Kara Lascola and Stuart Clark-Price Department of Clinical Sciences, College of Veterinary Medicine, Auburn University, 1220 Wire Road, Auburn, AL, 36849, USA The neonatal foal presenting for general anesthesia is a unique challenge in the equine species. Several reviews of anesthesia for the equine neonate have been previously published, and the reader is directed to those for a global overview of anesthesia of the neonate (Tranquilli and Thurmon 1990; Dunlop 1994; Hubbell and Muir 2009; Fischer and Clark‐Price 2015). This chapter will serve to provide an update to current practices and provide focused information on specific techniques and conditions related to anesthesia of the neonatal foal while still covering some general information. The neonatal period is generally classified as the first four weeks of life and is a period of very rapid physiologic change and adaptation, particularly in the cardiovascular, respiratory, immune, and neurologic systems. Accordingly, these systems function at a reduced capacity to tolerate stresses and disease states can tip the balance into uncompensated illness. Providing anesthesia for these patients thus requires an understanding of the differences in physiology of the neonate from mature horses so that anesthetic plans are tailored to this unique subset of horses. While an exhaustive description of neonatal physiology is beyond the scope of this chapter, aspects of cardiovascular and respiratory physiology that can impact clinical anesthetic management will be discussed. Much of the information provided in this chapter comes from studies in human neonates, as comparatively, literature on anesthesia of the equine neonate is sparse. As the equine fetus nears term, heart rate decreases from 120 beats per minute to 80 beats per minute. This is a result of a baroreceptor reflex from an increase in fetal blood pressure. This indicates a response to increased plasma concentrations of catecholamines, angiotensin converting enzyme, and vasopressin as the fetus readies for birth (Fowden et al. 2020). Interestingly, cardiac baroreceptors sensitivity decreases during the last trimester which suggests that central “resetting” of baroreceptor response occurs after birth in the neonatal period and is thus often described as immature. In the first few moments after birth, a transition from fetal to neonatal (transitional) circulation occurs. Pulmonary vascular resistance is very high in the fetus so that most of the blood ejected from the right ventricle bypasses the lungs and enters the aorta and then systemic circulation. Upon birth, inflation of the lungs causes a drop in pulmonary vascular resistance and blood flows from the right ventricle into the pulmonary circulation causing a pressure drop and a narrowing of the ductus arteriosus. Left atrial pressure rises and right and left heart volume and ventricular output equalize causing closure of the foramen ovale. The ductus arteriosus further constricts as oxygen tension increases and placental‐derived prostaglandins decrease (Marr 2015; Saikia and Mahanta 2019). In horses, the ductus arteriosus may be patent in normal healthy foals up to one week of life (Fries et al. 2020). A rise in pulmonary vascular resistance (pulmonary hypertension) in foals can cause the transitional circulation to revert to fetal circulation and result in tissue hypoxia (Cottrill et al. 1987). The neonatal heart contracts at near maximal effort because of a large release of catecholamines and thyroid hormone late in gestation and at birth. Neonates need a larger cardiac output relative to the adult horse to provide oxygen and nutrients to growing tissues with very high metabolic demands. As a result, stroke volume remains relatively constant and cardiac output is mainly a product of changes in heart rate (Saikia and Mahanta 2019). Therefore, there is very little cardiac reserve in myocardial performance and small decreases in heart rate can produce large reductions in cardiac output. Sedative, anesthetic, and analgesic drugs that decrease heart rate can therefore have clinically relevant effects on the cardiovascular system and may alter patient management (see the following text). Neonatal lung physiology and pulmonary mechanics are different from older foals and adult horses (Neumann and von Ungern‐Sternberg 2014). Immaturity of the respiratory center in the brain stem and peripheral and central chemoreceptor responses lead to irregular breathing patterns that can present anywhere from tachypnea to long periods of apnea. This results from an inefficient and easily impaired response to blood pH and carbon dioxide and oxygen tensions (PaCO2 and PaO2). The response to hypercapnia can be attenuated without an appropriate increase in tidal volume and/or ventilation rate while a biphasic response to hypoxia can be seen with an initial increase in ventilation rate and a subsequent decrease that may progress to apnea. The addition of sedative and anesthetic drugs can further blunt these responses (Kurth et al. 1987). Additionally, neonates can have an exaggerated Hering‐Breuer inflation reflex in that activation of stretch receptors in the lungs and airways can result in vagal inhibition of the respiratory centers of the brain stem (Stocks et al. 1996). Anatomically, compliance of the pharynx, larynx, trachea, and bronchial tree is greater in neonates and can lead to dynamic airway collapse, particularly during inspiration (Neumann and von Ungern‐Sternberg 2014). Because of small airways, luminal material such as blood or secretions can greatly increase the work of breathing which can be more difficult on neonates due to a highly compliant chest wall compared to older horses. Newborn foals have fewer alveoli and alveolar surface area compared to one‐month‐old foals and adult horses (Johnson et al. 2014). As such, neonates can develop atelectasis more easily in dependent lung regions than more mature horses. Additionally, ill foals may have reduced production of pulmonary surfactant, which can further affect alveolar opening. A compliant and deformable chest wall result in a larger portion of the energy generated from diaphragmatic contraction to be wasted on thoracic distortion (Neumann and von Ungern‐Sternberg 2014). Immaturity and weakness of the diaphragm and intercostal muscles also contribute to the potential for respiratory insufficiency, and this can be further potentiated with the addition of sedative and anesthetic agents. Functional residual capacity (FRC) is lower in neonates than in adults. This can lead to reduced oxygen reserves and higher chance of hypoxemia. Additionally, due to lower elastic properties of lungs, closing volume in neonates can be less than FRC resulting in terminal closure of airway (Mansell et al. 1972). Anesthetized neonatal foals may require assisted or mechanical ventilation due to an inability to maintain appropriate PaO2 and PaCO2. Lung‐protective strategies for ventilation of neonatal lungs are recommended to minimize the possibility of ventilator‐induced lung injury (VILI) (Neumann and von Ungern‐Sternberg 2014) (see the following text). Anesthesia of a foal is not simply anesthesia of a small horse. Physiologic and immunologic immaturity, unique disease conditions and responses to illness, and size differences can make general anesthesia more challenging. Indeed, horses less than one month of age have an increased risk of dying in the peri‐operative period (within seven days after surgical procedure) compared to young adult horses (Johnston et al. 2002). This may be because of a lack of familiarity with providing anesthesia for foals, lack of pharmacokinetics and pharmacodynamics information of drugs used in neonates, and that neonates are most commonly anesthetized for correction of serious illnesses. Appropriate planning, preparation, and equipment are key factors for increasing the odds of successful outcomes. Knowledge of signalment, history, results of diagnostic tests, medications administered, and a thorough physical examination are the mainstay of pre‐anesthetic evaluation of foals and are critical when formulating an anesthetic plan. The breed of the foal is important as genetic disease in familial lines may help identify foals that are potentially at higher risk for anesthetic complications. An inclusive list of genetic disorders that may clinically manifest in foals is beyond the scope of this chapter. However, some examples include hyperkalemic periodic paralysis in Quarter horses, fragile foal syndrome in Warmbloods, glycogen branching enzyme deficiency in Quarter horse and Paint breeds, polysaccharide storage myopathy, and malignant hyperthermia (Traub‐Dargatz et al. 1992; Finno et al. 2009; Martin et al. 2020). Inquiring about the mare’s health during pregnancy, primiparous or multiparous status, lactation, the foal’s gestational age, birth location and delivery, and immediate post‐partum activity should be routine to determine if developmental delays and/or failure of passive transfer may have occurred. It is also important to determine if any medications have been administered prior to the foal presenting for anesthesia. Previously administered antimicrobial, sedative, or analgesic drugs may negatively interact with drugs in an anesthetic plan and may require adjustments. Sample collection for clinical pathological diagnostic tests to assess immune function and status, hydration and nutrition, acid–base and electrolyte balance should be performed to establish baselines and repeated at necessary intervals to monitor health status. A complete blood count, biochemistry panel, electrolyte analysis, and arterial blood gas may be necessary to assess function of various physiologic systems. Additionally, samples for blood culture should be acquired before initiating antimicrobial therapy if septicemia is suspected. Quantification of plasma IgG concentration should be assessed for status of passive transfer of immunoglobulins from colostrum. Thoracic and abdominal radiographs and ultrasound can be utilized to assess for rib fracture, pneumonia, abscesses formation, free fluid (samples should be collected for diagnostic tests), or structural abnormalities that may require advances therapeutic techniques. Physical examination of a foal should be through and systematic with a focus on the neurologic, cardiovascular, respiratory, and urinary systems. Select normal physical exam and clinical pathology parameters can be found in Table 13.1. Abnormalities detected during physical examination should be investigated for identification of underlying cause and corrective or stabilizing therapies employed to the greatest extent possible prior to an anesthetic event. Neonatal foals usually present with their dam and separation for anesthesia and surgery can be a stressful event for the mare, foal, and personnel. Some mares will become agitated and potentially dangerous when foals are handled and/or removed from the mare’s line of sight. Facility procedures and protocols that maximize personnel safety should be followed at all times when working around a mare and foal pair. If the foal is ambulatory, then walking the mare and allowing the foal to follow to the induction area prior to premedication may minimize stress and anxiety. If the foal is not ambulatory, premedication in the stall will sedate the foal and allow for transportation on a gurney without flailing. Sedation of the mare is frequently necessary to minimize vocalization, agitation, aggressive behavior, and stall walking and can be done with a combination of xylazine or detomidine with acepromazine for immediate sedation and longer duration tranquilization during the period the foal is absent. Separation should be held off as long as possible until right before premedication and induction and the foal should be allowed to nurse up until just before sedation to minimize to potential for hypoglycemia. Once sedative medications are administered, foals should be prevented from nursing to reduce the risk of aspiration of milk. After induction of the foal, the sedated mare can be returned to the stall. Table 13.1 Select normal reference ranges for various physiologic and clinical pathology parameters in neonatal foals (7–12 days of age). Commonly used sedative, analgesic, and anesthetic agents can have a clinically relevant impact on physiologic systems and can lead to an increase in morbidity and mortality in equine patients. In healthy, mature horses, there are adequate physiologic reserves to allow for safe compensation of these effects. However, the equine neonate can be particularly susceptible to adverse anesthetic events because of immaturity in physiologic systems and thus requires careful titration of dosages and intensive monitoring for physiologic parameter deviations. Statements can be found in literature suggesting that the neonatal blood–brain barrier is immature, leaky, or even absent in the neonate and thus is more permeable to anesthetic/sedative agents. However, more recent evidence suggests that this barrier is impermeable and that tight junctions are formed very early in fetal life (Ek et al. 2006; Saunders et al. 2019). There is little doubt that a developing brain is more vulnerable to drugs and toxins than an adult brain, but this appears to be related to a higher transport capacity of transporter proteins in the central nervous system (Saunders et al. 2019). Perinatal hypoxic–ischemic‐related brain injury is associated with pathologic disruption of the blood–brain barrier (Disdier and Stonestreet 2020). Thus, foals with perinatal asphyxia syndrome (PAS) should be treated as though they have disruption of the blood–brain barrier with further considerations of risk versus benefit and dosages for drug selection. Common sense implies that reduced dosages and use “to effect” should be applied to anesthetic/sedative drug administration to neonatal foals. Dosages of various sedative, analgesic, and anesthetic agents used in neonatal foals can be found in Table 13.2. In adult horses, reliable, fast acting sedation is necessary to improve handling and the quality of anesthesia. However, with neonatal foals, many of the commonly used sedatives can have detrimental effects on an immature cardiovascular system. The most commonly used classes of sedative in horses are alpha‐2 agonists and phenothiazines. The alpha‐2 agonists, xylazine, detomidine, and romifidine, are favored in adult horses because of their reliable, quick acting sedation and analgesia (Sanchez and Robertson 2014; Moorman et al. 2019). However, drugs in this category can have profound effects through alpha‐receptor agonism. In healthy foals up to 28 days of age, xylazine administration can result in bradycardia, hypertension and then hypotension, upper airway obstruction, ventilatory stridor, decreased minute ventilation, marked ataxia, and recumbency (Carter et al. 1990). A reduction in cardiac output associated with alpha‐2 agonist use is well known and likely to be more profound in foals as their cardiac output is more heart rate dependent than mature horse. Alpha‐2 agonists in horses may negatively affect gastrointestinal motility and perfusion and increase post‐glomerular diuresis that may be detrimental to fluid and electrolyte balance, particularly in compromised patients (Rutkowski et al. 1991; Sutton et al. 2002; Nuñez et al. 2004; Zullian et al. 2011). Due to the immature cardiovascular status of neonatal foals and the likelihood of clinically relevant disease, minimizing the use of alpha‐2 agonist drugs, whenever possible, is recommended. Acepromazine, a phenothiazine, is a centrally acting dopamine receptor antagonist that is useful as a tranquilizer in horses. Onset of action is up to approximately 20 minutes and has a dose‐dependent duration of action of 1–2.5 hours in horses (Marroum et al. 1994). Additionally, packed cell volume is reduced by up to 20% and blood pressure significantly decreases (Marroum et al. 1994). Due to its long duration of action, lack of a reversal agent, and actions on the circulatory system, acepromazine has not gained wide popularity for sedation in neonatal foals. Benzodiazepines have centrally acting muscle relaxation and sedative effects through enhancing the effects of the inhibitory neurotransmitter γ aminobutyric acid (GABA) on chloride and other ion channels. Benzodiazepines have minimal clinically relevant effects on cardiovascular or ventilatory performance. Additionally, drugs in this class have potent anticonvulsive activity which may be helpful in foals with neonatal asphyxia (Johne et al. 2021). Traditionally, benzodiazepines such as midazolam and diazepam are combined with ketamine for their muscle relaxant properties during induction of anesthesia in horses. In adult horses, benzodiazepines provide little to no sedation but can produce ataxia, anxious, agitated, or aggressive behavior and are not recommended as a single therapy (Muir 2009; Fischer and Clark‐Price 2015). However, in foals less than two to four weeks of age, benzodiazepines can produce profound sedation, relaxation, and recumbency (Fischer and Clark‐Price 2015). Midazolam may be preferred over diazepam due to formulation differences. Diazepam is not water‐soluble and is therefore found with propylene glycol as a carrier agent. At high doses, propylene glycol can be tissue irritating and cause necrosis, hemolysis, and cardiac arrhythmias (Lim et al. 2014). Midazolam gives the benefit of being water‐soluble and thus formulations are made without propylene glycol. Midazolam can be administered intramuscularly if intravenous access is limited. Additionally, intranasal midazolam is reported to be effective for seizure control in dogs (Charalambous et al. 2017) and though not published, anecdotal reports suggest similar anti‐seizure and sedative effects when administered intranasal in foals. The pharmacokinetics of midazolam have been well described in adult horses at dosages of 0.05 and 0.1 mg/kg (Hubbell et al. 2013). However, the pharmacokinetics have not been described in juvenile or neonatal horses. Neonatal foals presenting for anesthesia frequently have disease that may have an immunological component (i.e. failure of passive transfer, sepsis). Midazolam has been shown to suppress phagocytosis and oxidative burst, essential functions of the innate immune system, in equine neutrophils, and macrophages; however, it is unknown if this has any clinical implications (Massoco and Palermo‐Neto 2003). Table 13.2 Dosages of sedative, analgesic, anesthetic, and adjunctive drugs for use in neonatal foals. It is important to recognize that, at birth, animals have a developing nociceptive system. Although not completely mature, neonatal animals are able to process and interpret pain sensations. In fact, the ability to “feel” pain occurs early in the second half of gestation (Bellieni 2020). The central nervous system is quite plastic at birth, and structural and functional “fine‐tuning” of the nociceptive system continues as the animal develops. The spinal circuitry is activity dependent and therefore there can be long‐term detrimental consequences to untreated pain (van den Hoogen et al. 2019). Untreated pain can lead to neurotoxicity, alterations in spinal neuronal circuits, and apoptosis of developing neuronal brain cells (van den Hoogen et al. 2019). Questions continue to surround the use of opioids as analgesics in horses with behavioral changes (aggression, sedation), gastrointestinal stasis, and respiratory depression during anesthesia being main points of contention. Butorphanol, a κ agonist – μ antagonist, is the most commonly used opioid in horses. Butorphanol has been evaluated in healthy neonatal foals at 0.05 mg/kg and although the pharmacokinetics are different from adult horses (greater volume of distribution and faster clearance in neonates), it appears to be well tolerated with minimal effects on vital parameters (Arguedas et al. 2008). Clinical signs of sedation and increased nursing behaviors can be expected to occur. Of the numerous μ agonist opioids available for clinical use, there is little published data in neonatal foals. Anecdotal use of morphine suggests that it provides clinically relevant sedation when combination with midazolam as a premedication prior to surgery. Fentanyl, a μ agonist opioid, is considered 100 times more potent than morphine and is used commonly as an infusion during anesthesia for surgery in small animal patients. Fentanyl has been studied in healthy foals in a dose escalation manner (Knych et al. 2015). At dosages greater than 4 μg/kg, foals develop ataxia, muscle rigidity, and may head press. Foals become heavily sedated and recumbent at 32 μg/kg. It is important to note that efficacy studies with opioids have not been performed in neonatal foals and clinical use is frequently based on clinical experience and extrapolation from adult horses and other species. Local use of opioids, particularly intra‐articular use of preservative free morphine, may allow for a reduced dosage and minimizing systemic effects while still benefiting from the analgesic properties. In the authors’ opinion, μ agonist opioids should be considered for routine use in anesthetized neonatal foals that are undergoing invasive, painful procedures as the negative effects of pain outweigh the potential negative effects of opioid use. Ketamine continues to be the induction agent used most commonly in adult horses as it provides rapid unconsciousness, can be administered in a reasonable volume, and provides for a smooth and controlled induction. However, in neonatal foals, the concern about controlling a large body mass during induction is not as problematic as an adult horse and therefore allows for more options for induction. When inducting a neonatal foal for anesthesia, quick control of the airway is essential to provide oxygen and ventilatory support as hypoxemia can rapidly occur. In human infants, hypoxemia during anesthesia is recognized as a critical factor that increases morbidity and mortality (Disma et al. 2021). Intubation can be quickly accomplished with the use of inhalants, ketamine, propofol, or alfaxalone in neonatal foals. Inhalant anesthetics has been used for induction of anesthesia in foals (Steffey et al. 1991). A facemask with an airtight seal can be applied over the nose of a foal for induction, but in depressed or gently restrained foals, nasotracheal intubation can be performed in the awake animal. This requires use of a long, straight, silicone endotracheal tube with an internal diameter of 6–14 mm depending on the size of the foal (Figure 13.1). Soaking the endotracheal tube in warm water to soften and applying water‐based lubricant to the outer surface will facilitate insertion. Additionally, desensitizing the nasal mucosa with lidocaine may improve compliance from the foal. Once placed, the endotracheal tube can be attached to a breathing circuit on an anesthesia machine, and oxygen and inhalant anesthetic can be administered for induction. Inhalant anesthetics cause dose‐dependent vasodilation and decreased cardiac output through reduced stroke volume in adult horses and similar changes should be expected in foals (Steffey and Howland 1980; Steffey et al. 2005). Careful titration of the dose of inhalant anesthetic during induction and maintenance of anesthesia while minimizing side effects can be achieved through attentive physiologic parameter monitoring of the foal. Figure 13.1 Various sized silicone tubes that can be utilized for nasotracheal or endotracheal intubation in neonatal foals. Ketamine, a dissociative anesthetic, induces anesthesia through non‐competitive interaction with N‐methyl‐D‐aspartate receptors in the central nervous system. Ketamine administration results in maintenance or even elevation of heart rate and blood pressure through increased release and inhibition of reuptake of endogenous catecholamines like epinephrine. After a relatively small volume for injection, a predictable pattern of induction is observed in adult horses. However, ketamine use, particularly in short procedures can result in excitement and dysphoria during the recovery period and more recently, research in laboratory animals suggests that ketamine can increase neuronal apoptosis in developing brains and produce long‐term cognitive impairment, even after a single dose (Blaise 2015; Sampaio et al. 2018). To date, no research has been published in equine neonates, so it is unknown how ketamine effects the developing foal brain. Propofol is a unique phenolic anesthetic agent in that it is structurally distinct from all other injectable anesthetic drugs. It acts as an agonist at the GABA complex resulting in cell hyperpolarization. Propofol has the favorable properties of a rapid, smooth induction and a short, delirium free recovery. Propofol has administration rate and dose‐dependent adverse effects on spontaneous ventilation and blood pressure. Slow infusion at the lowest dosage necessary for intubation can minimize hypoventilation and hypotension. While research in adult horses is available, published studies on use of propofol in neonatal foals is limited. Anecdotal reports indicate that veterinarians are using propofol as an induction agent for foals more commonly. However, similar to ketamine, studies in laboratory animals suggest that propofol may have toxic effects in a developing brain. Repeated injections but not a single injection of propofol in neonatal mice resulted in long‐term motor and behavioral learning impairment (Zhou et al. 2021). The combination of ketamine and propofol (“ketofol”) has been described for use in human neonates and healthy adult dogs (Martinez‐Taboada and Leece 2014; Hayes et al. 2021). In human neonates, the combination may result in a small reduction in the risk of hypotension, bradycardia, and apnea, with a slight increase in tachycardia. In adult dogs, ketofol induction resulted in a higher pulse rate and mean arterial pressure with a quality of induction and ease of intubation greater than propofol alone. The authors have used this combination to induce anesthesia in foals, and clinical impression is that it achieves a smooth induction and a stable plane of anesthesia. Alfaxalone is a steroidal anesthetic agent with a physical structure similar to progesterone. Alfaxalone is thought to work through interaction with GABA. Alfaxalone has been used as an induction agent in adult horses with an induction time shorter than ketamine but with a more prolonged recovery time (Wakuno et al. 2017). Total intravenous anesthesia with alfaxalone has been reported in healthy foals and one donkey foal (Jones et al. 2019; Loomes 2020). Prolonged recovery was noted. Pharmacodynamic data in foals suggests clinically acceptable heart rate and blood pressures during anesthesia after a single injection; however, oxygen supplementation may be required to prevent hypoxemia (Goodwin et al. 2012). Alfaxalone is not approved for use in humans, and there are currently no studies available assessing neurotoxicity in developing brains. For short procedures (<30 minutes), maintenance of anesthesia may be provided with additional doses of injectable anesthetics such as propofol or alfaxalone. Oxygen/ventilatory support may be necessary due to the respiratory depressant effects of the drugs combined with the foal’s physiologic immaturity. For major surgical procedures or the need for prolonged anesthesia (>30 minutes), inhalant anesthetics isoflurane and sevoflurane are the mainstay. This requires tracheal intubation and the use of an anesthesia machine. Many of the large animal anesthesia machines that are commonly used for adult horses are not suitable for anesthesia of foals weighing less than 330 lbs. (150 kg). The large volume of the breathing circuit in these machines makes quick adjustment of anesthetic vapor concentration nearly impossible and the larger one‐way valves may result in clinically relevant resistance to breathing. Additionally, for foals that require mechanical ventilation, large animal anesthesia machine bellows may not safely accommodate smaller tidal volumes, flow rates, and pressures necessary to prevent lung injury. Standard small animal anesthesia machines and mechanical ventilators can be utilized for safe delivery of anesthetic. More recently, an electronic large animal anesthesia machine with a precision motor piston driven ventilator became available on the veterinary market (Tafonius, Hallowell Engineering and Manufacturing Corporation). With the use of a “foal circuit,” very precise ventilation utilizing various modes of ventilation can be applied for use in foals. The minimum alveolar concentration (MAC), common use, and side effects of isoflurane (MAC = 1.31) and sevoflurane (MAC = 2.84) have been well described in adult horses (Steffey et al. 1977, 2005; Brosnan 2013). MAC values of isoflurane and sevoflurane in foals have not been reported. However, MAC values of inhalant anesthetics are decreased in human term neonates less than one month of age and even further decreased in human pre‐term neonates (LeDez and Lerman 1987). Thus, neonatal foals likely require lower concentrations of isoflurane and sevoflurane similarly and practitioners are advised to adjust vaporizers downward in neonatal foals during anesthesia to minimize excessive anesthetic depth. Previously mentioned, cardiovascular side effects of vasodilation and decreased stroke volume can be problematic and techniques (use of supplemental analgesic drugs) that allow for a reduced concentration of inhalant to be administered should be considered. Ventilatory changes can also be expected to occur in neonatal foals during inhalant anesthesia. Hypoventilation progressing to apnea should be expected as inhalant dose increases. The neonatal brain has an altered response to elevations in carbon dioxide resulting in irregular breathing patterns (Neumann and von Ungern‐Sternberg 2014). Further blunted by inhalant anesthetics, a weak respiratory drive frequently results in the need for assisted or mechanical ventilation of foals. In 2016, the United States Food and Drug Administration issued a warning that repeated or lengthy (>3 hours) general anesthesia in children less than three years of age may affect the development of the child’s brain (Olutoye et al. 2018). This statement was made due to mounting evidence of anesthetic toxicity in the developing brains of research animals. Drugs in two classes of sedative/anesthetic agents were implicated: GABA agonists (e.g. inhalant anesthetics, benzodiazepines, and propofol) and N‐methyl‐D‐aspartate (NMDA) antagonists (e.g. ketamine). Neuronal anesthetic toxicity can lead to structural, functional, and compensatory changes in sensory and learning systems (Aksenov et al. 2020). Neurotoxicity of inhalant anesthetics appears to be through enhancement of apoptosis in various parts of the brain that can lead to cognitive deficits (Jevtovic‐Todorovic et al. 2003). This phenomenon appears to occur only in developing brains, as inhalants do not have similar effects in mature brains (Stratmann et al. 2010). To date, there is no information on the effect of anesthetic drugs on the development of the equine brain. However, in light of the recommendations in human neonates, it seems prudent that practitioners should limit the duration and number of anesthetic episodes and the dosages of sedative and anesthetic drugs used in foals as much as possible. Depending on the physical condition of the foal, the anticipated length of the anesthetic event and the procedure for which it is being anesthetized for, the level of intensity of monitoring and support varies. It should be stressed that regardless of the procedure, physical assessment of the foal should be performed at regular intervals as physical status of neonatal foals can change rapidly. This included thoracic auscultation of the heart and lungs, palpation of pulse quality, mucous membrane color, and capillary refill time. For procedures or diagnostic of less than 15 minutes, nasal insufflation of oxygen, and the addition of pulse oximetry to physical assessment may be all that is required. For longer or invasive procedures, use of a vital parameter monitor including electrocardiogram, end‐tidal gas analysis, pulse oximetry, and direct arterial blood pressure measurement should be considered. The goal of cardiovascular monitoring is assessing the adequacy of circulation and tissue perfusion. Heart rate and rhythm (ECG), mucous membrane color and capillary refill time, peripheral temperature, arterial blood pressure, and blood lactate concentration are useful tools for assessment. Dark mucous membranes, prolonged capillary refill times, cool extremities, and elevated lactate concentrations can all suggest poor perfusion and indicate the need for intervention. Likewise, decreases in heart rate and blood pressure can indicate depressed pump function. Resting values for heart rate and blood pressure change during the first few weeks of a foal’s life as physiologic growth and maturity progress. Foals can be expected to have higher heart rates and lower blood pressure in the first week as compared to the second week, and younger foals have a decreased corrective response to anesthetic‐induced hypotension (O’Connor et al. 2005). Vital signs should be interpreted based on the foal’s age, and more aggressive therapies to combat hypotension may be necessary in younger foal. Arterial blood pressure can be measured via indirect methods with an appropriately sized cuff placed around the base of the tail. However, similar to other species, indirect blood pressure measurement is likely not suitable for decision making after a single measurement and only useful for detecting trends over time. However, as the cardiovascular status of anesthetized foals can change quickly, the authors recommend direct blood pressure measurement from an arterial catheter for systemically ill foals or any foal undergoing general anesthesia for more than 30 minutes. Arterial catheters can be placed in the transverse facial, lateral metatarsal, lingual, or femoral arteries (Figure 13.2) (Fischer and Clark‐Price 2015). In adult horses, a target value for mean arterial blood pressure of 70 mmHg or greater has been associated with a decrease in morbidity and mortality, particularly associated with equine peri‐anesthetic myopathy (Schauvliege and Gasthuys 2013). Neonatal foals likely do not need to maintain mean arterial blood pressures of 70 mmHg or greater. Cardiovascular studies in foals for recommendation of arterial blood pressure are not available. However, in anesthetized human neonates, a 20% reduction in systolic blood pressure compared to awake baseline resulted in a less than 10% chance of cerebral desaturation (Michelet et al. 2015). When awake neonatal foal blood pressure is considered, the human study suggests that mean arterial blood pressure of >50 mmHg may be acceptable in anesthetized foals. Figure 13.2 Cannulation of the facial artery in a foal during anesthesia for direct measurement of arterial blood pressure. Cardiac output monitoring is not routinely performed in clinical veterinary patients. However, more recently, an ultrasound dilution (UDCO) method has been developed for human infants that utilizes a peripheral venous and arterial catheter. Invasive heart catheterization or loss of blood that is necessary for other methods is not needed for UDCO. This method has been validated for use in foals to assess cardiac function and has the advantage of being useful in awake and anesthetized foals (Shih et al. 2009; Fries et al. 2020). For critically ill foals, measurement of cardiac function with UDCO before, during, and after anesthesia may help detect cardiac performance changes early and guide goal‐directed therapy. Hypotension during anesthesia may be related to vasodilation, hypovolemia, bradycardia, and/or decreased contractility (decreased stroke volume). Treatment aimed at hypotension should be directed at an identified underlying cause and not “shot gun” therapy aimed at improving a number on a vital signs monitor. As previously stated, foals have minimal cardiovascular reserve and thus heavy‐handed administration of corrective therapies may result in further worsening of cardiovascular function and patient health status. Hypotension may be simply related to the anesthetic agents used, and decreasing the plane of anesthesia may allow for acceptable correction of parameters. Hemodynamic instability related to shock may be observed in any anesthetized foal as most anesthetic episodes are related to correction of diseases that have systemic impact. Shock in neonates is most commonly diagnosed only after the onset of the hypotensive phase which indicates serious disease progression. Early identification and intervention is key to successful management (Singh et al. 2018). Ideally, identification of potential or the onset of shock occurs early on and the foal is stabilized prior to anesthesia (see sepsis in the following text). Once meaningful hypotension is identified, a systematic examination for cause should be performed. A combination of physical parameters (anesthetic depth, capillary refill time, mucous membrane color, extremity temperature), vital sign monitor parameters (heart rate and rhythm, pulse oximetry, end‐tidal CO2) and clinical pathology findings (blood gas, electrolyte, lactate, and hematocrit) should be utilized for determining course of action. While anticholinergic drugs (atropine, glycopyrrolate) have traditionally been avoided for use in adult horses due to concerns surrounding gastrointestinal stasis, use to accelerate heart rate in foals may be sufficient to counter anesthetic‐induced bradycardia. Hypovolemia should be corrected with intravenous fluid therapy to correct losses. However, overaggressive use of fluids may result in fluid overload and hypernatremia (Fielding and Magdesian 2015). Fluid boluses of 20 ml/kg have been suggested and should be repeated up to three times (Fielding and Magdesian 2015). Goals for fluid therapy are a blood lactate concentration less than 4 mmol/l, normalization of perfusion parameters, and urine production. If hypotension persists after fluid therapy, infusion of inotropic and/or vasopressors should be instituted. Dobutamine is used for inotropic support, and norepinephrine is used for vasopressor support (Hollis et al. 2006; Valverde et al. 2006; Fielding and Magdesian 2015). The combined use of dobutamine and norepinephrine in foals has been described and has been used by the authors for cardiovascular support in severely ill foals that did not have adequate response to single therapy (Hollis et al. 2006). Vasopressin increases vascular resistance and blood pressure in anesthetized foals; however, splanchnic circulation is decreased resulting in a potential decrease in gastric mucosal perfusion (Valverde et al. 2006). The unique respiratory physiology of the neonatal foal and the depressant effects of sedative, analgesic, and anesthetic drugs, often necessitate the use of assisted or mechanical ventilation during anesthesia. Adequacy of ventilation in the anesthetized foal can be assessed with a combination of pulse oximetry, end‐tidal gas determination, and arterial blood gas analysis. Hypercapnia (PaCO2 > 55 mmHg, pH ≤ 7.25) is usually an indicator of hypoventilation while hypoxemia is usually an indicator of a reduced gas diffusion across the alveolar‐capillary barrier. Some indications for mechanical ventilation during general anesthesia in the neonatal foal include persistence of hypoxemia and/or hypercapnia, use of neuromuscular blocking agents, thoracic procedures or imaging studies, irregular ventilator pattern that impedes uptake and maintenance of inhalant anesthetic, and spontaneous movements of the thoracic wall or abdomen that impedes a surgical procedure. Due to the immaturity of a foal’s lungs, lung‐protective strategies that minimize VILI should be considered. VILI can result from excessively high tidal volume (volutrauma), higher pressures during ventilation (barotrauma), shear forces damaging lung that is insufficiently opened (atelectotrauma), subsequent release of pro‐inflammatory cytokines, and high levels of oxygen resulting in oxidative stress (oxygen toxicity) (Neumann and von Ungern‐Sternberg 2014). Lung‐protective ventilation strategies that avoid high tidal volume and airway pressure, use of recruitment maneuvers, and preventing repetitive opening and closing of alveoli through use of positive end‐expiratory pressure are recommended (Table 13.3). Fluid, electrolyte, and acid–base status is monitored via analysis of blood samples. Shifts in parameters can happen rapidly and frequent sampling can allow for identification and initiation of therapies aimed at correction and balance. Derangements in sodium, chloride, and potassium are common in sick foals and fluid and electrolyte balance may vary depending on the disease (see below for treatment recommendations for specific diseases and conditions). Correction of fluid deficits and electrolyte imbalance should take place prior to inducing anesthesia whenever possible. While foals have a higher percentage of total body water than adult horses (10%), foals have a lower colloidal oncotic pressure, that, when accompanied with excessive exogenously administered fluids, can result in rapid development of edema (Magdesian 2013). Therefore, high volumes of fluids (other than fluid boluses needed for hypotension) should not be administered during anesthesia. If daily maintenance fluids are being administered as part of a treatment plan, those can be continued at a similar rate, or if maintenance fluids are not being administered, fluid rates of 2–5 ml/kg/h can be administered during anesthesia. While initial replacement fluids should utilize isotonic fluids such as lactate Ringer’s solution, maintenance fluids before and during anesthesia should be comprised of hypotonic fluid solutions. Hypotonic fluids with supplementation of potassium (based on serum potassium concentrations) and dextrose (based on serum glucose concentrations) are thought to be more appropriate than isotonic fluids (Dathan and Sundaram 2021; Fielding and Magdesian 2015). Daily sodium requirements are quickly met with oral and intravenous fluid therapy in foals; thus, the use of isotonic fluids results in sodium retention secondary to a foal’s inability to excrete large amounts of sodium quickly. Commercial maintenance fluids are hypotonic (approximately 110 mOsmol/l) and can be utilized or standard isotonic crystalloid fluids (approximately 295 mOsmol/l) can be diluted with sterile water to make a hypotonic solution similar to commercial products. If potassium supplementation is required, potassium phosphate may be used to supplement the maintenance fluids instead of potassium chloride to minimize hyperchloremia. For older healthy foals (>2 weeks), standard isotonic solutions can be utilized. Table 13.3 Lung‐protective mechanical ventilator strategies for neonatal foals. Source: Adapted from Neumann and von Ungern‐Sternberg (2014). Dextrose support will likely be needed during anesthesia of neonatal foals, as glycogen storage is not sufficient to maintain appropriate blood glucose levels. Blood glucose should be checked at regular intervals (30–60 minutes) during anesthesia and adjustments made to intravenous fluid dextrose concentrations to maintain blood glucose concentrations between 150 and 250 mg/dl during anesthesia. Blood glucose in awake foals should be maintained at approximately 150 mg/dl. Dextrose can be added to the intravenous fluids to achieve a concentration of 1–5%. Solutions greater than 5% can be hyperosmolar and result in hyperglycemia, lysis of red blood cells, and osmotic diuresis, and should be avoided. Plasma can be utilized for foals with a low oncotic pressure and/or hypoalbuminemia. In adult horses, plasma can be cost prohibitive for colloidal support but in foals, 20–40 ml/kg of fresh frozen plasma may be feasible. In cases of failure of passive transfer, hyperimmune plasma may be used for immunoglobulin replacement and immune therapy. However, failure of passive transfer should be corrected well before anesthesia and surgery is considered as these foals are at high risk for infections and sepsis. Inadvertent peri‐anesthetic hypothermia (IPH) is one of the more common adverse events associated with general anesthesia. IPH is associated with delayed recovery, poor wound healing, increased risk of infection, altered coagulation, and hypotension (Clark‐Price 2015). Neonatal foals have a larger surface area to body mass ration than older horses and have less skeletal muscle mass, thus they lose body heat more rapidly and have less capacity to generate heat. Additionally, hypothermia is further exacerbated by the impairment of thermoregulatory centers in the brain stem (Sessler 2015). Monitoring the body temperature of foals is therefore an essential part of an anesthetic plan. Aggressive heat support is necessary throughout the anesthetic period and during recovery. During anesthesia, use of active warming techniques (circulating warm water pads, force warm air blankets, resistive polymer electric blankets) that cover as much of the foal as possible can help reduce the temperature gradient from the foal to the environment and minimize hypothermia. Similarly in recovery, use of active warming as well as covering the foal in prewarmed blankets can be used to reduce recovery time and complication. The goal of the recovery period is to transition the foal to an awake and standing state as quickly as possible. This will allow the foal to be returned to the mare and nursing to maintain blood glucose. Recovery of a foal can be done in either a dedicated recovery stall or in a stall with the mare nearby. Recovery in a stall with the mare is not recommended to prevent injury to personnel and the foal by an agitated mare. The authors prefer to recovery foals in a warm, quiet, and padded recovery stall and then bring the mare to the foal once the foal is standing. The mare and foal can be walked back to the wards once the foal is standing and has nursed. Foals should be placed in sternal recumbency to promote resolution of atelectasis. As stated previously, warming the foal will help reduce recovery time; however, reversal of sedative drugs may be necessary if recovery is prolonged. In general, foals should be fully recovered within one hour after discontinuing inhalation anesthetics. Intravenous fluids for electrolyte and glucose support can be continued during recovery and supplemental oxygen via nasal insufflation can be utilized to maintain SPO2 values greater than 92%. Neonatal foals can be manually restrained during the recovery period by having control of the head and keeping the nose pointed upward. This minimizes struggling and the potential for injury. When the foal demonstrates vigorous attempts to stand, assistance to stand can be performed by grasping the base of the tail and giving firm support while the foal moves to a standing position. Neonates are a special population when it comes to pain management, as there is a fine balance between optimal pain relief and adverse drug effects (Maitra et al. 2014). As previously mentioned, untreated pain can have short‐ and long‐term negative consequences to a foal. Pain management can not only alleviate pain, it can lower anesthetic requirements during anesthesia and improve recovery afterward. In humans, opioid use in neonates undergoing surgery is associated with a blunted stress response and improved outcomes (Anand et al. 1987; Anand and Hickey 1992). The stress response initiates protein, fat, and carbohydrate catabolism and can cause metabolic acidosis, hypoglycemia, hyperglycemia, and electrolyte abnormalities that can increase morbidity and mortality (Goldman and Koren 2002). Opioids are the mainstay of analgesia in neonates; however, local and regional anesthetic techniques should be considered whenever possible. While many of the commonly used techniques in adult horses have not been described in neonates, anatomical landmarks are similar facilitating use. Care should be taken to reduce the dosage of local anesthetics utilized due to increased risk of toxicity in the neonate. Non‐steroidal anti‐inflammatory drugs (NSAIDs) are utilized in neonates; however, publications on their use in equine neonates is lacking and their use should be done with caution and close monitoring of renal and gastrointestinal function. Stabilization prior to administration of any sedative or anesthetic agents is critical for improving the odds of a successful outcome. Foals with many of the following conditions present as medical emergencies and not surgical emergencies. Therapies aimed at normalizing fluid volume, electrolyte, acid/base, and immunological status should be considered prior to surgical planning. Waiting for a period of time (in some cases, just a few hours) for correction of imbalances will result in a foal much more suitable for anesthesia and surgery. Sepsis is one of the most common medical conditions affecting neonatal foals (≤14 days of age) and is a leading cause of morbidity and mortality in this age group. The financial burden to owners is significant particularly when the prognosis for recovery is often uncertain (Weber et al. 2015; Sheats 2019; Furr and McKenzie 2020). The reported prevalence of sepsis in neonatal foals is approximately 25–60% of cases presenting for intensive care to tertiary veterinary hospitals (Weber et al. 2015; Wong and Wilkins 2015; Giguère et al. 2017; Wong et al. 2018; Furr and McKenzie 2020). Survival varies significantly (26–81%) with more recent reports demonstrating improvements in outcome (Gayle et al. 1998; Corley et al. 2005; Sanchez et al. 2008; Wong et al. 2018; Sheats 2019; Furr and McKenzie 2020). Sepsis in hospitalized humans, including pediatrics, also represents a leading cause of morbidity and mortality (Hall et al. 2013) and much of the criteria used in veterinary medicine for defining sepsis and the related condition systemic inflammatory response syndrome (SIRS) are derived from human medicine with modifications as needed for species‐specific differences in disease pathophysiology and diagnostic criteria. Sepsis is defined as life‐threatening organ dysfunction secondary to a dysregulated host response to infection (Singer et al. 2016). SIRS is defined as an overwhelming and dysregulated systemic inflammatory response to various infectious and noninfectious triggers, one of which may be sepsis (Bone et al. 1992). In uncontrolled disease, sepsis can progress to septic shock which includes circulatory, cellular, and metabolic derangements and ultimately multiorgan dysfunction (MODS) in the face of appropriate and aggressive treatment. These definitions and accompanying scoring systems for sepsis and SIRS have been developed in an attempt to provide clinically relevant criteria to improve early recognition in at‐risk human and equine neonatal patients (Brewer et al. 1988; Brewer and Koterba 1988; Bone et al. 1992; Corley et al. 2005). More recently, these definitions and scoring systems have been modified to account for physiologic and maturational differences among different age groups of foals, and the reader is referred to the associated references for further details (Wong and Wilkins 2015
13
Anesthetic Management of Foals
Introduction
Physiology of the Neonate
Cardiovascular Physiology
Respiratory Physiology
General Considerations for Anesthesia of Foals
Pre‐anesthetic Evaluation and Preparation
Parameter
Range
Physiologic parameters
Heart rate (beats/min)
80–100
Respiratory rate (breaths/min)
20–40
Temperature (°F/°C)
99–102/37–39
Parameter
Mean ± SD
Arterial blood gas (FiO2= 0.21)
pH
7.442 ± 0.015
PaO2 (mmHg)
78.4 ± 5.9
PaCO2 (mmHg)
38.5 ± 2.4
HCO3 (mEq/l)
27.6 ± 0.7
Lactate (mmol/l)
1.4 ± 0.2
Complete blood count
RBC (×106/μl)
9.26 ± 0.8
Hemoglobin (g/dl)
13.2 ± 1.2
Packed cell volume (%)
36.5 ± 3.1
Total protein (g/dl)
6.4 ± 0.6
Fibrinogen (mg/dl)
310 ± 90
Total white blood cells (WBC) (cells/dl)
9000 ± 2200
Neutrophils (cells/dl)
6500 ± 2000
Bands (cells/dl)
<50
Lymphocytes (cells/dl)
2200 ± 575
Monocytes (cells/dl)
300 ± 135
Eosinophils (cells/dl)
20 ± 4
Basophils (cells/dl)
17 ± 2
Serum biochemistry
Sodium (mEq/l)
140 ± 4.2
Chloride (mEq/l)
101 ± 4.0
Potassium (mEq/l)
4.5 ± 0.4
Calcium (mg/dl)
11.4 ± 0.8
Magnesium (mg/dl)
2.7 ± 0.15
Glucose (mg/dl)
150 ± 30
Blood urea nitrogen (mg/dl)
13.5 ± 5.5
Creatinine (mg/dl)
1.3 ± 0.3
Albumin (g/dl)
3.1 ± 0.5
Globulin (g/dl)
3.2 ± 0.5
Albumin : Globulin ratio
1.1 ± 0.4
Anesthetic/Analgesic Drugs
Sedatives and Tranquilizers
Drug
Dosage
Comments
Sedative drugs
Diazepam
0.05–0.2 mg/kg, IV
May cause excitement in healthy foals over two weeks of age. Propylene glycol vehicle may cause vascular irritation
Midazolam
0.05–0.2 mg/kg, IV
May cause excitement in healthy foals over two weeks of age
Xylazine
0.5–1.0 mg/kg, IV
Can have profound cardiovascular side effects. Avoid in sick foals and foals less than two weeks of age
Detomidine
0.005–0.015 mg/kg, IV
Can have profound cardiovascular side effects. Avoid in sick foals and foals less than two weeks of age
Dexmedetomidine
0.003–0.007 mg/kg, IV
0.001 mg/kg/h constant rate infusion (CRI), IV
Can have profound cardiovascular side effects. Avoid in sick foals and foals less than two weeks of age
CRI will reduce MAC of inhalant
Acepromazine
n/a
Not recommended for use in foals due to a long duration of effect, associated hypotension, and lack of reversal agent
Analgesic drugs
Butorphanol (opioid)
0.1–0.2 mg/kg, IV
Agonist–antagonist profile and may provide less analgesia than other opioids
Morphine (opioid)
0.1–0.5 mg/kg, IV
Higher dosages may cause excitement
Hydromorphone (opioid)
0.025–0.05 mg/kg, IV
Dosages described are for adult horses
Fentanyl (opioid)
0.002–0.004 mg/kg, IV
1–2 μg/kg/h, IV
Higher dosages may cause excitement and increased locomotor activity
Buprenorphine (opioid)
0.01–0.02 mg/kg, IV
Longer duration of action and not reversible
Flunixin meglumine (NSAID)
0.25–1.1 mg/kg, IV
Risk of GI ulceration
Meloxicam (NSAID)
0.2–0.6 mg/kg, IV
Risk of GI ulceration
Firocoxib (NSAID)
0.1 mg/kg
May cause less GI upset than other NSAIDs
Lidocaine (local anesthetic)
1–2 mg/kg total dose for local anesthesia techniques
1 mg/kg, IV followed by 25 μg/kg/min CRI
CRI provides MAC reduction
Mepivacaine (local anesthetic)
1–2 mg/kg total dose for local anesthetic techniques
Cardiotoxic and should not be administered IV
Bupivacaine (local anesthetic)
1–2 mg/kg total dose for local anesthetic techniques
Cardiotoxic and should not be administered IV
Anesthetic drugs
Ketamine
1.0–2.0 mg/kg, IV
Combine with a benzodiazepine for muscle relaxation
Propofol
2.0–4.0 mg/kg, IV
Administer to effect slowly
Dose and rate‐dependent hypotension and respiratory depression
Smooth recovery
Ketamine/propofol combination (“ketofol”)
0.5 mg/kg, IV ketamine
2.0 mg/kg, IV propofol
Administer ketamine first and then administer propofol to effect
Alfaxalone
2 mg/kg, IV
6 mg/kg/h CRI, IV
Muscle rigidity and rough recovery possible
Isoflurane
MAC = 1.31
Value is for adult horses and is likely lower for neonatal foals
Sevoflurane
MAC = 2.84
Value is for adult horses and is likely lower for neonatal foals
Adjunctive drugs
Atropine
0.02–0.04 mg/kg, IV
Glycopyrrolate
0.005–0.01 mg/kg, IV
Dobutamine
3–10 μg/kg/min, IV
Inotropic support
Norepinephrine
0.1–1.0 μg/kg/min, IV
Vasopressor support
Vasopressin
0.1–1.0 mU/kg/min, IV
May reduce splanchnic circulation
Atipamezole
0.05–0.1 mg/kg, intramuscular (IM)
Used for reversal of α2 agonist drugs
IM use only
Severe hypotension can result if administered IV
Flumazenil
0.01–0.05 mg/kg, IV
Reversal of benzodiazepines
Naloxone
0.005–0.05 mg/kg, IV
Reversal of opioids
Repeat dosage until desired effect achieved
Mannitol
0.25–1.0 g/kg, IV
May be useful in foals with PAS. Administer over 15–30 min through a filter
Regular insulin
0.25–0.5 U/kg, IV
Check serum glucose 20 min after administration and as needed afterward to monitor for hypoglycemia or hyperglycemia
Polymyxin B
2000–5000 U/kg, IV
Treatment for endotoxemia. Higher doses can be ototoxic and nephrotoxic
Dextrose
In IV fluids as 1–5% solution
Solutions greater than 5% can result in red blood cell damage/lysis
Opioid Analgesics
Induction Agents
Maintenance Agents
Monitoring and Support During Anesthesia
Cardiovascular Monitoring and Support
Ventilatory Monitoring and Support
Fluid and Electrolyte Monitoring and Therapy
Aim
Means
Maintain normal functional residual capacity
Optimize tidal volume
Maintain normocapnia (ETCO2 30–45 mmHg; PaCO2 35–45 mmHg)
Optimize oxygenation
Body Temperature Monitoring and Thermal Support
Recovery
Pain Management of the Foal
Anesthetic Considerations of Neonatal Foals with Specific Conditions/Diseases
Sepsis and Sepsis Associated Localized Infections
Stay updated, free articles. Join our Telegram channel

Full access? Get Clinical Tree
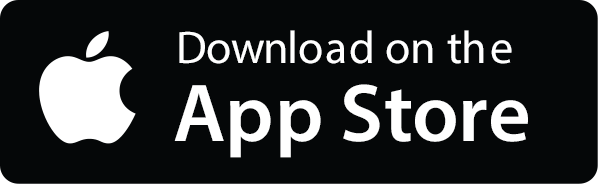
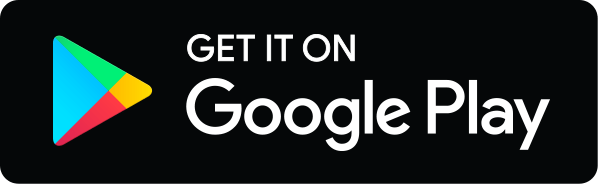