© Springer International Publishing Switzerland 2016
Chi-Chao Chan (ed.)Animal Models of Ophthalmic DiseasesEssentials in Ophthalmology10.1007/978-3-319-19434-9_77. Animal Models of Retinitis Pigmentosa (RP)
(1)
The Jackson Laboratory, 600 Main Street, 04609 Bar Harbor, ME, USA
7.1 Retinal Degeneration in Retinitis Pigmentosa Patients
Retinitis pigmentosa (RP) is the most common form of inherited retinal degeneration (RD) with a frequency of 1 in 3000–7000 individuals (Ferrari et al. 2011) , with more than one million people affected worldwide. The term “retinitis” was first used in the 1850s because doctors thought the retina appeared inflamed. We now know that most RP is not caused by infection, but is generally an inherited condition. The word “pigmentosa” refers to the pigment deposits seen during the examination of the fundus. Typical symptoms include night blindness followed by decreasing visual fields, leading to tunnel vision and eventually legal blindness or, in many cases, complete blindness. On the cellular level, this correlates with a predominantly dysfunctional rod photoreceptor system. In later stages, the disease may further affect cone photoreceptors , eventually causing complete blindness. Findings on retinal examination include “bone spicule” pigmentary deposits, retinal vessel attenuation, abnormal, diminished or absence of a- and b-waves in electroretinogram (ERG) , and reduced visual field (Daiger et al. 2013) . RP can be inherited in an autosomal dominant, autosomal recessive, or X-linked manner. While usually limited to the eye, RP may also occur as part of a syndrome as in the Usher syndrome and Bardet–Biedl syndrome. Over 50 genes have been associated with RP and nearly 3100 mutations have been reported in these genes (Daiger et al. 2013). Among the autosomal recessive RP (arRP) genes identified thus far, mutations within PDE6A (coding for phosphodiesterase 6A) and PDE6B (coding for phosphodiesterase 6B) , the genes associated with the PDE6 complex that are essential for photoreceptor function and maintenance are, in fact, the second most common identifiable cause of arRP. About 36,000 cases of simplex and familial RP worldwide are due to defects in the PDE6 complex, estimated to account for approximately 8 % of all diagnosed arRP (Ferrari et al. 2011; Daiger et al. 2013) . Mutations in the gene encoding the beta subunit of rod phosphodiesterase (PDE6B) are the most common identified cause of arRP, accounting for approximately 4 % of cases in North America (McLaughlin et al. 1995) .
7.2 RD in Animal Models
Models of RD have been discovered or generated in mice, rats, rabbits, pigs, zebrafish, and nonhuman primates . Mouse models offer the advantages of low cost, disease progression on a relatively quick time scale, and the ability to perform genetic manipulations. The ability to target and alter a specific gene(s) is an important and necessary tool to produce mouse models with mutations in genes of choice. Inducing mutations in genes of choice (known as knockout or transgenic) is termed as “reverse genetics,” while “forward genetics” approaches initiate as spontaneous/induced mutations that are discovered as a result of the overt phenotypes, and the underlying mutation is subsequently identified. Through the “forward” and “reverse” genetic approaches, mouse models in > 100 genes that underlie human retinal diseases have been studied (Chang et al. 2007a; Samardzija et al. 2010; Won et al. 2011) .
Mouse models of RD have been known for many years. The first RD model, discovered by Dr Clyde E. Keeler more than 90 years ago (Keeler 1924, 1966) , was identified as a mutation in Pde6b (formerly rd1, rd, identical with Keeler rodless retina, r) (Pittler et al. 1993) . Frequently, mutated genes are identified first in the mouse. For example, the rod phosphodiesterase beta-subunit (PDE6B) and peripherin/RDS were first found to be the cause of RD in mice before their connection to human diseases was demonstrated. Discovery of the mouse retinal degeneration 1 (rd1) mutation in the gene for the beta-subunit of cGMP-phosphodiesterase (Pde6b) (Bowes et al. 1990) led to the identification of mutations in the human homolog (PDE6B) in similar human disorders (McLaughlin et al. 1993) , and discovery of the mutation Rds (retinal degeneration slow) in the peripherin 2 (Prph2) (Travis et al. 1989) paved the way for the identification of defects in the RDS/peripherin gene in patients with autosomal dominant RP and various cone, cone–rod, and macular dystrophies (Keen and Inglehearn 1996) . Identification of the tubby (tub) mouse gene family (Noben-Trauth et al. 1996) led to the finding of tubby-like protein 1 (TULP1) alterations in individuals affected with arRP (Hagstrom et al. 1998; Banerjee et al. 1998) . Similarly, the shaker 1 (sh1) mutation in the myosin 7A (Myo7a) (Gibson et al. 1995) mouse gene led to identification of mutations in MYO7A in patients with Usher’s syndrome type 1b (Weil et al. 1995) . Additionally, it was the studies on mouse that led to the identification of apoptosis as an important mechanism of photoreceptor cell death in RD (Chang et al. 1993; Portera-Cailliau et al. 1994) .
7.3 Using the rd10 Mouse Model for studies of RP
The retinal degeneration 10 (rd10) model was discovered more than 10 years ago (Chang et al. 2002) . Mice homozygous for the rd10 mutation show grainy retinas with sclerotic retinal vessels by indirect ophthalmoscopy at 4 weeks of age. Histology at 3 weeks of age shows photoreceptor degeneration that progress as mice age. Electroretinograms of rd10/rd10 mice are never normal. The maximal response occurs at 3 weeks of age and is not detectable at 2 months of age. Genetic analysis showed that this disorder was caused by an autosomal recessive mutation that mapped to mouse Chr 5. Sequence analysis showed a missense mutation in exon 13 of the beta subunit of the rod phosphodiesterase gene. Therefore, the gene symbol for the rd10 mutation is Pde6b rd10 (Chang et al. 2002). In contrast to rd1, the onset of RD in the rd10 mouse is later and can be delayed by rearing in darkness. Also, while the PDE6B protein and activity in rd1 mutant mice are undetectable, they are detected early, at P10, in rd10 mouse retinas with western blotting and immunostaining. However, the protein level is significantly reduced compared with that of age-matched wild-type controls (Chang et al. 2007b). The residual activity of PDE6B in rd10 retinas may prevent a toxic increase in cGMP early in the mouse’s life, thus delaying the onset of photoreceptor cell death, and extending the window for therapeutic intervention. Many missense, pathogenic human mutations in PDE6B leading to arRP are located within the catalytic domain (McLaughlin et al. 1995) , potentially resulting in partial loss of function and reduced PDE6B enzymatic activity, as seen in the rd10 allele. Thus, the rd10 represents a better mouse model than rd1 for developing strategies for treating human patients with recessive RP (Han et al. 2013) .
7.3.1 Methodology Used to Characterize the rd10 Mouse Model
Many of the clinical and functional methods used in routine eye examinations in human patients have been adapted to examine the small eyes of the mouse. Additionally, invasive methods that cannot be used in human patients are available and being developed to further our understanding of progression and pathology of retinal diseases. In the following paragraphs, we describe the methods that have been used to characterize the Pde6b rd10 model (herein referred to as rd10), which is proving to be an invaluable model for understanding RP and for testing different therapeutic approaches.
Indirect ophthalmoscopy, a noninvasive clinical method, which allows for the examination of the fundus, is a primary screen used in mice. However, due to the small size of the mouse eye, a 60–90 diopter lens is used to magnify the fundus. Using this method, retinal vessel attenuation is observed at 3 weeks of age and “bone spicule” pigmentary deposits at 2 months of age in rd10 mutants (Chang et al. 2007b) . Spectral domain optical coherence tomography (sd-OCT) scans are another noninvasive clinical technique that can be a powerful tool to monitor the course of RD with respect to morphometric changes. In rd10 mice, retinal thickness declines up to 9 weeks of age; the loss of thickness is predominantly due to the thinning of the outer nuclear layer (ONL). Subsequently, the ONL is no longer visible by OCT, while the thickness of inner retinal layers remains nearly constant (Rösch et al. 2014) . A previously unreported phenotype was observed using OCT, a separation of the retina from the retinal pigmented epithelium (RPE) was reported in some rd10 mutants at P27, but by P64, almost all rd10 mice demonstrated some degree of retinal separation. The retinal separation was never seen in rd1 mice, which bears a mutation in the same Pde6b gene (Pennesi et al. 2012) . In contrast, retinal separation was only observed in two of six mice examined by OCT, one 9-week-old and another 24-week-old rd10 mouse by Rösch and coworkers (Rösch et al. 2014) , suggesting potential segregating genetic background modifiers or environmental interactions with the mutant allele.
The sd-OCT has also been used to evaluate a therapeutic endpoint in rd10 mice. The ability to compare relative retinal thicknesses noninvasively in treated versus untreated eyes of rd10 mice provides a valuable tool in which to score the effects of therapeutic intervention. The ability to monitor therapeutic outcome (retinal thickness) longitudinally without terminating the experimental animals greatly enhances the ability to carry out long-term rescue studies (in the rd10 model as well as in others) and dramatically decreases the number of animals required for such studies (Pang et al. 2011) . Finally, blood flow (BF) and anatomical magnetic resonance imaging (MRI) can also be used as a clinical method of assessing retinal alterations. In rd10 mice, retinal BF decreased progressively over time while choroidal BF was unchanged. Similar to observations using OCT, by anatomical MRI, the rd10 retina became progressively thinner at later time points compared with that of age-matched controls (Muir et al. 2013) .
While clinical methods allow for noninvasive approaches to examine potential morphometric alterations as a consequence of disease, it is also important to be able to detect functional abnormalities that may or may not accompany morphological defects. Electroretinography is a functional test that assesses the response of photoreceptors and secondary neurons to light stimulation. The rd10 mouse ERG amplitude is smaller at all flash intensities at 18 days of age compared to wild-type responses, and there is a progressive loss of cone function initiating at P30. However, the rate of cone function loss is not as fast as rod function loss in rd10 mutants. At P18, the rd10 dark-adapted response was 30% of the C57BL/6 response while the light-adapted response was 50% of the wild-type response. Similarly, at P30, the rd10 dark-adapted b-wave was only 10% of controls while the light-adapted response was 30% (Chang et al. 2007b; Jae et al. 2013; Rösch et al. 2014) . The optomotor test is a noninvasive technique, widely used for the evaluation of visual function in rodents. It is based on the optokinetic reflex that causes the animals to track a drifting stripe pattern with eye and head movements. Optometry demonstrated that the rd10 mutants show a progressive decline in visual performance (Benkner et al. 2013; Thomas et al. 2010) . The optomotor test, which is used to test the efficacy of AAV8 (Y733F)-smCBA-PDE β for rescue of both photopic and scotopic vision in rd10 mice, demonstrated that visual performance was significantly better in treated rd10 eyes compared with untreated rd10 eyes (Pang et al. 2011) .
Animal models allow for the direct examination of changes by invasive methods that cannot be reasonably done in human patients. Standard histology of tissue specimens has been commonly used to assess changes in particular cell types in the retina. For example, histological examination revealed progressive retinal ONL degeneration in rd10 mice that initiates in the central retina at P16 and spreads to the peripheral retina by P20. By P25, only two to three layers of nuclei are left, and by P45, only a single layer of photoreceptors is left. Complete absence of photoreceptor nuclei is observed by P60. However, the cell number within the inner nuclear and ganglion cell layers does not appear to be affected (Chang et al. 2007b; Gargini et al. 2007) . While histology has been considered the gold standard for assessing and quantifying the rate of RD in animal models , as well as for judging the efficacy of potential treatments, many disadvantages are associated with histologic processing. Because of the inherent need to sacrifice animals to obtain histologic data, an increase in the number of sequential time points in a long-term study greatly increases the total number of animals needed to conduct histologic analyses. Additionally, gathering histologic samples makes it impossible to perform such studies on the same animal over time. OCT and MRI may potentially be used to replace or at least significantly reduce the number of specimens examined by histology, as histologic results have been correlated and confirmed by OCT (Rösch et al. 2014; Pennesi et al. 2012; Pang et al. 2011) and by MRI (Muir et al. 2013) in rd10 mutants. There are situations, however, in which direct examination of the tissue may provide insights into the disease phenotypes observed. A number of investigators have used RPE whole mounts to examine the effects of the Pde6b rd10 mutation on RPE cells, which functionally and structurally support the overlying photoreceptor cells. In normal retinas, the RPE morphology resembles a regular hexagonal array of cells of uniform size. In the rd10 retina, a clear disruption of RPE cell size and shape is observed (Chrenek et al. 2012; Jiang et al. 2013, 2014) . This RPE abnormality might be the cause for the retinal separation (retinal detachment) (Jae et al. 2013; Rösch et al. 2014) observed in the rd10 mouse. Further study is needed to determine the cause of the retinal detachment in rd10 mutants that is not observed in rd1 mutants.
Immunohistochemistry (IHC) allows for the localization of antigens or proteins in tissue sections by the use of labeled antibodies as specific reagents through antigen–antibody interactions that are visualized by a marker such as fluorescent dye, enzyme, or colloidal gold. It has been widely used in rd10 mice. By using a PDE6b polyclonal antibody, staining was found in the inner and outer segments of photoreceptors in cryosections of P22 wild-type and rd10 retina, but not in age-matched rd1 retina cryosections, indicating the delayed photoreceptor degeneration in rd10 mutants compared with rd1 mutants. But the level of PDE6B expression in rd10 mutants was significantly reduced compared with that of wild-type control (Chang et al. 2007b) . Other groups also have used IHC to evaluate the progression of neuronal remodeling of second- and third-order retinal cells and their synaptic terminals in retinas from rd10 mice at varying stages of degeneration ranging from P30 to postnatal month 9.5. Significant remodeling is observed in rd10 mutants relative to wild-type control mice, especially at later stages of disease progression, as assessed by examination of cell- and synapse-specific markers (Phillips et al. 2010, 2011; Mazzoni et al. 2008; Barhoum et al. 2008) .
Because of the milder disease phenotype in rd10 mice, in which photoreceptor loss progresses more slowly than in rd1 mice, examination of the effects of photoreceptor loss on the remaining retinal structures can be done. For example, Menzler et al. (2014) examined the retinal ganglion cell (RGC) structure and function in rd10 mice. RGCs are the only retinal neurons that project to the brain and their normal structure and function are very important for vision. Using a single-cell approach, the investigators showed that all types of RGCs in rd10 mice retain their dendritic architecture and overall viability, even after complete photoreceptor degeneration and regressive remodeling of the retina’s second-order neurons up to 9 months of age (Menzler et al. 2014) . Rhythmic RGC activity in healthy retinas was detected upon partial photoreceptor bleaching using an extracellular high-density multitransistor array. The mean fundamental spiking frequency in bleached retinas was 4.3 Hz; close to the RGC rhythm detected in blind rd10 mouse retinas (6.5 Hz) (Biswas et al. 2014) . Other studies showed that the rhythmic RGC activity in rd10 mouse retinas was around 3–7 Hz, and in the rd1 mouse retinas was around 10–16 Hz (Goo et al. 2011a, b) . These results suggest that in rd10 retinas, the retinal circuitry may be less affected by photoreceptor loss. Further evidence is presented in a study on light-evoked RGC activity (Stasheff et al. 2011) , where the light response properties of wild-type mice and rd10 mice RGCs at P28 appear very similar, which is a strong indicator that basic circuitry evolved normally in rd10. The rd1 retina, on the other hand, seems to possess a more defective circuitry.
The rd10 mouse model has also been used to validate new in vitro methods, which in turn have provided additional characterization of the model. An example is the use of a laser micrometer to make noncontact measurements of linear external dimensions of the mouse eye. The resolution of the laser micrometer is less than 0.77 μm, and it provides accurate and precise noncontact measurements of eye dimensions on three axes: axial length (anterior–posterior (A–P) axis) and equatorial diameter (superior–inferior (S–I) and nasal–temporal (N–T) axes). External dimensions of the eye strongly correlated with eye weight. The rd10 mice were found to have a slightly smaller eye size and weight when compared with that of WT C57BL/6J mice, which might cause the hyperopia observed in rd10 mutant mice (Wisard et al. 2010) . The rd10 mice had significantly greater hyperopia relative to the WT controls throughout normal development; however, axial length became significantly shorter in rd10 mutants relative to WT mice starting at 7 weeks of age. Interestingly, while RD models with low basal levels of DOPAC (3,4-dihydroxyphenylacetic acid) in general showed an increased susceptibility to form deprivation myopia; refractive development under normal visual conditions was disrupted toward greater hyperopia from 4 to 12 weeks of age in the rd10 mice, despite significantly lower DOPAC levels. These results indicate that photoreceptor degeneration may alter dopamine metabolism, leading to increased susceptibility to myopia with an environmental visual challenge (Park et al. 2013) .
7.3.2 Studies Focused on the Underlying Cellular and Molecular Changes in the rd10 Mouse Model to Provide Insights About RP
A number of approaches have been taken to elucidate the cellular responses that result from the rd10 mutation, which include both global- and pathway-specific approaches. A global transcriptome study carried out by Uren and coworkers (Uren et al. 2014) found a total of 1079 genes with significant changes in mRNA levels. Of those, 385 showed a relative increase in expression, while 694 showed a relative decrease in expression in rd10 retinas compared with that of controls. Genes with relative decreased expression were mainly rod-specific genes, and their extreme decrease is expected, driven by the loss of rod photoreceptors. Genes with relative increased expression were predominantly Müller-specific genes; the proportion of which was significantly higher than expected under the null hypothesis that increased transcript expression would not favor any particular cell type. This suggests either increased numbers or modified activity of Müller glial cells. In addition to the prominent changes observed in photoreceptor and Müller cells, a relative decrease in expression of a few amacrine and bipolar cell-specific genes and increase in amacrine, ganglion, and bipolar-specific genes were also observed. This indicates transcriptional changes in cells other than rods and Müller glia (Uren et al. 2014) . This study implicates remodeling of the inner retina and possible Müller cell dedifferentiation in the rd10 mouse retina.
Pathway-specific transcriptome analysis of survival pathways showed that the rd10 retina appears to attempt to protect photoreceptors from death and provides an excellent model to study the molecular mechanisms of neuroprotection and cell death. The degenerating rd10 retina rapidly induces expression of transcriptional regulators Atf3 (transcription factor 3) and Cebpd (CCAAT/enhancer-binding protein delta). Induction of Atf3 was transient and only lasted for several days at the beginning of degeneration, whereas levels of Cebpd remained elevated throughout the period of photoreceptor loss. Several protective genes such as Lif (leukemia inhibitory factor), Edn2 (endothelin-2), and Fgf2 (fibroblast growth factor-2), which are implicated in a potent endogenous survival pathway, and Mt1 (metallothionein-1) and Mt2, were strongly upregulated in the rd10 retina. In addition, increased expression of Casp1 (caspase-1) and Il1b (interleukin-1β) suggested an inflammatory response (Samardzija et al. 2012) . Other studies also demonstrated that when retinal cells are stressed there is an initial struggle to survive, mediated through inhibition of PP2A (protein phosphatase 2A) and subsequent upregulation of survival pathways in the rd10 mouse (Finnegan et al. 2010).
While in general, rod photoreceptor cell death occurs through apoptosis, necrosis, a form of cell injury that results in autolysis of living tissue, can also cause premature cell death. Ultrastructural analysis of rd10 mouse retinas revealed that a substantial fraction of dying cones exhibited necrotic morphology. RIP1 and RIP3, two members of the receptor-interacting protein (RIP) kinase family of proteins, have been identified as critical mediators of programmed necrosis, and interestingly, Rip3 deficiency is able to mediate partial rescue of cells in rd10 mutants. Additionally, pharmacologic treatment with a RIP kinase inhibitor attenuated histological and functional deficits of cones in rd10 mice (Murakami et al. 2012b) .
Recently, there has been a great deal of interest in the role of microglia homeostasis in retinal diseases (Karlstetter et al. 2010) . Notwithstanding, activated microglia have been reported to be an important contributor to the overall apoptosis of photoreceptors in rd10 retinas. The suppression of microglia activation by minocycline or SC-560, a cyclooxygenase-1 (COX-1)-specific inhibitor, reduced microglia-mediated photoreceptor death, and significantly improved retinal structure and function and visual behavior in rd10 mice. Their neuroprotective effects are thought to occur through both anti-inflammatory and antiapoptotic mechanisms (Peng et al. 2014) . The activated Cx3cr1 (chemokine (C-X3-C motif) receptor 1, deficient of microglia, had an additional neurotoxic effect on photoreceptor survival in rd10 retinas, suggesting that the Cx3cl1 (chemokine (C-X3-C motif) ligand 1)/Cx3cr1 signaling pathway might protect against microglia neurotoxicity (Peng et al. 2014, Zieger et al. 2014). In comparison with wild-type controls, retinas of rd10 mice have significantly lower levels of total CX3CL1 (fractalkine) protein (from P10 onwards) and lower CX3CL1 mRNA levels (from P14), even before the onset of primary rod degeneration (Zieger et al. 2014) . The rd10 mouse retina exhibits high levels of photoreceptor cell death and reactive Müller gliosis early in the degenerative process. In explant cultures, both degenerative processes were abrogated by insulin-like growth factor 1 (IGF-1) treatment. Moreover, the beneficial effect of IGF-I was diminished by microglial depletion using clodronate-containing liposomes. Interestingly, in the absence of IGF-I, microglial depletion partially prevented the cell death without affecting Müller gliosis. These findings strongly suggest a role for microglia–Müller glia crosstalk in neuroprotection, and the beneficial neuroprotective effects may be achieved through strategies that modulate microglial cell responses (Arroba et al. 2011, 2014) . Neuroinflammation involving CC chemokine (or β-chemokine) proteins such as monocyte chemoattractant protein-1 (MCP-1) and its receptor CCR2 (chemokine (C-C motif) receptor 2) have been studied in the rd10 retina and results suggest that the MCP-1/CCR2 system plays a role in RD (Guo et al. 2012) . The number of microglia in the retinas of the Ccr2−/−; rd10/rd10 double mutant mice was significantly lower than that in the retinas of mutant mice bearing the rd10/rd10 mutation alone. The average number of cells in the ONL and the ERG amplitude were significantly higher in the Ccr2−/−; rd10/rd10 double-mutant mice than in the rd10/rd10 single-mutant mice (Guo et al. 2012). Finally, Yoshida and coworkers have shown that the sustained chronic inflammatory reaction contributes to the pathogenesis of RD in rd10 mice and antioxidant treatment with N-acetylcysteine (NAC) prevented the photoreceptor cell death along with suppression of inflammatory factors and microglial activation (Yoshida et al. 2013) . This suggests that an intervention for ocular inflammatory reaction using antioxidants may be a potential treatment for patients with RP.
The latter study implicates oxidative stress in the disease pathology observed in rd10 mutants. Oxidative stress reflects an imbalance between the systemic manifestation of reactive oxygen species and a biological system’s ability to readily detoxify the reactive intermediates or to repair the resulting damage. DNA oxidation was increased in an early phase of RD in the rd10 mouse. Transgenic overexpression of human MutT homolog-1(hMTH1) in the rd10 mouse attenuated accumulation of oxidative DNA damage, prevented the subsequent poly(ADP-ribose) polymerase (PARP) activation, and delayed photoreceptor cell death (Murakami et al. 2012) . The metallo-complex zinc-desferrioxamine (Zn/DFO) may ameliorate the oxidative stress by chelation of labile iron in combination with release of zinc. Zn/DFO-treated rd10 mice showed significantly higher electroretinographic responses at 3 and 4.5 weeks of age compared with saline-injected controls. Corresponding retinal (photoreceptor) structural rescue was observed by quantitative histological and immunohistochemical techniques (Obolensky et al. 2011) . Coexpression of superoxide dismutase 1 (SOD1) with glutathione peroxidase 4 (Gpx4), which like SOD1 is localized in the cytoplasm, but not with catalase targeted to the mitochondria, reduced oxidative stress in the retina and significantly slowed the loss of cone cell function in rd10 mice (Usui et al. 2011) . The benzopyran BP (3,4-dihydro-6-hydroxy-7-methoxy-2,2-dimethyl-1(2H)-benzopyran) is a free radical scavenger that is structurally similar to alpha-tocopherol and has provided neuroprotection in a number of disease models where oxidative stress is a causative factor. A novel derivative of BP with improved lipid solubility has been designated BP3. Systemic dosing with BP3 on alternate days between postnatal days 18 and 25 preserved rod photoreceptor numbers and cone photoreceptor morphology in rd10 mice (O’Driscoll et al. 2011) . Orally administered NAC reduced cone cell death and preserved cone function by reducing oxidative stress in the rd10 and rd1 mice. In rd10 mice, supplementation of drinking water with NAC promoted partial maintenance of cone structure and function for at least 6 months. Topical application of NAC to the cornea of rd10 mice also reduced superoxide radicals in the retina and promoted survival and functioning of cones (Lee et al. 2011) . Two constituents of bile, bilirubin and tauroursodeoxycholic acid (TUDCA), have antioxidant activity. In rd10 mice, intraperitoneal injections of 5 or 50 mg/kg of bilirubin or 500 mg/kg of TUDCA every 3 days starting at P6 caused significant preservation of cone cell number and cone function at P50. Rods were not protected at P50, but both bilirubin and TUDCA provided modest preservation of ONL thickness and rod function at P30 (Oveson et al. 2011) . Other studies showed that TUDCA treatment preserved rod and cone function and greatly preserved overall photoreceptor numbers (Drack et al. 2012; Phillips et al. 2008; Boatright et al. 2006) . Para-aminobenzoic acid (PABA) is a cyclic amino acid, which may act to decrease lipid peroxidation and oxidative stress. PABA (50 mg/kg) was administered intraperitoneally six times per week in rd10 mice from P3. PABA treatment may protect retinal function and attenuate the course of RD in rd10 mice. Biochemical parameters indicate a lower degree of oxidative injury in PABA-treated retinas (Galbinur et al. 2009) . Coexpression of superoxide dismutase 2 (SOD2) and catalase, but neither alone, significantly reduced oxidative damage in the retinas of P50 rd10 mice as measured by protein carbonyl content. Cone density was significantly greater in P50 rd10 mice with coexpression of SOD2 and catalase together than rd10 mice that expressed SOD2 or catalase alone, or expressed neither (Usui et al. 2009) . Systemic treatment of rd10/rd10 mice with a mixture of antioxidants, including α-tocopherol, ascorbic acid, MnTBAP, and α-lipoic acid between P18 and P35, showed preservation of cone function as shown by a significant increase in photopic ERG b-wave amplitudes, and surprisingly showed temporary preservation of scotopic a-wave amplitudes and prolonged rod survival (Komeima et al. 2007) . Members of the insulin family are well-characterized neuroprotective molecules and expression of human proinsulin in rd10 mice attenuates RD, as assessed by the maintenance of the ERG and the histologic preservation of photoreceptors. Systemic proinsulin was able to reach retinal tissue, delay apoptotic death of photoreceptors, and decrease oxidative damage (Corrochano et al. 2008) . Iron is suggested as a potential source of oxidative radicals and it is associated with the RD processes in the rd10 mouse by generating oxidative injury (Deleon et al. 2009; Picard et al. 2010; Jeanny et al. 2013) . Enhanced survival of cones and rods in the retina has been demonstrated through overexpression or systemic administration of human transferrin (hTf) in rd10 mice (Picard et al. 2010; Jeanny et al. 2013) .
7.3.3 Therapeutic Interventions Tested in the rd10 Mouse Model to Improve Photoreceptor Survival and/or Visual Response
In addition to those studies described above in which anti-inflammatory or antioxidant treatment was used to delineate pathological pathways induced by the rd10 mutation, a host of investigators have used the rd10 mutant, with its milder disease progression, to test the efficacy of therapeutic interventions at both the global and pathway levels. For example, environmental enrichment (EE), an experimental manipulation based on exposure to enhanced motor, sensory, and social stimulation, when started at birth, has been shown to exert clear beneficial effects by slowing vision loss. Likewise, early exposure to EE efficiently delayed photoreceptor degeneration in rd10 mutants, extending the time window of cone viability and cone-mediated vision well beyond the phase of maximum rod death (Barone et al. 2012) . Cone counting in retinal whole mounts showed that rd10 EE mice at 1 year had almost three times as many surviving cones (34,000 ± 4000) as the standard (ST) laboratory condition control mice (12,700 ± 1800, t test, p = 0.003). Accordingly, the EE-treated rd10 mutants at 1 year of age were still capable of performing the visual water task under photopic conditions, showing a residual visual acuity of 0.138 ± 0 cycles/degree. This ability was virtually absent in the rd10 ST age-matched mice (0.063 ± 0.014, t test, p = 0.029) (Barone et al. 2014) .
Neurotrophic factors have also been suggested as a potential global approach to promote photoreceptor survival. Long-term expression of glial cell line-derived neurotrophic factor (GDNF) in the rd10 mouse had significant increases in ONL thickness and mean photopic and scotopic ERG b-wave amplitudes compared with that of the untreated rd10 mouse (Ohnaka et al. 2012) . Erythropoietin (Epo), a neurotrophic molecule discovered in the retina, administered systemically leads to morphological photoreceptor protection in the light-damage and RDS/peripherin (Prph2) models of RD. In contrast, no photoreceptor protection was observed following Epo gene transfer in the rd10 model (Rex et al. 2004) . Valproic acid (VPA) is an anticonvulsant and mood-stabilizing drug and it has the potential to increase the expression of neurotrophic factor (NF) genes. A single systemic dose of VPA can change retinal NF such as brain-derived neurotrophic factor (BDNF), GDNF, ciliary neurotrophic factor (CNTF), fibroblast growth factor-2 (FGF2/bFGF), and rod-specific gene expression in the immature retina. Daily VPA treatment from P17 to P28 can also alter gene expression in the mature neural retina. While daily treatment with VPA could significantly reduce photoreceptor loss in the rd1 model, VPA treatment slightly accelerated photoreceptor loss in the rd10 model (Mitton et al. 2014) .
< div class='tao-gold-member'>
Only gold members can continue reading. Log In or Register a > to continue
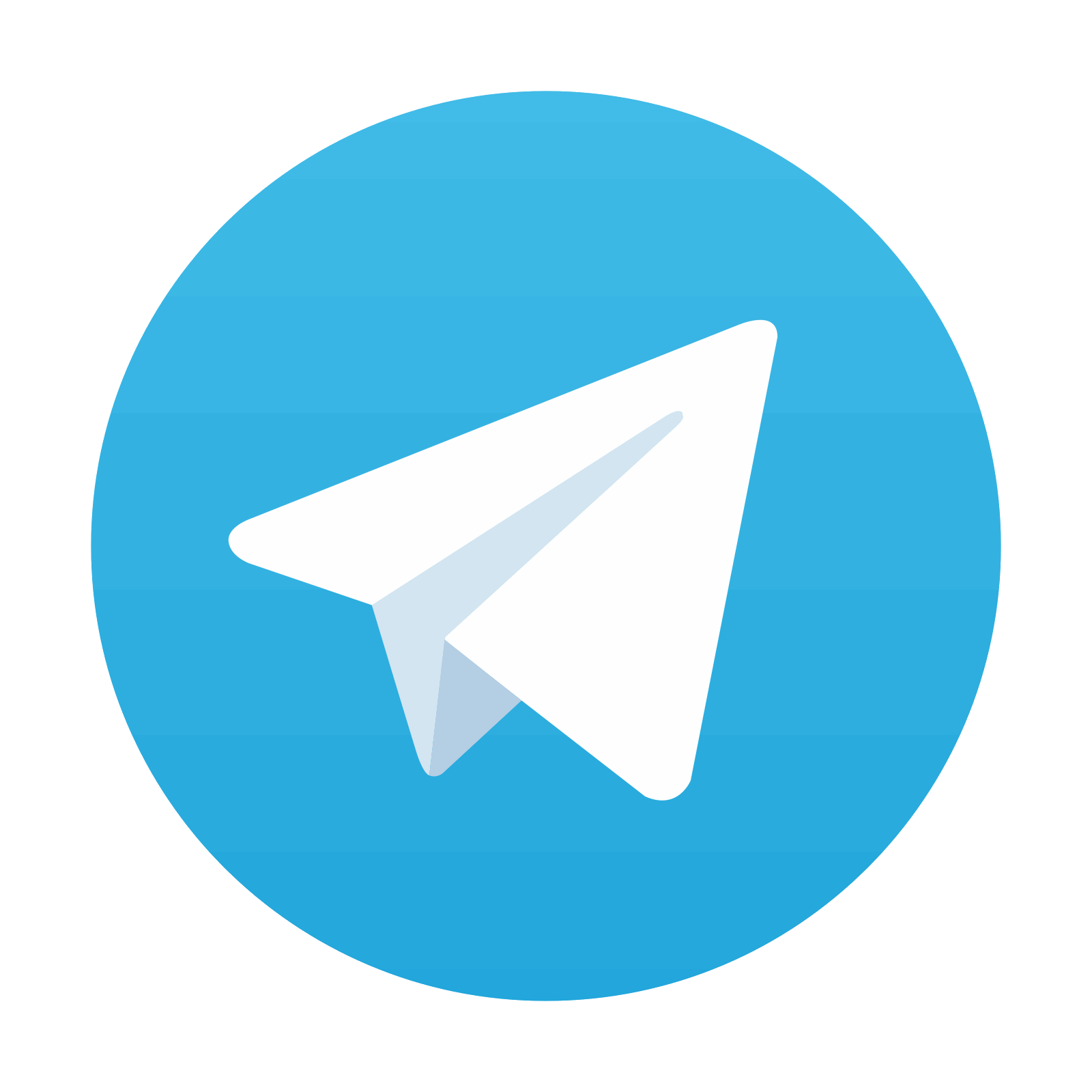
Stay updated, free articles. Join our Telegram channel

Full access? Get Clinical Tree
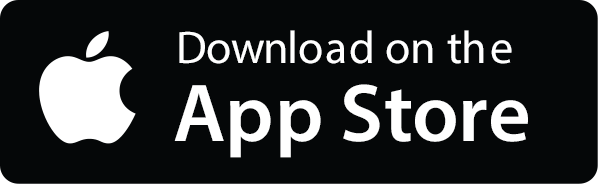
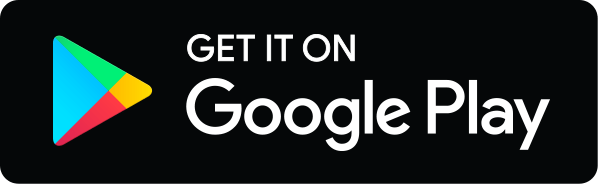