Erica McKenzie1 and Stuart Clark‐Price2 1 Department of Clinical Sciences, Carlson College of Veterinary Medicine, Oregon State University, 700 SW 30th Street, Corvallis, OR, 97331, USA 2 Department of Clinical Sciences, College of Veterinary Medicine, Auburn University, 1220 Wire Road, Auburn, AL, 36849, USA Anesthesia of adult horses and foals with pre‐existing muscular disorders is likely commonly practiced since clinical signs are often subtle, inhibiting recognition in advance of the procedure. The incidence of neuromuscular complications related to general anesthesia of horses has continued to decline as practices have evolved and greater understanding of equine muscular disorders is achieved (Bidwell et al. 2004, Bidwell et al. 2007; Senior et al. 2007; Dugdale and Taylor 2016; Valberg 2018). However, general anesthesia retains the potential to cause substantial primary muscle or peripheral nerve damage in horses, and may trigger clinical disease in a proportion of horses with pre‐existing muscular disorders. Minimizing the potential for harm relies on thorough pre‐anesthesia assessment of equine patients, attention to specific features relevant to the muscular and nervous systems during anesthesia, and careful post‐anesthesia monitoring and management of complications. Muscle is a large and metabolically active tissue in the horse, comprising between 40 and 55% of body mass, and receiving as much as 80% of cardiac output during intense exercise activity (Piercy and Rivero 2012; Poole and Erickson 2011). The vast majority of the horse’s muscle mass is located in the back and upper limbs, which facilitates speed and power (Kearns et al. 2002). This distribution makes horses considerably more vulnerable to neuromuscular injury during anesthesia, since some degree of compression of dependent muscle tissue and potentially peripheral nerves is likely to occur in typical recumbency positions. Total muscle mass and distribution of mass can vary considerably between different breeds, which also likely influences individual vulnerability to disease and clinical manifestation of disease. For example, Quarter Horses typically have considerably greater muscle mass in the hindquarter region than do comparable light breeds such as Arabians (Crook et al. 2008, 2010). Draft horses have proportionately less muscle than Thoroughbreds and Quarter horses; however, their much larger total body mass likely increases their risk of neuromuscular complications during general anesthesia (Gleed and Short 1980; Kearns et al. 2002; Rothenbuhler et al. 2006). Over 90% of muscle tissue is composed of myofibers, with the remainder comprised of specialized connective tissues, vessels, and nerves. Horses have a considerably higher proportion of fast twitch muscle fibers compared to other species with this fiber type representing more than 90% of muscle fiber populations in Thoroughbreds and Quarter horses, and ≥75% in other breeds (Piercy and Rivero 2012; Valberg 2014). Fast twitch fibers are located more superficially in the musculature, with type I, slow twitch fibers located deeper in the tissue to support the constant activity of postural maintenance. Muscle fibers are grouped into motor units, each of which consists of a single alpha motor neuron and the multiple fibers innervated by that neuron. A single motor neuron might service over a thousand individual fibers distributed among many different fascicles in the muscle; however, all fibers within that motor unit will be of a similar type. The number of motor units that are activated at one time is the key determinant of the force that the muscle will ultimately produce (Piercy and Rivero 2012; Valberg 2014). The majority of the internal environment of the muscle cell consists of organized bundles of overlapping contractile filaments that are arranged longitudinally into repeating units, referred to as sarcomeres. Individual sarcomeres are demarcated at each end by Z‐disks, which appear ultrastructurally as dense perpendicular striations. Emerging work indicates that Z‐disks are not inert and simple anchoring structures, but large and complicated arrays of different proteins with important roles in cell structure and signaling (Luther 2009). Between the Z‐disks, each sarcomere contains longitudinally oriented actin filaments anchored at each disk and extending from each disk toward the center of the cell, with their associated proteins including tropomyosin and troponins C, T, and I in contact along their length. Actin filaments overlap with centrally located and thicker bipolar myosin filaments, the globular heads of which are oriented toward each Z‐disk. Contraction occurs when calcium ions bind to troponin C which subsequently interacts with troponin I to resolve its inhibitory effect, resulting in exposure of myosin binding sites on actin. Cyclical binding of the many myosin heads to actin filaments brings the actin filaments in toward the center of the sarcomere from each end, resulting in shortening of the sarcomere (Cooper 2000; Lodish et al. 2000; Valberg 2014). Contraction of skeletal muscle commences with a nervous impulse, which must be converted to mechanical action (excitation‐contraction coupling). Depolarization of the motor neuron releases acetylcholine into the synaptic cleft, which binds reversibly to the motor end plate via nicotinic acetylcholine receptors, inducing depolarization of the sarcolemma. The electrical signal propagates internally into the myofiber along extensive invaginations of the sarcolemma, the T‐tubules. Depolarization of the tubules results in conformational change in their membrane‐associated voltage sensors, the dihydropyridine receptors. This subsequently invokes a change in conformation of the closely apposed calcium release receptors, also known as ryanodine receptors, which occur as the RYR1 isoform in skeletal muscle. Rapid release of a large number of calcium ions into the sarcoplasm massively increases the calcium concentration around the myofilaments, encouraging binding of calcium to troponin C and subsequent events promoting contraction. Relaxation is achieved via active transport of calcium ions back into the SR via the activity of the SR calcium ATPase enzyme. (Cooper 2000; Lodish et al. 2000; Valberg 2014). Many of the anesthetic, analgesic, and adjunct medications used during anesthesia of horses have direct or indirect effects on skeletal muscle. There is relatively limited information regarding the effects of these drugs on contractile mechanics or myocyte metabolism in horses. Most studies have focused on responses to halothane, and have usually examined in vitro systems (Hildebrand et al. 1990; Beech et al. 1993; Lentz et al. 1999a, b; Raisis et al. 2000; Edner et al. 2005). Investigations have commonly focused on the impact of drugs on perfusion of muscle tissue because of the association of hypoperfusion with muscle damage in anesthetized horses, attributed to factors including large muscle mass and hypotension creating particular risk to dependent musculature (Trim and Mason 1973; Grandy et al. 1987; Serteyn et al. 1988; Lindsay et al. 1989; Raisis et al. 2000; Raisis 2005). The effects of individual drugs used in premedication, induction, maintenance, and as adjunct therapies on the muscular system are described below. The α2‐adrenergic receptor agonist agents, encompassing xylazine, detomidine, romifidine, medetomidine, and dexmedetomidine, are frequently utilized in standing sedation of horses for examination and diagnostic purposes, for analgesia, and as premedications prior to general anesthesia. In addition to sedative and analgesic effects, these drugs can provide muscle relaxation via centrally mediated effects. Muscle relaxation may arise from depressant effects on the locus coeruleus neurons in the brain resulting in disfacilitation of spinal reflexes (Palmeri and Wiesendanger 1990). The cardiovascular side effects of α2‐adrenergic receptor agonists are well described and include vasoconstriction followed by vasodilation, bradycardia, and decreased cardiac output (Bettschart‐Wolfensberger 2015). In combination, these effects can reduce blood flow to skeletal muscle in standing and anesthetized horses (Hennig et al. 1995; Raisis 2005). This can promote anaerobic metabolism within affected muscle tissue during anesthesia, and may increase vulnerability of skeletal muscle to continued insult after anesthetic recovery (Edner et al. 2002). Phenothiazine use in horses is largely limited to acepromazine. Acepromazine provides minor sedation with some muscle relaxation and is commonly used as a sedative prior to equine anesthesia and during anesthetic recovery. However, as a potent alpha antagonist, acepromazine can provoke profound vasodilation and decrease blood pressure and perfusion. Conversely, at moderate dosages, acepromazine may improve arterial oxygen tension and systemic perfusion, particularly in anesthetic plans that utilize α2‐adrenergic receptor agonists in healthy horses, and may therefore preserve or promote muscle perfusion (Marntell et al. 2005). Interestingly, a large‐scale study of anesthetized horses reported a reduced risk of mortality when acepromazine was utilized as a sole premedication agent. However, there is no evidence that this beneficial effect related to preservation of perfusion in skeletal muscle, and other mechanisms are more likely responsible (Johnston et al. 2002; Auckburally and Flaherty 2009a). Nonetheless, acepromazine can cause a substantial decrement in blood pressure, which should be taken into account when other exacerbating factors for hypotension are expected. There is little available data on the effect of opioid medications on intrinsic skeletal muscle function in horses. Butorphanol, an agonist–antagonist opioid had no effect on ex‐vivo equine esophageal skeletal muscle response to electrical stimulation and was thought to not interfere with in vivo contractile mechanisms (Wooldridge et al. 2002). Benzodiazepines likely have little to no direct effect on skeletal muscle perfusion or metabolism. However, they have potent muscle relaxant properties that are centrally mediated through enhancing the inhibitory effects of gamma‐aminobutyric acid (GABA) at the GABAA receptor, making them beneficial in reducing spasticity in combinations with ketamine (Olkkola and Ahonen 2008). Diazepam and midazolam represent the most commonly utilized benzodiazepine agents in horses. Administration at high doses can promote weakness, fasciculations, ataxia, and recumbency, even in the absence of sedation, and the potential for synergistic effects with other relaxant agents should also be considered (Muir et al. 1982; Hubbell et al. 2013). Guaifenesin is a centrally acting muscle relaxant used as an adjunct agent during equine anesthesia for sedation and relaxant properties. However, excessive or repeated dosing can lead to toxic effects manifesting as extensor muscle spasm or rigidity (Funk 1973). Although not completely understood, the central effects of guaifenesin appear to be mediated through inhibition of the N‐methyl‐D‐aspartate receptor (Keshavarz et al. 2013). The use of anticholinergic agents in horses under anesthesia is controversial, mainly because of their potential to induce gastrointestinal stasis and subsequent colic (Teixeira Neto et al. 2004). The authors could find no reports on the direct effect of atropine or glycopyrrolate on skeletal muscle in horses; however, glycopyrrolate improved cardiac output, arterial blood pressure, and tissue oxygen delivery during halothane anesthesia and xylazine infusion in horses (Teixeira Neto et al. 2004), suggesting implications for muscle resilience under anesthesia. Dobutamine is currently considered the sympathomimetic agent of choice for the treatment of hypotension during anesthesia in horses. Its primary action is mediated via agonist activity at the beta‐1 receptor, increasing cardiac contractility and cardiac output. Dobutamine increases peripheral oxygen delivery and increased femoral artery blood flow when mean arterial pressure approached 80 mmHg in horses (Schier et al. 2016). In contrast, dopamine, norepinephrine, and phenylephrine have more variable effects on cardiac output, vascular resistance, and peripheral perfusion (Dancker et al. 2018). Dobutamine therefore appears to be the agent of choice for improvement of cardiac output in anesthetized horses that are hypotensive. However, it should be noted that increased femoral artery flow has not been definitively proven to translate to improved muscle tissue perfusion at the microvascular level in anesthetized horses (Raisis et al. 2000). The dissociative anesthetic ketamine is the most commonly used induction agent utilized in equine anesthesia, and disrupts communication between the limbic and thalamocortical systems (Reich and Silvay 1989). The result of this action is to induce a cataleptic‐like state that manifests as muscle spasticity, rigidity, and even unconscious spontaneous movement (Berry 2015). For this reason, medications with muscle relaxant properties are frequently co‐administered with ketamine. These include α2‐adrenergic receptor agonists, benzodi‐azepines, and guaifenesin (Nowrouzian et al. 1981; Fisher 1984; Matthews et al. 1991; Vien and Chhabra 2017). In regard to muscular perfusion, ketamine is generally accepted to maintain or increase cardiovascular function in horses, and in a rabbit model of hemorrhage, ketamine maintained skeletal muscle capillary perfusion more effectively than pentobarbital or propofol, suggesting possible benefit in hemorrhaging patients (Gustafsson et al. 1995; Gozalo‐Marcilla et al. 2014). Propofol is being progressively utilized in equine anesthesia. It is not useful as a sole induction agent in adult horses; however, when combined with other agents such as ketamine, it can replace benzodiazepines for sedation and muscle relaxation (Wagner et al. 2002; Posner et al. 2013). If used as a sole maintenance agent in horses, propofol infusion decreases cardiac contractility and systemic vascular resistance in a dose‐dependent manner (Nolan and Hall 1985; Oku et al. 2006) which may impair skeletal muscle perfusion during anesthesia. However, when infused in combination with ketamine it creates minimal cardiovascular depression and appears to have limited impact on skeletal muscle perfusion in horses (Mama et al. 1996; Edner et al. 2002; Umar et al. 2015). In humans, prolonged propofol infusion has resulted in “propofol infusion syndrome” in a small number of patients, characterized by metabolic acidosis, cardiac electrical abnormalities, cardiac and/or renal failure, hyperkalemia, hepatomegaly, hyperlipidemia, and rhabdomyolysis (Krajčová et al. 2018). This is thought to occur through an adverse effect on mitochondrial function of skeletal muscle and is considered a propofol‐related bioenergetics failure. Although this condition has not been described in veterinary patients, long‐term propofol infusion in animals with skeletal muscle dysfunction should be performed with caution. Furthermore, propofol may enhance the potency of nondepolarizing neuromuscular blocking agents such as rocuronium (Stäuble et al. 2015). Alfaxalone is a neuroactive steroid anesthetic, and its action is mediated via the GABAA receptor. Although the pharmacokinetics of alfaxalone have been studied in horses, and it has been utilized in intravenous combinations for brief surgical procedures in this species, there is minimal information available regarding its effects on equine musculature (Goodwin et al. 2011; Goodwin et al. 2013; Aoki et al. 2017). However, there is a higher probability of muscle tremors at induction and more prolonged recovery time when alfaxalone is utilized for induction of horses compared to ketamine, which could have implications for muscular health and post‐anesthesia serum muscle enzyme activity (Keates et al. 2012; Wakuno et al. 2017). The volatile anesthetic agents currently used most commonly in horses are isoflurane and sevoflurane. Isoflurane has largely replaced halothane as the standard inhalant used for general anesthesia in horses because of reduced cardiovascular depression. Specifically, horses anesthetized with isoflurane have higher heart rate, cardiac output and cardiac index, greater oxygen delivery, aortic blood flow velocity, and femoral arterial and venous blood flow, and lower systemic vascular resistance compared to horses anesthetized with halothane (Steffey and Howland 1980; Raisis et al. 2000; Durongphongtorn et al. 2006). Sevoflurane appears to be very similar to isoflurane in regard to cardiopulmonary function (Steffey et al. 2005). Anesthesia with isoflurane also improves intramuscular blood flow compared to halothane (Lee et al. 1998). However, muscle blood flow is still decreased compared to that in non‐anesthetized horses, and decreases as the minimum alveolar concentration (MAC) of isoflurane is increased (Goetz et al. 1989). Furthermore, there is no evidence in a large‐scale study that the risk of equine anesthesia associated myopathy is less with isoflurane compared with halothane (Johnston et al. 2004). However, reducing cardiovascular depression via inhalant agent selection, and preventing hypoxemia and maintaining mean blood pressure above 70 mmHg might prevent or reduce muscle damage during anesthesia and into the recovery period (Grandy et al. 1987; Duke et al. 2006; Portier et al. 2009). Equine anesthesia associated myopathy (EAAM) is the most common muscular complication of general anesthesia in horses. Previously implicated in more than 7% of equine peri‐anesthetic deaths in a large study in the 1990s (Johnston et al. 2002), the transition to newer inhalant agents combined with greater recognition and control of potential precipitating factors appears to have resulted in a substantial decline in clinical cases (Dugdale and Taylor 2016). More recent studies report prevalences of 0.76% and 1.7% for clinical disease (Johnston et al. 2004; Franci et al. 2006), though higher prevalences are reported in special procedures including magnetic resonance imaging (2.3%) and transvenous electrical cardioversion (9.2%) for undefined reasons (Franci et al. 2006; Bellei et al. 2007). It is likely that subclinical muscle damage is also a common occurrence in anesthetized horses. In a study by one of the authors of a Quarter horse dominant group of 116 horses undergoing general anesthesia at four different institutions between 2008 and 2010, 6% of studied horses had serum creatine kinase (CK) activities exceeding 5000 U/l within 24 hours after anesthesia without clinical signs of EAAM (McKenzie, unpublished data). A total of 50% of horses had post‐anesthesia serum CK activities exceeding 1000 U/l, a value which was used as a benchmark for EAAM in a commonly cited study (Duke et al. 2006). Muscle and/or nerve damage is also suggested to increase the probability of traumatic fractures in horses in recovery, which is regarded as the greatest cause of recovery mortality (Friend 1981; Johnston et al. 2004; Dugdale and Taylor 2016). Predisposing factors that contribute to neuromuscular damage under or after anesthesia include inadequate padding of vulnerable body regions, long duration of anesthesia and/or anesthetic recovery, inappropriate positioning of limbs, hypotension, and potentially hypoxemia (Dugdale and Taylor 2016; Ayala et al. 2009; Young 2005). Large body mass is likely also a factor, and 6% of draft horses undergoing upper airway surgery were reported to develop neuromuscular complications related to anesthesia (Kraus et al. 2003). A history of fitness, recent exertion, or exertional rhabdomyolysis (ER) is also linked to anesthesia‐related muscle damage in horses (Hildebrand et al. 1990; Klein 1978; Aleman et al. 2009, Auckburally and Flaherty 2009a, McKenzie, unpublished data). In a large study of over 8000 horses, procedures lasting greater than 90 minutes were associated with a tenfold increase in risk of myopathy, and lateral recumbency was also associated with greatly increased risk (Johnston et al. 2004). An association of EAAM with hypotension has been made in several studies, leading to the recommendation for mean arterial blood pressure (MAP) to be maintained at or above 70 mmHg, which might not prevent myopathy but which might decrease clinical severity (Grandy et al. 1987; Duke et al. 2006; Young 2005). Though commonly accepted, the validity of this specific recommendation is unclear, since it was based on a small number of horses in one institution, and was not verified by large‐scale assessment across multiple institutions (Johnston et al. 2004). Furthermore, severe EAAM has been reported in horses with optimal blood pressure status, suggesting that maintenance of blood pressure cannot reliably prevent EAAM, particularly if other precipitating factors are present (Short and White 1978; Johnston et al. 2004; Ayala et al. 2009). Clinical manifestations of EAAM most frequently involve dependent muscle groups including the quadriceps, triceps, and masseter muscles in laterally positioned animals or the gluteal and longissimus dorsi muscles in dorsally positioned animals (Clark‐Price et al. 2012). The adductor muscles can be compromised in dorsally positioned horses in spite of their non‐dependent position, possibly due to constriction of the medial circumflex femoral artery (Dodman et al. 1988). Anecdotal reports of horses developing EAAM in other dependent and non‐dependent muscle groups emphasize the limited understanding of the pathophysiology of this disorder. Clinical signs consistent with EAAM are most likely to become evident during recovery or in the hours afterward. Whether single or multiple muscles are involved, affected horses display combinations of prolonged recumbency, violent or difficult recovery with repeated attempts to rise, weakness or reduced weight bearing on one or more limbs, fasciculations, distress, myoglobinuria, and sweating, with pain, swelling, and firmness of the affected muscle groups (Friend 1981; Dodman et al. 1988; Ayala et al. 2009; Clark‐Price et al. 2012). A dropped position of the elbow can reflect triceps muscle damage with or without accompanying radial nerve paralysis (Figure 8.1), and crouching or weakness of the hind limbs can reflect damage to large muscles and/or nerves in these regions. Neuropathy related to anesthesia has been suggested to be minimally painful despite obvious dysfunction of the affected limb, with minimally deranged serum CK values (Coumbe 2005; Auckburally and Flaherty 2009a). However, horses may become frantic from an inability to utilize a limb and may manifest behavioral signs that can be confused with pain (e.g. tachycardia, sweating, frenetic movement). Mixed neuropathy and myopathy syndromes are possible and the degree to which dysfunction relates to each tissue may not be clear, particularly in the early stages of disease (Young 2005). Figure 8.1 A horse after recovering from anesthesia with inability to put weight on the left forelimb demonstrating a “dropped elbow.” Initial clinical signs of EAAM may be subtle and not recognized until hours later when affected horses might display lameness, visible muscle swelling, or biochemical indicators of muscle injury. In rare cases, biochemical and clinical evidence of muscle damage has appeared several days after anesthesia, though the reasons for such apparently delayed onset are unknown (Grint et al. 2007). Serum CK activity in horses with EAAM is often only mildly elevated immediately after anesthesia (~1000–5000 U/l), but frequently increases over the next 24–48 hours, and elevations can be profound (Waldron‐Mease and Rosenberg 1979; Duke et al. 2006; Ayala et al. 2009, McKenzie et al. 2015). Therefore, serial monitoring of clinicopathologic variables, particularly muscle enzyme activities and serum electrolyte concentrations is recommended in any horse considered at risk of or potentially affected with EAAM. Some horses can have significant elevations in serum CK with relatively limited clinical signs if there is diffuse damage to a large amount of muscle mass. Conversely, significant clinical signs can occur with relatively limited increases in serum CK if a small area or single muscle group is severely damaged, such as the triceps (Ayala et al. 2009; McKenzie et al. 2015). As a result, serum CK should always be interpreted in conjunction with thorough physical examination, and should be serially monitored to determine need for systemic interventions including intravenous fluids, and to monitor progression and resolution of rhabdomyolysis. Given the dynamics of this enzyme, evaluation at 12‐hour intervals is ideal in the early stages of disease. Serum CK is often reported as a cut‐off value, typically between 2000 and 20 000 U/l, which can obscure the true magnitude of increase (Friend 1981; Overmann et al. 2010). If cut‐off values are exceeded and the potential for significant rhabdomyolysis exists, manual dilution of samples should be performed to achieve an approximate maximum value (Overmann et al. 2010). Serum CK activity can exceed 1 million U/l in very severe rhabdomyolysis; remarkably, this is not always correlated with a fatal outcome (Collins et al. 1998). Furthermore, horses with massively elevated serum CK can also display spuriously high serum total CO2 concentrations and negative anion gap if these values are determined on a chemistry analyzer versus a blood gas analyzer. This results from interference of enzymatic reactions in the analyzer generated by release of pyruvate and lactate dehydrogenase from injured muscle cells (Overmann et al. 2010). Serum aspartate aminotransferase (AST) is less labile than CK and is also useful in determining immensity of damage and progress over time, particularly if CK has normalized. Horses with severe muscle damage will have AST values exceeding several thousand U/l, with a maximum ceiling of approximately 45 000 U/l; values above 20 000 U/l are uncommon and indicate profound generalized rhabdomyolysis (Friend 1981; Collins et al. 1998). Diagnostic ultrasound can be performed on visibly affected muscle groups to evaluate local swelling and edema, and to differentiate probable EAAM from a traumatic muscle tear sustained during anesthetic recovery. Ultrasound is particularly useful if the contralateral muscle appears normal and can provide an appropriate comparison (Walmsley et al. 2010). Horses with suspected or confirmed EAAM should be treated promptly and aggressively, to limit pain and muscle swelling, to correct acid–base and electrolyte abnormalities, and to reduce the deleterious effects of myoglobinuria. The disorder is often progressive, and horses that are initially weight bearing and standing can become non‐weight bearing or even recumbent as muscle swelling and damage progresses over the next 24–28 hours, hence early treatment and close observation over time is warranted. Serial monitoring of biochemical variables including blood urea nitrogen (BUN) and creatinine, CK and AST, serum electrolytes and acid–base status is indicated. Horses with visible myoglobinuria should receive intravenous or nasogastric fluids at least until urine color returns to normal and any perfusion and electrolyte anomalies are resolved. Blood and urine pH should be assessed, since the production of acid urine can greatly enhance the nephrotoxicity of myoglobin. Horses with disturbed acid–base status (blood pH < 7.2, urine pH < 7.0) and myoglobinuria might benefit from inclusion of sodium bicarbonate in intravenous fluids until normal values are achieved (Waldron‐Mease 1978). Myoglobinuria is usually a transient phenomenon since this product is cleared rapidly from the serum, so careful attention should be paid to urinations for resolution over time. Profound muscle swelling can damage peripheral nerves and exacerbate discomfort and muscle necrosis, and surgical fasciotomy might be required in severe cases to relieve elevated pressure in intra‐ and inter‐fascial compartments (Figure 8.2). Figure 8.2 A horse with equine anesthesia associated myopathy with severe swelling of the epaxial and gluteal musculature. Surgical fasciotomy was performed to relieve elevated intra‐ and inter‐fascial compartment pressure. Pain management can be very challenging in horses with severe focal myopathy causing non‐weight‐bearing lameness, and multi‐modal analgesia is indicated. Non‐steroidal anti‐inflammatory agents are valuable, but should be applied with caution if patients are azotemic, visibly myoglobinuric, receiving concurrent nephrotoxic drugs, or have other contraindications to their use (Cook and Blikslager 2015). Acepromazine has also been utilized empirically in EAAM for its sedative, relaxant, and vasodilatory effects and can be given by intermittent administration orally or parenterally. Opioid medications including morphine and butorphanol can be useful adjunct analgesics in horses with significant discomfort. Intermittent administration may be adequate; however, more severe pain may respond more favorably to constant rate infusions of these drugs. Horses should be monitored for potential adverse effects including excitement, compulsive walking, indicators of reduced gastrointestinal motility, and urinary retention, particularly if receiving high intermittent doses or constant rate infusions of opioids (Clutton 2010; Gozalo‐Marcilla et al. 2014; Sanchez and Robertson 2014). Constant rate infusions of other analgesic drugs including lidocaine and ketamine can be beneficial in horses with severe discomfort. Horses with severe pain creating danger to themselves and their handlers may require administration of a combination of drugs by bolus and constant rate infusion, including alpha‐2 agonists, to permit additional measures such as placement of epidural medication to reduce acute or ongoing distress. Epidural injection or epidural catheter placement with intermittent or constant infusions of analgesic drugs should be strongly considered in horses with profound pain inhibiting weight bearing. This is particularly important if initial pain control measures fail to achieve reasonable comfort within 12 hours, to reduce the risk of supporting limb laminitis. The combination of morphine with xylazine or detomidine can provide potent relief when one or more limbs are partially or totally non‐weight bearing (Natalini 2010). The volume of injection can be increased in horses with forelimb involvement to promote cranial analgesia. As pain improves, analgesic drugs can be reduced or removed in a serial fashion over time while monitoring the patient’s progress. The process should commence by removing agents most likely to produce side effects and/or that are the most expensive or inconvenient to administer. Ideally, only a single medication is manipulated at any time to reduce risk of compromising clinical stability and to accurately assess the effect of specific changes. Figure 8.3 A horse with equine anesthesia associated myopathy in a sling to assist the horse with remaining in a standing position. Horses should be encouraged to remain standing if possible, and may require a lift or sling to assist them to rise (Figure 8.3). If they are recumbent and slinging is not tolerated or feasible, then attempts should be made to rotate lateral recumbency frequently or to maintain them in sternal position in a deeply bedded stall. Manual assistance to stand several times a day can help relieve compression of musculature, but must be performed with attention to the risk of additional injury. Limbs should be wrapped and shoes removed or wrapped to help prevent additional trauma. Cryotherapy consisting of ice packs or cold‐water hydrotherapy over localized areas of muscle damage may assist with pain management and recovery (Figure 8.4). Horses with severe focal or diffuse rhabdomyolysis may benefit from the administration of dantrolene sodium (4–6 mg/kg, every 12 hours per os to a recently fed horse) (McKenzie et al. 2010). This drug prevents additional muscle necrosis and potentially speeds recovery and reduces muscular pain. Dosing should begin as soon as recognition of a muscular complication is made. Administration of dantrolene via nasogastric tube can be performed as soon as a swallowing reflex is present in the recovering patient if feasible in a manner that prevents risk to the operator (Waldron‐Mease and Rosenberg 1979). Figure 8.4 Treatment of a horse with equine anesthesia associated myopathy with cryotherapy using ice packs placed over the affected muscles. Figure 8.5 A horse in lateral recumbency during anesthesia. The down forelimb is manually pulled forward to minimize pressure on the dependent muscles and nerves. Prevention of EAAM should be approached in a multi‐faceted manner due to the range of contributing factors. Tactics include careful limb positioning to protect dependent musculature, minimizing duration of anesthesia, and maintaining mean arterial pressure at values above 70 mmHg, which may not prevent but can potentially reduce severity (Duke et al. 2006). In laterally recumbent horses, the dependent front limbs should be pulled forward to minimize pressure from bones on large nerve bundles and non‐dependent limbs should be placed in a neutral position and supported with padding or stirrups (Figures 8.5 and 8.6). Placing horses on an adequately padded surface is critical, and since the duration of procedures can be variable and challenging to predict, padding should be considered even for procedures expected to be transient in nature. Padding should be thick and soft enough to allow for a diffuse area of contact with the horse’s body to minimize pressure localization on small areas. If procedures are to extend significantly beyond their expected duration, then re‐assessment of patient positioning and padding should be made to determine if there are beneficial alterations that could be feasibly achieved to avoid complications. Specialized recovery systems including air mattresses, pools, and rope systems may reduce the risk of additional muscular trauma related to prolonged or violent recovery. Figure 8.6 A horse in lateral recumbency during anesthesia. Padded stirrups are used to maintain the limbs in a neutral position to the trunk to minimize strain and pressure on the musculature. Prevention of EAAM can potentially also be achieved by premedication with dantrolene sodium in horses considered at high risk. Studies demonstrate substantially lower serum CK activities in horses anesthetized after dantrolene administration than after sham treatment, even under hypotensive conditions (McKenzie and Mosley 2010; McKenzie et al. 2015). A dose of 4–6 mg/kg can be provided orally or by nasogastric tube one to two hours prior to anesthesia (McKenzie et al. 2015). It should be noted that dantrolene absorption is minimal in horses fasted for 12 hours, and optimal in horses fed in the 4 hours preceding administration, which can interfere with standard pre‐anesthesia fasting protocols (McKenzie et al. 2010). Other potential disadvantages of dantrolene administration include a negative effect on cardiac output, and potential development of hyperkalemia in anesthetized horses approximately two hours after administration of the drug, and one hour after commencement of anesthesia (McKenzie et al. 2015). These findings might relate to the dose used in the reporting study (6 mg/kg) and protective effects are possibly achieved at lower doses. However, substantial inter‐individual variability in plasma concentrations after treatment makes determination of an appropriate and reliable oral dose challenging. Currently, premedication with dantrolene should be considered only for horses considered at high risk of EAAM and avoided in horses susceptible to hyperkalemic periodic paralysis (). Horses that receive dantrolene prior to anesthesia should have serial monitoring of blood potassium concentrations and cardiac rhythm performed throughout anesthesia (McKenzie et al. 2015). HYPP is a well‐described hereditary muscular disorder that predominantly affects Quarter horses and related breeds including Paints, Palominos, Appaloosas, and the Pony of the Americas breed (Naylor 1997). The disease is associated with a point mutation in the SCN4A gene encoding the alpha subunit of the skeletal muscle sodium channel, and is heritable in a codominant autosomal fashion (Naylor et al. 1999; Mickelson and Valberg 2015). This results in a higher resting membrane potential in muscle fibers containing mutant sodium channels, increasing their susceptibility to depolarization, particularly in the presence of high potassium concentrations (Pickar et al. 1991). Clinically, this is most often reflected by muscle fasciculations with subsequent weakness as a result of depolarization block. Abnormal muscular activity during clinical episodes of disease promotes efflux of potassium into the extracellular environment in many, but not all affected horses, evidenced by an increase in serum potassium concentration. The genetic mutation associated with HYPP has been previously estimated to occur with a prevalence of 4.4% in the general Quarter horse population, and remarkably, exceeded 55% in a genetic survey of elite Halter horses (Tryon et al. 2009). This is likely the result of positive selection pressure related to enhanced muscle mass in affected animals (Naylor 1994; Bowling et al. 1996; Tryon et al. 2009; Mickelson and Valberg 2015). Since 2007, foals that test homozygous for the relevant mutation cannot be registered with the American Quarter Horse Association; however, there is no evidence that this has reduced the prevalence of the disorder, perhaps unsurprising since most horses that carry the defect are heterozygotes (Mickelson and Valberg 2015). Horses that carry the mutation can be asymptomatic, or may display episodic weakness, third eyelid protrusion, facial myotonia, sweating and muscle fasciculations. Homozygous animals may also display dysphagia as foals, or respiratory compromise with stridor, respiratory distress, and hypercapnia due to laryngeal and pharyngeal dysfunction (Meyer et al. 1999). Hyperkalemia is a common corollary of abnormal muscle activity and can result in cardiac arrhythmias. Horses often display clinical episodes of HYPP from two to three years of age onward, and horses under or recovering from anesthesia are at particular risk, even in their first year of life (Cornick et al. 1994; Bailey et al. 1996; Baetge 2007). It is unclear why anesthesia might enhance susceptibility to clinical episodes of HYPP. A clinical HYPP event during anesthesia is possible even in horses that have previously been anesthetized without complication (Cornick et al. 1994; Pang et al. 2011). Clinical signs may be mild and nonspecific, reflected simply by prolonged recovery and ineffective attempts to stand. Severe signs include fasciculations, tachycardia, rigidity, sweating, hypercapnia, hypertension, and cardiac arrhythmias (Baetge 2007). Hyperkalemia can develop as a progressive phenomenon over time, hence serial sampling during anesthesia is recommended in any patient with known or possible HYPP (Baetge 2007). Electrocardiogram analysis can reveal development of abnormalities consistent with hyperkalemia, indicating the need for rapid pharmacologic intervention. Changes to the electrocardiogram (ECG) observed in hyperkalemic horses include widening of the P wave with decreased amplitude, and eventual obliteration of P wave activity. The P‐R interval and T wave amplitude might both increase (Carpenter and Evans 2005). The QRS interval may become prolonged and begin merging with the T wave, with irregular ventricular rate preceding ventricular fibrillation. It should be noted that there is poor correlation between heart rate and plasma potassium concentration, and absence of ECG changes does not rule out the potential for severe hyperkalemia to be present. Horses can display severe cardiac rhythm anomalies once plasma potassium reaches or exceeds 5.5 mEq/l. Treatment to address hyperkalemia is prudent if plasma potassium reaches or exceeds this level or displays a progressive increase, regardless of the underlying provoking condition (Glazier et al. 1982; Meyer et al. 1999; McKenzie et al. 2015; Langdon Fielding 2015). Recognition of an HYPP episode during anesthesia should prompt emergency measures to address hypercapnia and hyperkalemia (Table 8.1). Affected horses should be ventilated appropriately to maintain acceptable arterial partial pressure of CO2 as an aid to increase blood pH. Infusion of crystalloid solutions with added potassium, or whole blood should be ceased and replaced with crystalloid fluids (lactated Ringer’s solution [LRS], Plasmalyte) with no external additives. These formulations contain minimal potassium, exerting a dilutional effect on plasma potassium concentrations, and also provide a pH buffering effect which may help further reduce plasma potassium through cellular ion exchange (Dépret et al. 2019). The use of normal saline has recently been questioned as this solution is acidifying and can increase plasma potassium concentration and exacerbate acid–base aberrations (Khajavi et al. 2008; Cunha et al. 2010; Li et al. 2016; González‐Castro et al. 2018; Dépret et al. 2019). Hyperkalemia can also be addressed via the administration of dextrose and insulin, and calcium gluconate to enhance intracellular movement of potassium and reduce the risk of cardiac arrhythmias (Langdon Fielding 2015; Dépret et al. 2019). Sodium bicarbonate also represents a traditional treatment for hyperkalemia; however, hypertonic saline is similarly effective in decreasing plasma potassium concentration and preventing cardiac arrhythmia (Trefz et al. 2017). This likely reflects vascular expansion, diuresis, and hypernatremia‐mediated intracellular movement of potassium. Additionally, hypertonic saline increases the velocity of the rising action potential, which is depressed during hyperkalemia, and therefore may act as a membrane stabilizer similarly to calcium (Dépret et al. 2019). Administration of crystalloid fluids after administration of hypertonic saline is indicated to prevent detrimental cellular dehydration and chloride‐induced acidemia.
8
Anesthetic Management for Muscular Conditions
Introduction
Muscle Physiology
Effects of Commonly Used Anesthetic/Analgesic Medications on Equine Skeletal Muscle
Premedication and Adjunctive Agents
α2‐Adrenergic Receptor Agonists
Phenothiazines
Opioids
Benzodiazepines
Guaifenesin (Glyceryl Guaiacolate)
Anticholinergics
Dobutamine
Induction Agents
Dissociatives
Propofol
Alfaxalone
Maintenance Agents
Volatile Anesthetics
Specific Neuromuscular Disorders of Horses
Equine Anesthesia Associated Myopathy
Hyperkalemic Periodic Paralysis (HYPP)
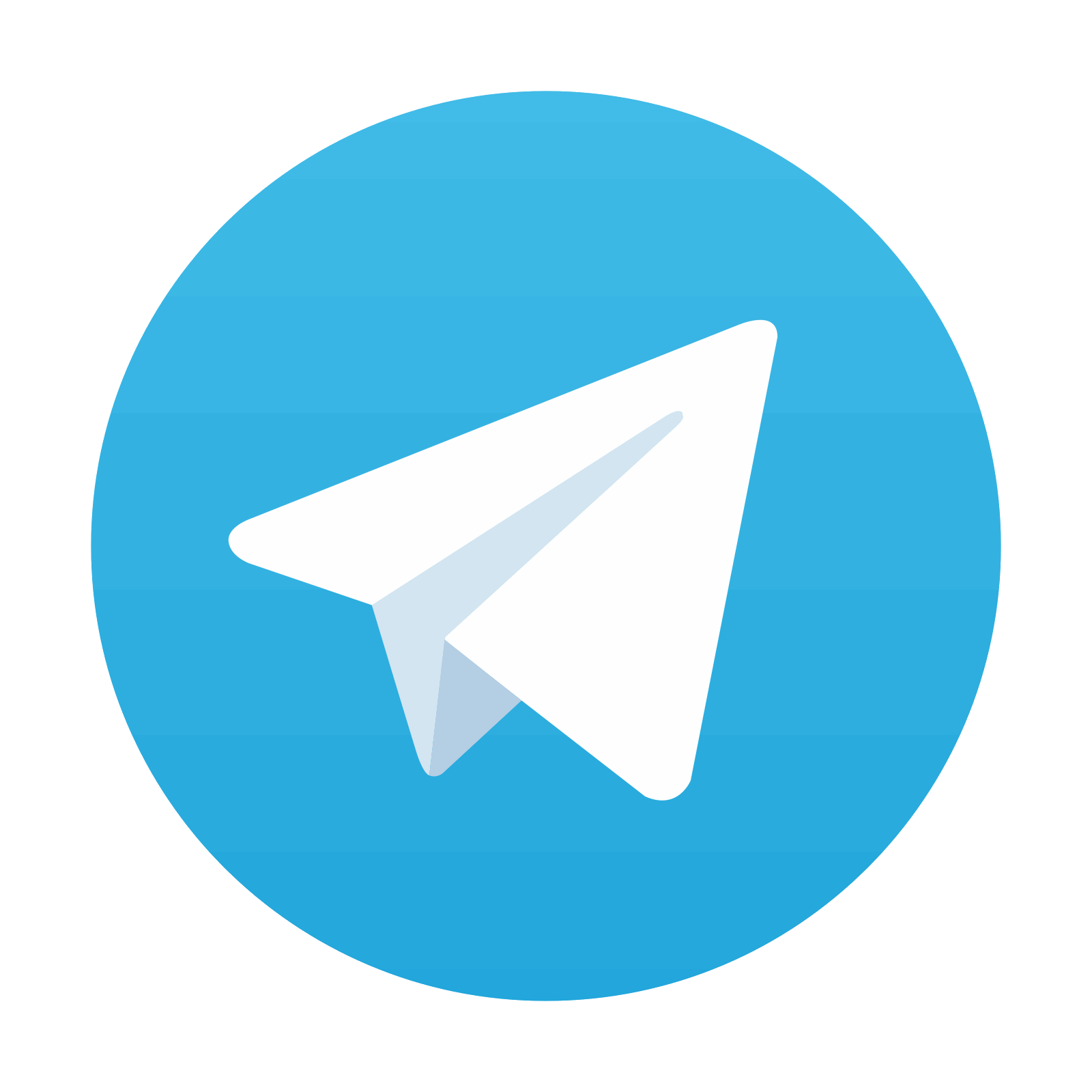
Stay updated, free articles. Join our Telegram channel

Full access? Get Clinical Tree
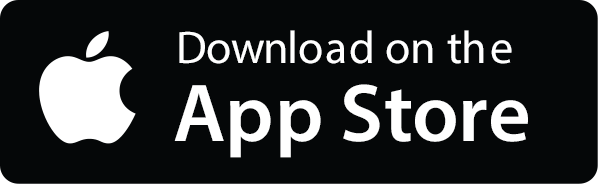
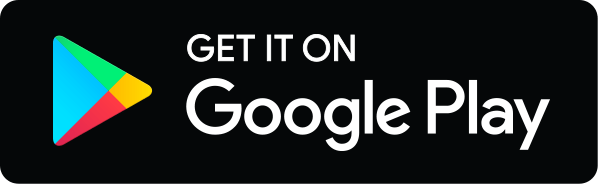