CHAPTER 71 Antimicrobial Therapy
MICROBIAL SUSCEPTIBILITY
Streptococcus and Pasteurella
These bacteria are consistently susceptible to β-lactam antibiotics such as the penicillins and cephalosporins. Resistance among Streptococcus spp. to trimethoprim-sulfonamide (TMS) combinations and chloramphenicol appears to be increasing. Trimethoprim-sulfonamide combinations for the horse may include either trimethoprim-sulfadiazine or trimethoprim-sulfamethoxazole; these drugs are also referred to as “potentiated sulfonamides”. (See Appendix C for dosing information.) Many of the fluoroquinolones (e.g., enrofloxacin, orbifloxacin, marbofloxacin) used in veterinary medicine have low activity which is reflected in high minimum inhibitory (MIC) values against Streptococcus spp., whereas Pasteurella organisms are frequently susceptible to fluoroquinolones as well as other drugs. Aminoglycosides have good activity against Pasteurella spp., but against streptococci, they should be combined with a penicillin.
Actinobacillus
Actinobacillus spp. have historically been susceptible to many of the β-lactam antibiotics and the potentiated sulfonamides, although resistance has been documented in the last several years. One report of postoperative wound infection showed that 100% and 60% of the isolates were resistant to penicillin and TMS, respectively.1 This resistance to penicillin may be caused by the production of β-lactamases by some strains.2 Susceptibility to the cephalosporins and aminoglycoside antibiotics is usually anticipated.
Staphylococcus
Recent reports have raised concerns of staphylococcal resistance in horses.3–5 The isolated methicillin-resistant Staphylococcus aureus (MRSA) strains colonized both horses and people who were in contact with the horses (see Chapter 44). Evidence for human-to-animal transmission was reported. These strains were resistant to other antibiotics, in addition to β-lactams. MRSA has been reported more often in some referral centers. These MRSA strains present an important problem for veterinarians because they are resistant to all β-lactam antibiotics, regardless of whether they are combined with a β-lactamase inhibitor. Some of these strains remain sensitive to fluoroquinolones and TMS, but there may be cases for which the only active drugs are the glycopeptide vancomycin or the oxyzolidinone linezolid (Zyvox). Vancomycin has been used sporadically in the treatment of equine MRSA as an intravenous (IV) infusion at doses of 4.3 to 7.5 mg/kg body weight every 8 hours (q8h).6,7 There are no reports of clinical use of linezolid in horses, and at the time of this writing, its use is considered to be cost prohibitive. (Linezolid is a human drug, and current retail costs exceed $55 per tablet.)
Anaerobic Bacteria
If the bacteria are anaerobic, predictable susceptibility patterns also are available. In horses, anaerobic bacteria causing infection include Clostridium, Fusobacterium, Peptostreptococcus, and Bacteroides spp.8 These bacteria are usually sensitive to penicillin, chloramphenicol, metronidazole, or one of the second-generation cephalosporins, such as cefotetan or cefoxitin. If the anaerobe is from the Bacteroides fragilis group, resistance may be more of a problem because these organisms can produce a β-lactamase that inactivates first-generation cephalosporins, penicillins, and ampicillin/amoxicillin. The incidence of resistant strains of Bacteroides has increased in recent years.9 Because many anaerobic infections in horses may be caused by B. fragilis,10 metronidazole is a logical choice for treatment. This drug is consistently active against anaerobes, including B. fragilis, and doses have been established from pharmacokinetic studies. Chloramphenicol also has consistent activity against many anaerobic bacteria. Clindamycin frequently has good activity against anaerobic bacteria, although resistance has increased over the last several years. However, clindamycin should never be used in the horse because of the likely development of a severe, often fatal, diarrhea. The activity of first-generation cephalosporins, TMS, or fluoroquinolones against anaerobic bacteria is unpredictable. None of the aminoglycosides is active against anaerobic bacteria.
Pseudomonas, Enterobacter, Klebsiella, and E. coli
If the organism is Pseudomonas aeruginosa, Enterobacter, Klebsiella, Escherichia coli, or Proteus, resistance to many common antibiotics is possible, and a susceptibility test is advised. Many E. coli isolates are resistant to common antibiotics such as penicillins, aminopenicillins, first-generation cephalosporins, and tetracyclines. Based on susceptibility data, gram-negative enteric bacteria are usually expected to be susceptible to fluoroquinolones and aminoglycosides. However, some reports suggest that resistance to fluoroquinolones may be increasing in small animals.11,12 Such resistance trends have not been reported for isolates from horses. Resistance to gentamicin among equine pathogens is increasing and has been documented in veterinary teaching hospitals for more than 15 years.13 Amikacin is the most active of the aminoglycosides against gram-negative bacteria in horses, including P. aeruginosa, and may be more suitable for the treatment of resistant gram-negative infections. P. aeruginosa is inherently resistant to many drugs, but it may be susceptible to fluoroquinolones, aminoglycosides, or extended-spectrum penicillins (e.g., ticarcillin, piperacillin). If a fluoroquinolone is used to treat P. aeruginosa, a large dose is necessary because the MICs of Pseudomonas spp. are higher than for other gram-negative organisms. Although pharmacokinetic studies have documented effective plasma concentrations for most gram-negative bacteria from typical doses of fluoroquinolones, no studies have used high enough doses to produce plasma concentrations considered to be effective against Pseudomonas spp. Moreover, the high doses recommended for treating Pseudomonas in dogs have not been tested for safety in clinical studies in horses. Of the currently available fluoroquinolones (human or veterinary drugs), ciprofloxacin is the most active against P. aeruginosa, but it is not absorbed well orally in horses.14
Fungi
Systemic fungal infections in horses can be difficult to treat because of the lack of availability of affordable treatments and the difficulty involved in culturing and identifying the organisms. However, some generalizations about susceptibilities can be made. Many of the yeast and yeastlike infections in horses have good susceptibility to the triazole antifungals—fluconazole, itraconazole, and voriconazole—including the organisms that cause candidiasis, histoplasmosis, blastomycosis, and coccidioidomycosis. Fluconazole is a rational treatment for these pathogens because it has excellent oral bioavailability and produces sustained plasma and tissue concentrations.15 This drug is now available in a generic human formulation, which has decreased its cost for effective therapy. Some Candida spp. have developed resistance to fluconazole however, and alternative therapies may be necessary. Aspergillus spp. are typically sensitive to itraconazole and voriconazole, but not to fluconazole. Fusarium spp. present a very difficult treatment dilemma in that they are resistant to many of the available drugs. Only voriconazole and amphotericin B demonstrate significant in vitro antifungal activity against Fusarium spp. If systemic treatment for dermatophytosis is required, griseofulvin has been used because it is labeled for use in the horse.
BACTERIAL SUSCEPTIBILITY TESTING
Agar Disk Diffusion Test
When bacterial resistance is likely, a susceptibility test is recommended. Bacterial susceptibility to drugs has traditionally been tested with the agar disk diffusion (ADD) test, also known as the Kirby-Bauer test. With this test, paper disks impregnated with the drug are placed on an agar plate and the drug diffuses into the agar. The zone of inhibition around the disk is correlated to the bactericidal or bacteriostatic activity of the drug against the bacteria. The ADD must be performed according to strict procedural standards for inoculation size, depth of agar, and incubation time set by the Clinical and Laboratory Standards Institute (CLSI), formerly known as the National Committee for Clinical Laboratory Standards (NCCLS).16,17 The ADD test results are qualitative and determine only resistance or sensitivity for the bacteria tested. If this test is performed using standardized procedures, it is valuable; at times, however, it may overestimate the degree of susceptibility.
Minimum Inhibitory Concentration Determination
When the MIC is measured, resistance and susceptibility are determined by comparing the organism’s MIC to the drug’s breakpoint, as standardized by CLSI.16–18 If bacteria have an MIC equal to or below the “susceptible” breakpoint, treatment with this drug should produce a cure unless there are other factors independent of the drug’s activity. An MIC equal to or above the “resistant” breakpoint indicates that the organism is resistant regardless of the dose administered or location of the infection. An MIC in the “intermediate” range means that the organism is resistant to the drug unless dosing modifications are used, or unless the drug concentrates at the site of infection, as with topical treatment, or in the lower urinary tract for drugs excreted via the kidney.
Even though we believe that an MIC determination is valuable to guide therapy, some limitations exist. One important limitation for interpreting susceptibility information for pathogens infecting horses is that interpretive criteria to establish susceptibility breakpoints are available for only a small number of drugs used in horses (ceftiofur, gentamicn) (See Tables 1 and 2 in the CLSI M-31 document under Group A.18) For other drugs, human interpretive criteria are used.
PHARMACOKINETIC-PHARMACODYNAMIC OPTIMIZATION OF DOSES
To achieve a cure, the drug concentration at the site of the infection should be maintained above the MIC, or some multiple of the MIC, for at least a portion of the dose interval. Antibacterial dosage regimens are based on this assumption. (See Appendix C.) However, drugs vary with respect to the magnitude of the peak concentration and the time above the MIC that is needed for a clinical cure. Pharmacokinetic-pharmacodynamic (PK-PD) relationships of antibiotics attempt to describe how these factors can correlate with clinical outcome.19,20 Parameters that describe the plasma concentration versus time profile may be used as pharmacokinetic factors to predict antibiotic cures (Fig. 71-1). The “Cmax” is simply the maximum plasma concentration attained during a dosing interval. The Cmax is related to the MIC by the Cmax/MIC ratio. The “AUC” is the total area under the curve. The AUC for a 24-hour period is related to the MIC value by the AUC24/MIC ratio. (Some authors refer to this as the “AUIC,” but this term has other meanings, and AUIC should not be used.) The duration of effective plasma concentrations is determined by the time above MIC measured in hours (T>MIC, or T>MIC), or reported as the percent of the time above the MIC during a 24-hour dosing interval.33
Aminoglycosides
Aminoglycosides are concentration-dependent bactericidal drugs; therefore, the higher the drug concentration, the greater the bactericidal effect. An optimal bactericidal effect occurs if a high enough dose is administered to produce a peak of 8 to 10 times the MIC. This can be accomplished by administering a single IV dose once daily, because a significant postantibiotic effect has been demonstrated. This regimen is at least as effective, and perhaps less nephrotoxic, than lower doses administered more frequently.21,22 Current dosing regimens in horses employ this strategy. The single daily dose is related to the drug’s volume of distribution (Vd) using the equation Dose = Cmax × Vd. A once-daily dose for gentamicin in adult horses using these guidelines is 4 to 6.8 mg/kg.23 The efficacy of these regimens has not been tested for conditions encountered in veterinary medicine, but the relationships are supported by studies in experimental animals. These regimens assume some competency of the immune system. If the animal is severely immunocompromised, the clinician may consider a more frequent interval for administration and synergistic combinations with β-lactam antibiotics.
Fluoroquinolones
Fluoroquinolone antibiotics are rapidly bactericidal and exhibit a significant postantibiotic effect. As reviewed in several papers,19,24–26 either the Cmax/MIC ratio or the AUC24/MIC ratio may predict clinical cure in studies of laboratory animals and in a limited number of human clinical studies. There are no published studies involving horses (or dogs and cats) to indicate which of these parameters will better predict clinical cure or what the respective target ratios might be. However, studies in experimental animals have demonstrated that a Cmax/MIC ratio of 8 to 10 or an AUC24/MIC ratio greater than 100 to 125 has been associated with a cure. The cited AUC/MIC ratio above 125 refers to administration to critically ill, neutropenic human patients. In other patients the ratio to achieve a cure may not be that high. Wright et al.25 presented evidence that AUC24/MIC ratios as low as 30 to 55 are associated with a clinical cure. This difference may reflect the severity of illness in the subjects of these investigations, but it also may be organism specific. With clinical doses used for many infections in veterinary medicine, the AUC/MIC ratios are often lower than 125, and clinical cures are still observed. An examination of the current use of the fluoroquinolones in veterinary medicine suggests that, in immunocompetent animals, AUC/MIC ratios of 50 to 60 are likely to be effective.26
Current guidelines recommend doses of fluoroquinolones to achieve Cmax/MIC ratios above these threshold levels, which have been associated with a lower incidence of the development of resistance.27 Sensitive bacteria from horses might be expected to have an MIC for enrofloxacin of 0.125 mg/mL or less based on available information.28 Pharmacokinetic studies available from horses showed that to achieve desirable PK-PD indices administering enrofloxacin, IV doses of 5 mg/kg once daily or 7.5 to 10 mg/kg orally may be adequate.29–32 We have monitored plasma concentrations in many clinical equine patients after oral and injectable administration of enrofloxacin (unpublished observations by the authors) and confirmed that these doses are adequate to achieve targeted plasma concentrations.
Beta-Lactam Antibiotics
Beta-lactam antibiotics such as penicillins, potentiated aminopenicillins, and cephalosporins are slowly bactericidal. Their concentration should be kept above the MIC throughout most of the dosing interval (long T>MIC) for the optimal bactericidal effect.33 In general, the goal is to maintain plasma concentrations above the MIC for at least 50% of the dosing interval. For the treatment of some gram-negative bacteria, some regimens for penicillins and cephalosporins require administration three to four times per day to meet this target. Some of the third-generation cephalosporins have longer half-lives, and less frequent dosing intervals have been used for these drugs (e.g., cefotaxime, ceftiofur). Because the MICs are lower for gram-positive bacteria, and antibacterial effects occur at concentrations below the MIC (postantibiotic effect, PAE), longer dose intervals may be possible for infections caused by gram-positive compared with gram-negative bacteria.
Bacteriostatic Drugs
Bacteriostatic drugs are most effective when concentrations of the drug at the site of the infection are maintained above the MIC for the entire dosing interval. In this way, they act in a time-dependent manner. However, the most predictive PK-PD index for antibacterial success is the AUC/MIC ratio. Many of the bacteriostatic drugs must be administered frequently or demonstrate a long half-life to achieve this goal. A property of some of these drugs is that they persist in tissues for a prolonged time, allowing infrequent dosing intervals. The macrolide derivative azithromycin (Zithromax, Pfizer) has shown tissue half-lives as long as 70 to 90 hours in cats and dogs, permitting infrequent dosing. Accumulation and persistence of azithromycin in polymorphonuclear leukocytes (PMNs) and macrophages of foals has also been demonstrated.34 Concentrations of clarithromycin can be maintained with twice-daily dosing in horses.35 Tissue concentrations of TMS persist long enough to allow once-daily dosing for many infections, although a study in equine joint infections showed that twice daily was more effective.36
TISSUE PENETRATION OF DRUGS
For most tissues, antibiotic drug concentrations in the serum or plasma can predict the drug concentration in the extracellular space (interstitial fluid). This is because no physical barrier impedes drug diffusion from the vascular compartment to extracellular tissue fluid.37 Pores (fenestrations) or microchannels in the endothelium of capillaries are large enough to allow drug molecules to penetrate. One important limitation involves drugs that are highly protein bound in the blood;38 examples of drugs for which this may be important in equine medicine include doxycycline and itraconazole.
For most antimicrobial drugs, the plasma drug concentrations produce tissue fluid concentrations in lung, pleural space, skin, abdominal fluid, joint fluid, soft tissues, and bone that are similar to steady-state plasma drug concentrations. For example, gentamicin reached concentrations in lymph fluid of horses that closely paralleled plasma concentrations.39 A study from our laboratory showed that ticarcillin diffuses into mare’s tissues adequately when one accounts for the percentage of tissue occupied by extracellular water.40 Although gentamicin and ticarcillin are not very lipophilic, they are able to diffuse from the plasma to extracellular fluid of these tissues easily. Rapid equilibration between the extracellular fluid and plasma is possible because of high surface area/volume ratio (high SA/V). That is, the surface area of the capillaries is high relative to the volume into which the drug diffuses.
Tissues once assumed to present a barrier to drug diffusion actually attain adequate drug penetration. For example, it is a common misconception that drug penetration into synovial fluid of joints is impaired in horses. Penetration of ampicillin and gentamicin is adequate from the vascular compartment to synovial fluid in horses,41 but equilibrium is often delayed because of the synovial volume (low SA/V). Ensink et al.42 and Anderson et al.39 also showed the ability of plasma concentrations to predict synovial fluid concentrations. In their studies of gentamicin and ampicillin, delayed equilibrium was caused by the lower SA/V ratio in joints, but after equilibrium was achieved, synovial fluid concentrations either paralleled plasma drug concentrations or declined more slowly. That these drugs penetrated joints is an important observation, because ampicillin and gentamicin are incapable of diffusing readily through lipid membranes and rely on diffusion through capillary pores. Beta-lactam antibiotics and aminoglycosides penetrated inflamed joints more rapidly and achieved higher concentrations than in healthy joints,43 most likely from increased blood flow to the joint. However, penetration during chronic inflammation could be impeded by presence of pus and fibrosis.
When the SA/V ratio is small and the drug’s elimination from the plasma is rapid, some drugs may not have sufficient time to diffuse adequately into infected sites. For example cefapirin diffuses poorly into infected tissue chambers in horses because (1) these tissue chambers have a different geometry (lower SA/V) than the tissue compartment predicted by a pharmacokinetic model, and (2) cefapirin is rapidly eliminated from plasma with a half-life of 19 minutes, which does not allow enough time for equilibrium between the tissue cage and serum.44
Impaired Diffusion into Tissues
Tissues that lack pores or channels may inhibit penetration of some drugs. In some tissues a lipid membrane (e.g., tight junctions in capillaries) presents a barrier to drug diffusion. In these cases a drug must be sufficiently lipid soluble or must be actively carried across the membrane to reach effective concentrations in tissues. These tissues include the central nervous system (CNS), eye, and prostate. Many clinicians believe that drug penetration across these barriers is not important when treating inflammatory diseases, because these barriers will be breached and drugs will be able to diffuse freely into the affected area. However, this is not always the case. In a study analyzing amikacin concentrations in the CSF of the horse, drug was not detected in any of the horses with a normal blood-brain barrier (BBB).46 In one horse that developed septic meningitis during the study, drug was not detectable until 4 hours after the second injection and reached a peak of only 0.97 μg/mL, which did not occur until 8 hours after the fifth injection.
Box 71-1 summarizes drugs known to penetrate into the cerebrospinal fluid (CSF) and aqueous humor of horses. Also, a barrier exists between plasma and bronchial epithelium (blood-bronchus barrier).45 This restricts penetration of some drugs in the bronchial secretions and epithelial fluid of the airways. However, disposition of drug into lung tissue not separated by the blood-bronchus barrier is not impaired (e.g., when treating pneumonia).
Box 71-1 Summary of Drugs Studied in Cerebrospinal Fluid (CSF) and Aqueous Humor in the Horse*
Intracellular Drug Penetration
Most bacterial infections are located extracellularly, and a cure can be achieved with adequate drug concentrations in the extracellular (interstitial) space rather than intracellular space. Intracellular infections, however, present a different problem. For drugs to reach intracellular sites, they must either diffuse passively or utilize a transport process. One of the most important equine intracellular organisms is Rhodococcus equi. Drugs traditionally used for treatment of R. equi pneumonia in foals include erythromycin and rifampin because these drugs are known for their ability to achieve high concentrations intracellularly.47 Treatment of rickettsial infections in horses also requires intracellular penetration. Infections caused by Neorickettsia risticii (formerly known as Ehrlichia risticii) and Anaplasma phagocytophilium (formerly known as E. equi) have been treated most often with tetracyclines because they are known to attain sufficient intracellular concentrations. Other intracellular organisms include Chlamydia and Mycobacterium. Staphylococci may become resistant to treatment in some cases because of intracellular survival.
Examples of drugs that accumulate in leukocytes, fibroblasts, macrophages, and other cells are fluoroquinolones, tetracyclines, macrolides (erythromycin, clarithromycin), and the azalides (azithromycin).48 Beta-lactam antibiotics and aminoglycosides do not reach effective concentrations within cells. Doxycycline, despite high protein binding in horses, achieves leukocyte concentrations 17 times greater than maximum plasma concentrations.49 This may explain why doxycycline is efficacious in the treatment of many bacterial infections, despite the low plasma concentrations. The erythromycin derivative azithromycin (Zithromax) achieves particularly high concentrations of active drug intracellularly. In equine studies the oral absorption of azithromycin in foals was 33%, and the concentrations achieved in phagocytes were 200 times the corresponding plasma concentrations.34 Either clarithromycin or azithromycin may have potential for treating intracellular infections such as R. equi in foals. In a study comparing treatment with azithromycin and rifampin to clarithromycin and rifampin, the group that received clarithromycin at recommended doses35 had a higher clinical success rate.50 This difference might have resulted from higher activity for clarithromycin rather than a pharmacokinetic difference.51
LOCAL FACTORS THAT AFFECT ANTIBIOTIC EFFECTIVENESS
Local tissue factors may decrease antimicrobial effectiveness. For example, pus and necrotic debris may bind and inactivate vancomycin or aminoglycoside antibiotics, causing them to be ineffective. Cellular material also can decrease the activity of topical agents such as polymyxin B. Foreign material in a wound, such as material surgically implanted, can protect bacteria from antibiotics and phagocytosis by forming a biofilm (glycocalyx) at the site of infection.52,53 Cations can adversely affect the activity of antimicrobials at the site of infection. Two important drug groups diminished in activity by cations (e.g., Mg+2, Al+3, Ca+2) at the site of infection are fluoroquinolones and aminoglycosides. (Cations such as magnesium, iron, and aluminum also can inhibit oral absorption of fluoroquinolones.)
The acidic environment of infected tissue may decrease the effectiveness of erythromycin, other macrolides, fluoroquinolones, and aminoglycosides. Penicillin and tetracycline activity is not affected as much by tissue pH, but hemoglobin at the site of infection will decrease the activity of these drugs. An anaerobic environment decreases the effectiveness of aminoglycosides because oxygen is necessary for drug penetration into bacteria. TMS combinations are sometimes not effective in vivo despite in vitro results that suggest susceptibility.54 The impediment to successful TMS activity is the material in infected tissue that inhibits drug activity. In a study using experimental ponies, trimethoprim-sulfadiazine was administered at 30 mg/kg (combined) every 12 hours (q12h).55 This dose produced drug concentrations above the MIC of the infecting pathogen, Streptococcus equi subsp. equi, in an infected tissue chamber model. However, trimethoprim-sulfadiazine was ineffective for eliminating the infection compared with injections of penicillin G. In a follow-up study the investigators showed that trimethoprim-sulfadiazine (30 mg/kg q12h) administered to ponies with experimentally infected tissue cages was also ineffective for preventing infection even if administered prophylactically.56 The investigators of these studies propose that treatment failure with trimethoprim-sulfadiazine in ponies is cause by composition of the tissue fluid in the infected chamber. Tissues may contain thymidine and para-aminobenzoic acid (PABA), which are inhibitors of the action of trimethoprim and sulfonamides, respectively. On the other hand, penicillin, ampicillin, and ampicillin derivatives have been successful in these same Streptococcus tissue cage models and may be preferred for treatment of clinical cases.
ABSORPTION OF ANTIMICROBIALS IN HORSES
Injectable Drugs
Many injectable solutions can be administered intravenously (IV), which delivers high concentrations to tissues rapidly. Intramuscular (IM) administration also is suitable for some drugs, although pain and muscle injury from injection can be important drawbacks. The absorption rate from an IM injection usually is sufficient to achieve high concentrations rapidly, and absorption usually is complete. For some drugs, slow release of the drug from the IM injection may effectively prolong the dosing interval. Uboh et al.57 showed that after IM administration of penicillin G potassium, the plasma penicillin concentrations 24 hours after administration were similar to concentrations after IM administration of procaine penicillin. Prior to that report, it was assumed that the sole reason for prolonged absorption of penicillin from procaine penicillin G was the presence of procaine and slow release from the injection site.58 Apparently, however, IM injection of soluble salts (e.g., penicillin potassium) also will produce prolonged plasma concentrations.57 The long half-life from IM injection of these solutions is probably caused by disruption of blood flow at the injection site after IM administration and slower uptake into the circulation. Because the rate of absorption determines the terminal half-life in these cases, half-life is prolonged. Pharmacokineticists refer to this as the “flip-flop effect.” Systemic availability (% F) may be falsely overestimated in studies in which the flip-flop effect is observed.
As in cattle,59 the site of IM injection also affects drug absorption. In studies comparing different IM sites, injections in the neck muscle of horses showed faster and more complete drug absorption compared with injections in the gluteal or hamstring muscles (semitendinosus).58
Oral Absorption
As reviewed by Baggot,60 oral absorption is low for many drugs in horses. Drugs such as aminopenicillins (ampicillin, amoxicillin), cephalosporins, and macrolide antibiotics are not absorbed as rapidly or to as great an extent compared with administration of these drugs in small animals or humans. This limits the use of the oral route for many drugs in horses. For example, oral amoxicillin is absorbed well enough in humans, dogs, and cats to be a useful and practical route of administration. However, systemic availability of oral amoxicillin in adult horses is only 2% to 10%.42,61 Ampicillin also is not a good option for oral administration in horses because of poor availability.62 Even though oral absorption is poor for these drugs in adult horses, there may be an advantage for oral administration in foals because they appear to exhibit higher oral absorption. For example, compared with the poor absorption cited for adult horses, oral absorption of amoxicillin in foals is better, 36% to 42%.63
For cephalosporins, the same pattern is observed. Cefadroxil is absorbed better in the foal than in adult horses.64,65 Oral absorption of cephalexin is low in horses (5%), but at 30 mg/kg orally q8h, concentrations can be maintained above the MIC of susceptible bacteria.66
Modification of some drugs has improved oral absorption in horses. The ester prodrugs of ampicillin, such as bacampicillin and pivampicillin, produce moderate systemic availability in horses after oral administration (35%-45%).67 However, these prodrugs are no longer available as commercial products in the United States. Esters and salts of erythromycin also have improved oral absorption in horses. Erythromycin base administered to horses is rapidly degraded into inactive metabolites in the equine stomach and intestine, and systemic availability of erythromycin is poor.68 However, if erythromycin is administered as an ester prodrug such as erythromycin estolate, it is absorbed as the intact ester and converted to the active drug after absorption. Oral absorption is also improved if erythromycin is administered as a phosphate salt, whereby it resists degradation in the stomach and intestine and is absorbed as active erythromycin.69
The use of an ester prodrug was recently demonstrated for cefpodoxime proxetil (Simplicef, Pfizer) in horses.70 The clinical use of cefpodoxime is discussed in more detail later (see Cephalosporins).
The effect of food on the oral absorption of drugs in veterinary medicine is often overlooked. Most pharmacokinetic studies administer drugs to horses that have been fasted before and after drug administration. However, this may not be possible, or practical, in field conditions. Significantly decreased drug absorption has been demonstrated for several oral antibiotics in the horse when they are administered with feed. When healthy foals were given microencapsulated erythromycin base, they had decreased plasma concentrations and systemic bioavailability when they were allowed to eat hay up until the time of drug administration compared with those that were fasted.68 Trimethoprim-sulfachlorpyridazine combinations were shown to bind to feed constituents and cecal contents in vitro.71 In addition, systemic bioavailability was significantly decreased when the drug was administered as a topical dressing compared with nasogastric intubation.72 Oral bioavailability of rifampin decreased from 68% when it was administered 1 hour before feeding to 26% when administered 1 hour after feeding.60 Recent studies have also shown that the extent of oral absorption of doxycycline increased by approximately 50% when it was administered by nasogastric tube to a fasted horse versus a fed horse.49 Whenever possible, feeding schedules should be taken into account when dosing with oral antibiotics.
Pharmaceutical compounds can be grouped into one of the following categories:
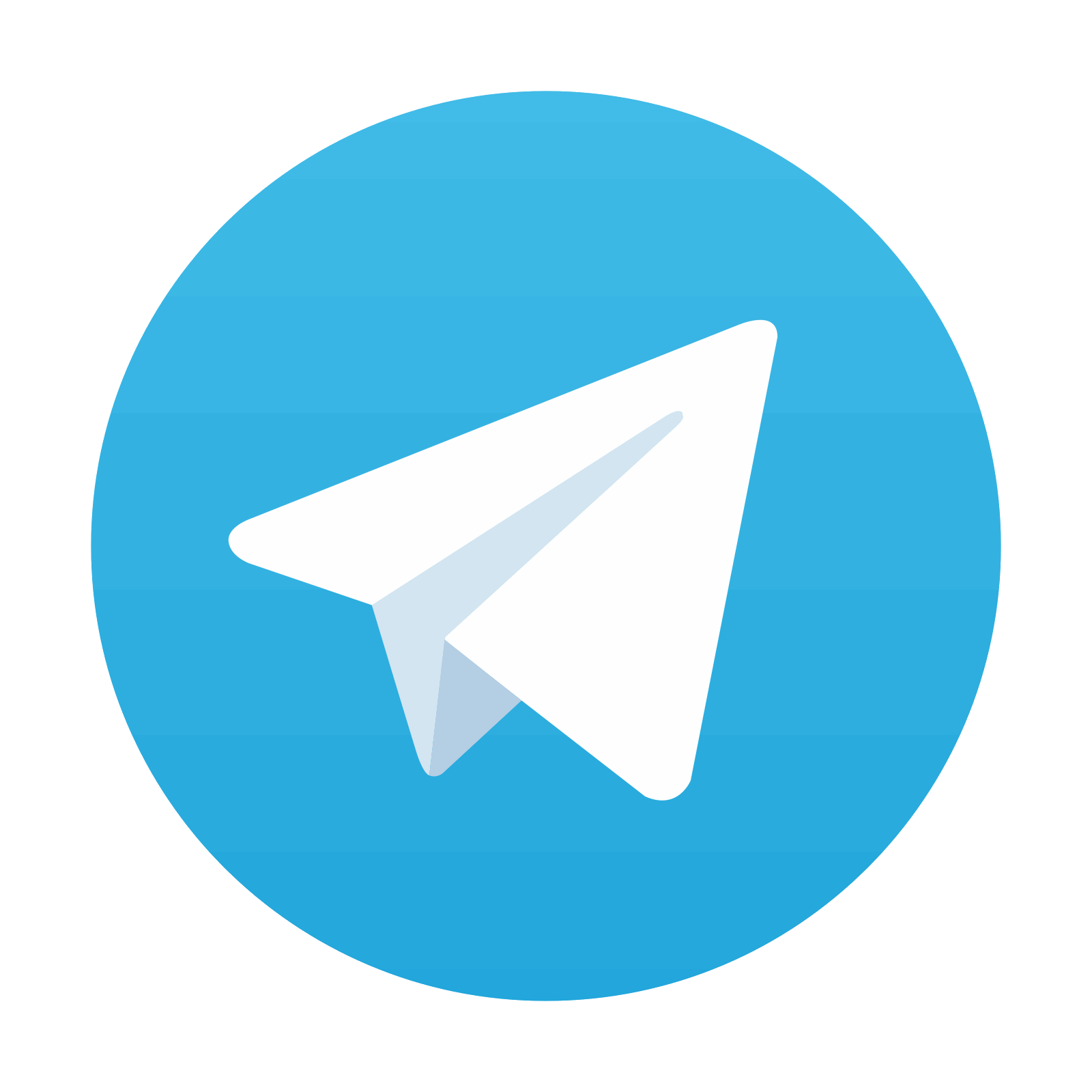
Stay updated, free articles. Join our Telegram channel

Full access? Get Clinical Tree
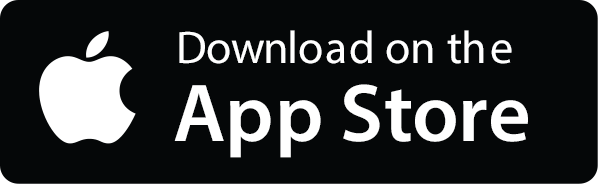
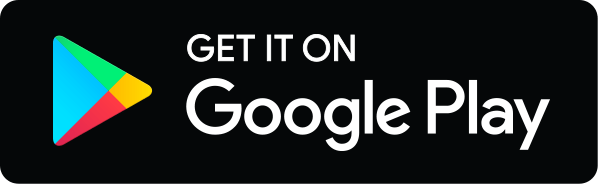