers.
The horse’s back usually sweats under the saddle, and focal areas of dryness indicative of sweat gland ischemia occur in areas of pressure concentration. Even higher pressures can be associated with clinical signs of saddle sores (heat, swelling, pain on palpation). Based on research studies of the amount of pressure associated with these lesions, threshold values for mean pressure lower than 11 kPa and maximal pressure lower than 30 kPa have been recommended for horses ridden at the sitting trot.
Many of the lesions that develop beneath the saddle are friction rubs rather than true pressure sores. These result from movement between the saddle or pad and the horse’s skin. The first sign may be that the hair is broken or rubbed off, with the damage progressing to abrasion of the skin if the rubbing persists. The site of a former friction rub or a pressure sore is often permanently marked by the presence of white hairs that indicate hair follicle damage.
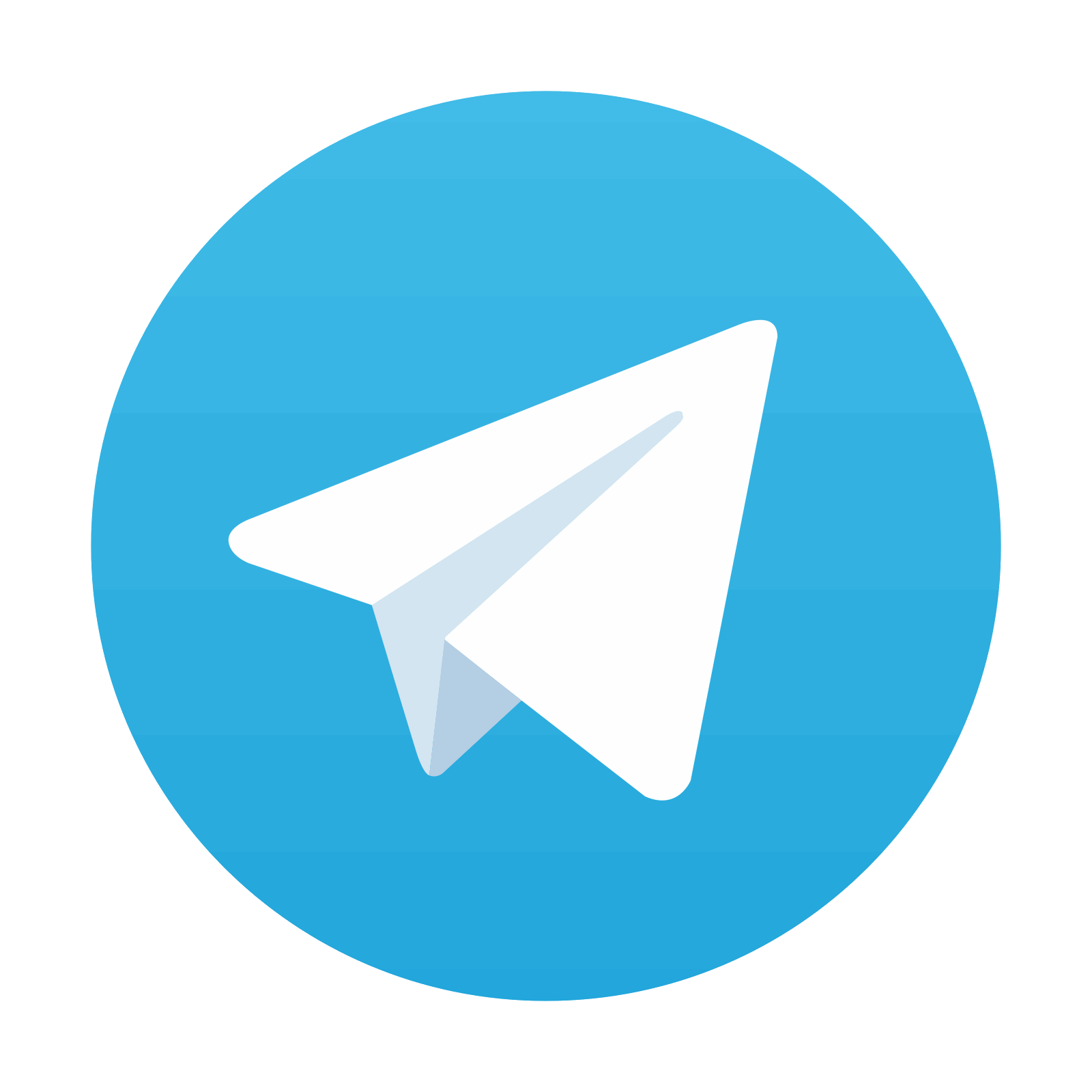
Stay updated, free articles. Join our Telegram channel

Full access? Get Clinical Tree
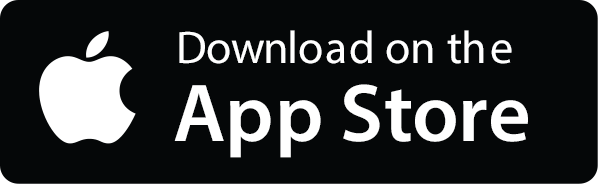
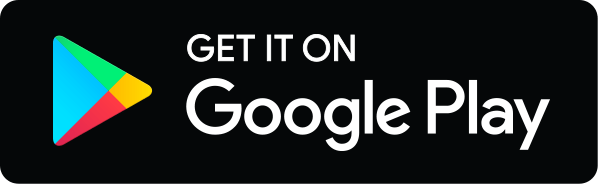