Alexander Valverde1, Valerie Moorman2, and Kirsty Gallacher3 1 Department of Clinical Studies, Ontario Veterinary College, University of Guelph, 45 College Ave W, Guelph, Ontario, N1G 1R8, Canada 2 Department of Large Animal Medicine, College of Veterinary Medicine, University of Georgia, 2200 College Station Road, Athens, GA, 30602, USA 3 School of Animal and Veterinary Sciences, The University of Adelaide, 1454 Mudla Wirra Road, Roseworthy, South Australia, 5371, Australia Several references are available and provide a comprehensive review of the anatomy of the male and female reproductive and urinary tracts in the horse. A brief summary is provided in the following text with focus on unique features in the horse that may be of benefit for clinical understanding. The female reproductive tract comprises of bilateral ovaries and oviducts, and the uterus, cervix, vagina, and external genitalia. The equine ovaries are relatively large compared to other species and frequently described as “kidney‐bean” shaped, but shape and size can be quite variable depending upon follicular content (range between 4 × 2 cm and 8 × 5 cm). The ovaries and uterine tubes are the most cranial structures of the reproductive tract and may be found as far cranially as the third lumbar vertebrae or as far caudally as the fifth lumbar vertebrae. They are not as freely mobile as cows’ ovaries, and, in non‐pregnant animals, they are often located 5–10 cm directly cranial to the upper third of the ipsilateral ileal shaft in the sublumbar region. The broad ligament, a peritoneal fold that attaches to the abdominal and pelvic walls, supports the reproductive tract. It consists of three regions (mesovarium, mesosalpinx, and mesometrium). The ovary is suspended by the mesovarium (the cranial border of this forms the suspensory ligament of the ovary). The mesosalpinx is a continuation of the mesovarium from its lateral border and suspends the uterine tube and forms the lateral proper ligament of the ovary, and the medial wall of the shallow ovarian bursa. The round ligament of the uterus extends from the uterine horn to the inguinal canal. The anatomy of the broad ligaments can be clinically relevant as exteriorization of the uterine horns or ovaries during surgical procedures is limited by the suspensory ligaments. The mesovarium is located for ecrasement and ligation during ovariectomy, and in standing animals, local anesthetics are applied to the mesovarium prior to performing these procedures. The equine uterus is bicornuate, the uterine horns are moderately developed in the mare and are approximately 20–25 cm long, with the body being slightly shorter to almost equal in length. When viewed from above it is Y‐ or T‐shaped. The uterus is capable of wide variations in size, shape, and location based on stage of estrous cycle, seasonal influences, or pregnancy. The uterine horns are generally located in the abdominal cavity and in the non‐pregnant mare the uterine body is found immediately in front of and frequently ventral to the cranial brim of the pelvis. It can also be partially located in the pelvic cavity where it is continuous caudally with the cervix. The cervix is approximately 6 cm long and lies in the pelvic cavity where it rests on the bladder and urethra. The vagina of the mare is defined into two regions. The cranial vagina has only reproductive functions and extends from the cervix to the entrance of the urethra. The caudal region, the vestibule, extends from the urethral opening to the external vulva, and has both reproductive and urinary functions. A relatively distinct transverse fold (hymen) on the floor and sides of the vaginal wall is located immediately cranial to the external urethral orifice. Many texts refer only to the cranial region as the vagina and describe the vestibule region separately. The male reproductive tract consists of bilateral testes, epididymides and ductus deferens, the accessory sex glands, and the penis. The scrotum protects and supports the testes and consists of four layers: skin, tunica dartos muscle, scrotal fascia, and parietal vaginal tunic. The scrotum of the stallion is located high in the inguinal region and is much less pendulous than in the ruminant species. The testicles of a normal stallion are palpable as two ellipsoidal structures and the long axes are horizontally positioned. Normal orientation of the testicle is determined by palpation of the tail of the epididymis and the ligament of the tail of the epididymis at the caudal pole of each testicle. There is a full complement of accessory genital glands in the horse, consisting of paired seminal vesicles, the prostate, the paired bulbourethral, and the paired ampullae of the ductus deferens. These are fully developed in the stallion and retain their juvenile status following castration in the gelding. The equine penis is of the musculocavernous type and consists of columns of erectile tissues. The cavernous spaces making up the erectile tissue of the penis are the corpus cavernosum, corpus spongiosum and corpus cavernosum glandis. Engorgement of these spaces with blood from branches of the external pudendal arteries and the obturator arteries is responsible for erection. The cavernous spaces within the penis are continuous with the veins responsible for drainage. When in a resting position, the penis measures about 50 cm with close to 20 cm being held within the prepuce. During maximal erection, it becomes three times as long. The prepuce in the horse is unique; the external lamina continues with the internal lamina at the level at which the preputial orifice is outlined, as in any other species, but the internal lamina makes an additional fold called the preputial fold. This fold allows for considerable lengthening of the penis on erection. The preputial fold separates the internal lamina from the free part of the penis and it also has both external and internal layers. The transition between the two layers outlines the preputial ring (entrance to the prepuce), which lies just within the preputial orifice. The preputial ring appears as a thick ring on the surface of the penis when it is protruded from the prepuce. A combined summary of the arteries, veins, and nerves supplying the male and female urogenital tract is provided in Table 12.1. The veins of the pelvic cavity by and large are satellite to the arteries; deviations are noted in the table. The pelvis and pelvic organs have both a somatic and an autonomic component. While the somatic innervation is both sensory and motor in function and relates predominantly to the external genitalia and the pelvic floor, the autonomic innervation provides the sympathetic and parasympathetic nerve supply to the pelvic organs. The general considerations regarding anesthetic drug selection, delivery, and monitoring techniques in animals undergoing urogenital surgery using sedation or anesthesia are similar to those of the non‐pregnant animal. However, changes in maternal physiology and concerns about fetal viability may further influence drug selection, patient positioning, and monitoring in pregnant mares. Table 12.1 Summary of urogenital organs and their blood supply and innervation. Data derived from: Sisson et al. (1975), Dyce et al. (1996), Budras et al. (2009), Auer and Stick (2012), Singh (2018). The mare has a relatively long gestation period with the average length being approximately 340 days, and the range of normal gestation is considered to be 320–360 days (Rossdale 1993). Pregnancy causes major adaptations in both physiology and anatomy and creates increased metabolic demands due to the growing fetal and uterine mass. Most of these adaptations influence anesthetic management of periparturient mare. Since almost all anesthetic agents depress cardiovascular and respiratory function, the physiological alterations occurring during pregnancy that have the most significant effect on anesthesia are those affecting these systems. The majority of the changes become more pronounced as gestation proceeds and in late gestation, can cause exacerbation of the cardiopulmonary changes associated with anesthesia and recumbency primarily because of decreases in respiratory reserve due to cranial displacement of the diaphragm. There are limited studies on cardiopulmonary effects of pregnancy in the horse, but a number of references from other species, particularly women and sheep from which much of the information is extrapolated. Tidal volume and minute ventilation increase early in pregnancy in woman, with greater than 50% increases seen at term (McAuliffe et al. 2002). It has been well documented in humans that pregnancy reduces functional residual capacity (Bobrowski 2010), which reduces respiratory reserve. For the lungs to function as efficient gas exchangers, large mammals must avoid uneven distribution of ventilation and perfusion resulting from compression of the lung by the weight of the thoracic and abdominal viscera and changes in cardiac output. In the horse, the largest portion of the lung is in the dorsal aspect of the thorax with relatively little lung tissue lateral to the heart. In addition, the diaphragm slopes steeply ventral and cranial so in the non‐pregnant horse the lungs lie dorsal to the abdominal cavity. As a result of this anatomical arrangement, the lungs are not compressed in the standing animal, and the weight of the abdominal viscera below the diaphragm typically aids lung expansion (Sorenson and Robinson 1980). However, has been shown in pregnant Shetland ponies in the standing position that as gestation advances, the ventral (dependent) lung regions are gradually less ventilated with a relative shift of regional ventilation toward dorsal (non‐dependent) regions and that this situation reverses after foaling (Schramel et al. 2012). This could add to the respiratory compromise observed when horses are placed in dorsal recumbency during anesthesia. During pregnancy in woman, the circulating blood volume increases but hemoglobin and plasma protein concentrations fall. Cardiac output increases throughout pregnancy, peripheral resistance decreases, but arterial blood pressure does not change (Robson et al. 1987). Near term, woman can become acutely hypotensive when placed on their backs due to aortocaval compression (McAuliffe et al. 2002). Aortocaval compression has not been specifically demonstrated in pregnant animals but heart rate, respiratory rate, and mean uterine artery blood pressure increased and arterial oxygenation decreased in cows during the third trimester of pregnancy, when positioned in dorsal recumbency (Dunlop et al. 1994), which suggests similar considerations exist in large animals. Additionally, in the pregnant animal uterine blood flow is not subject to autoregulation and is directly proportional to perfusion pressure and inversely proportional to uterine vascular resistance. Anything leading to vasoconstriction, hypovolemia or a decrease in arterial blood pressure will therefore reduce uterine blood flow and consequently the supply of nutrients and oxygen to the fetus (Taylor 1997). Doppler sonographic studies in the horse have illustrated a decrease in the peripheral blood flow resistance in the first weeks of pregnancy and an increase in uterine blood flow volume (BFV), in the last trimester of pregnancy where BFV increased 50‐fold in the uterine artery (2.5 ml/second at day 16 pregnancy to 124.4 ml/second near term). The diameter of the uterine artery was also shown to increase fourfold from 4 to 16 mm during gestation in mares. The distinct rise in uterine BFV in the last trimester is consistent with fetal growth as the metabolic demands of fetal tissues increase (Fowden et al. 2000a, Klewitz et al. 2015). Additional metabolic demand is met by increasing the supply of nutrients to the fetus from the placenta (Hay 1995). The horse’s placenta is classified as diffuse because the fetus is in contact with the allantoamnion inside the chorioallantois and has a complete set of layers, including endometrial epithelium, maternal connective tissue, and maternal endothelial cells, therefore, classified as epitheliochorial with attachments to the entire endometrium (Furukawa et al. 2014). These three layers represent different levels of barriers to drug transfer prior to the three tissue layers also present in the fetus, namely fetal chorionic epithelial cells, fetal connective tissue, and endothelial cells. Many studies regarding drug transfer across the placenta for human application have been done in ruminants and pigs, who share the epitheliochorial arrangement of the placenta with the horse. However, ruminants have a cotyledonary placenta; the pig’s is diffuse like the horse. The number of layers in the horse’s placenta could delay drug transfer to the fetus, but focal thinning can occur which would counter that (Furukawa et al. 2014). Ultimately, it is placental blood flow that influences drug transfer (Mihaly and Morgan 1983). The size of the uterus is governed by the size of the mare and in turn determines the available area for placentation and, hence, fetal growth (Allen et al. 2002). The placenta is a metabolically active organ and uses a range of substrates to meet its energy requirements which are higher than either the mare or the fetus (Hay 1991; Vaughan and Fowden 2016). Oxygen consumption by the combined uteroplacental tissues is similar to other species with epitheliochorial and hemochorial (humans) placentation and increases by 25–50% between mid‐gestation and late gestation in the horse (Fowden et al. 2000b; Hay 2006; Vaughan and Fowden 2016). Glucose consumption per kg of combined uteroplacental tissues also increases between mid‐gestation and late gestation in the horse (Fowden et al. 2000b). The equine uteroplacental tissues use 5 times more oxygen and 10 times more glucose than the fetus at both mid‐ and late gestation and are sensitive to changes in their nutrient supply producing prostaglandins in response to undernutrition (Fowden et al. 1994; Fowden et al. 2000b; Molina et al. 1991; Macdonald et al. 2000). Placental transfer of drugs is governed by the physicochemical properties of the drug and anatomical features of the placenta. The four main physicochemical properties of the drug are molecular size, protein binding in maternal blood, degree of ionization, and lipophilicity (Mihaly and Morgan 1983; Reynolds 1987, 1998), which provide a diffusion constant, unique to each drug. All four properties influence the behavior of the drug and cannot be considered individually, except for degree of ionization, which on its own can prevent placental crossing. Transfer of drugs can occur by simple diffusion, facilitated diffusion via transport systems, active transport, and pinocytosis. Most drugs used in anesthesia have large diffusion constants – low molecular weights, high lipid solubility, and poor ionization – and diffuse rapidly across the placenta. The concentration of drug in the umbilical vein of a fetus however is not that to which the fetal target organs such as the heart and brain are exposed as most of the umbilical blood passes through the liver, where the drug may be metabolized or sequestered. The remainder of the umbilical blood passes through the ductus venous to the vena cava where it is diluted by drug‐free blood from the hind end of the fetus. Thus, the fetal circulation protects vital tissues and organs from exposure to sudden high drug concentrations and when blood returns to the maternal circulation biotransformation and elimination is enhanced; therefore, the effects of anesthetic drugs in the fetus in utero could be considered more benign. Conversely, a neonate delivered under the effects of anesthetic drugs would need of its own biotransformation and elimination as soon as the placental unit is disrupted, and the effects of anesthetic drugs could be more profound given their underdeveloped systems. There are a number of surgical procedures in which standing surgical interventions are the procedure of choice. When making the decision to either perform a surgery standing or under general anesthesia, there are several factors to consider. The first and likely most important is the size and disposition of the horse as this will impact the safety of the horse and the personnel performing the procedure. Very small patients, such as miniature horses, may make the procedure more difficult for the surgeon to perform safely, as well as to maintain aseptic technique. Conversely, draft horses with a higher risk of post‐operative complications compared to light breed horses following general anesthesia (Gleed and Short 1980; Olson 2002; Kraus et al. 2003) and good temperament may be better suited to standing interventions. Debilitated horses may also be at higher risk of complications with general anesthesia and may be better candidates for standing procedures. In one report, several debilitated horses could not maintain appropriate blood pressures so general anesthesia was aborted and the procedure was performed standing at a later time (Arnold et al. 2010). Fractious horses or those horses that do not respond appropriately to sedation may be patients that are not ideal candidates for standing surgical procedures. Appropriate facilities are also needed to safely perform standing surgical procedures and facilitate maintaining an aseptic surgical field and minimize contamination of the surgical site. The ability to provide appropriate analgesia to which might include both local analgesia and systemic medication is also a determinant of feasibility. In most cases of urogenital disease, the horse only displays mild discomfort and systemic analgesia can be provided with NSAIDs, in addition to medications utilized for sedation (alpha‐2 adrenergic agonists and opioids). In situations where there is complete obstruction of the urinary tract (i.e. urethral obstruction) and the horse demonstrates moderate‐to‐severe signs of abdominal pain, a standing surgical procedure may be contraindicated (DeBowes 1988). In order for standing surgery to be performed optimally, appropriate patient restraint is critical. This typically involves the horse being contained in standing stocks, which limits the horse’s ability to move (Figure 12.1). The stocks should be located in a quiet location, with minimal foot traffic, and a non‐slippery flooring (Graham and Freeman 2014). These stocks ideally should be adjustable to accommodate differences in height and length of the horses; optimal stocks have padding and full rear door both for horse and surgeon safety (Beard 1991). In procedures where surgical access involves the flank, the height of the sides of the stocks needs to be adjusted so that the surgical field is not contaminated by the bars. Because of the location of the surgical site (either caudal abdomen or perineal location), the tail should be wrapped to prevent contamination of the surgical site and can be tied overhead to the stocks (Beard 1991). One additional benefit of the tail‐tie is for additional support of the hindlimbs if the horse develops hindlimb weakness following administration of epidural anesthetics (Beard 1991). For lengthy perineal surgical procedures in mares, placing and maintaining a urinary catheter can prevent surgical site contamination, especially since the use of alpha‐2 adrenergic agonists results in diuresis (Thurmon et al. 1984; Tranquilli et al. 1984; Beard 1991; Alexander and Irvine 2000; Valverde 2010). Figure 12.1 Standing surgery room. This set of stocks is contained within a dedicated room specifically for standing surgical procedures. The stocks have a non‐slip flooring, have adjustable bars to accommodate different sized horses and that can be opened if a horse falls, have an overhead bar to tie the tail, and have padded forward and rear bars. Note that there is also a door at the front of the room, providing a secondary exit for personnel and the horse. For shorter length urogenital procedures (standing castration, perineal urethrotomy, partial phallectomy), sedation has been performed with intravenous xylazine hydrochloride (0.5–1.0 mg/kg) or detomidine hydrochloride (0.01–0.02 mg/kg) with or without the addition of butorphanol tartrate (0.05–0.01 mg/kg), although other alpha‐2 agonists can also be used (Climent et al. 2009; Arnold et al. 2010; Adams and Hendrickson 2014). These doses can be re‐administered as needed throughout the procedure. The use of an intravenous jugular catheter will allow quick and easy access for re‐administration of medications (Graham and Freeman 2014). For longer duration procedures or with horses that do not respond as expected to typical doses of sedation, a variety of continuous rate infusions of alpha‐2 adrenergic agonists, with or without the addition of opioids, can be utilized. One benefit of a continuous infusion includes reaching a constant plane of sedation without the peaks and troughs that can accompany bolus dosing (Vigani and Garcia‐Pereira 2014). A number of these continuous rate infusions have been investigated in normal horses for their effects on sedation, ataxia, and analgesia (Solano et al. 2009; Ringer et al. 2012a, 2012b, 2013; Medeiros et al. 2017). Several other continuous rate infusions have been utilized for urogenital or other surgical procedures (Virgin et al. 2010; Adams and Hendrickson 2014; Seabaugh and Schumacher 2014; Potter et al. 2016). A number of reported combinations of alpha‐2 adrenergic agonists and opioids used for continuous rate infusions are listed in Table 12.2. A variety of local anesthesia/analgesia techniques are utilized to facilitate standing urogenital procedures. Many of these techniques can also be utilized to provide supplemental analgesia in anesthetized patients. The most commonly utilized local anesthetics in horses are lidocaine, mepivacaine, ropivacaine, and bupivacaine. These anesthetics differ in their degree of lipid solubility, onset of activity, duration of activity, and toxicity. Each of these four local anesthetics has reported use for both peripheral and epidural anesthesia. In general, the duration of the procedure, as well as clinician familiarity with the anesthetic, often dictates which of these local anesthetics is chosen for a particular procedure. Table 12.2 Continuous rate infusions reported for standing sedation in horses. While site of administration‐dependent, in general terms, lidocaine and mepivacaine are similar in their duration (90–180 minutes and 120–180 minutes, respectively) of activity and have a relatively quick onset; bupivacaine has a longer onset and duration (180–500 minutes) of action (Skarda et al. 2009). One important side effect of bupivacaine is its cardiotoxicity which is magnified with inadvertent intravenous administration (Skarda et al. 2009). Ropivacaine has a quick onset of action and a longer duration of action (180–360 minutes) than lidocaine and mepivacaine and is reported to have less cardiotoxicity than bupivacaine (Skarda et al. 2009). Especially for surgical procedures performed in the flank region of the horse, infiltrative blocks can be especially useful. Methods of infiltration include the use of an inverted L block around the proposed surgical site or direct infiltration of local anesthetic at the surgical site (Seabaugh and Schumacher 2014). The technique is simple as there are no specific anatomic landmarks that have to be identified and relatively large volumes of local anesthetic may be safely administered (Skarda et al. 2009). However, local infiltrate at the line of surgical incision has been suggested to increase the risk of infection, and delay healing of the incision site (Moon and Suter 1993). Additionally, deeper tissues may only be partially desensitized especially in cases of flank laparotomy in obese horses (Skarda et al. 2009). Infiltration of local anesthetic is commonly utilized for routine standing castration. Typically for the adult horse (weighing 400–500 kg), 10–15 ml of either 2% lidocaine or 2% mepivacaine is infused either at the proposed incision lines on either side of the median raphe of the scrotum or as a ring block made surrounding the area of proposed incisions (Adams and Hendrickson 2014). Intratesticular administration may also be helpful to supplement this as noted in following text; the total dose should not exceed 2 mg/kg. Local anesthesia is also routinely used for perineal surgeries that are short in duration, including the Caslick’s procedure. Again, approximately 10 ml of either 2% lidocaine or 2% mepivacaine (not to exceed 2 mg/kg) is used along the proposed incision line. Infiltrative anesthesia can also be useful for anesthesia of visceral organs as for example to provide anesthesia of the testicle and spermatic cord during a routine standing castration (Adams and Hendrickson 2014). Intratesticular lidocaine diffuses into the spermatic cord and allows for appropriate anesthesia of the structures within the cord during emasculation. Additionally, partial phallectomy can be performed in the standing gelding or stallion by utilizing a local ring block at the level of the opening of the prepuce (i.e. proximal to the site of phallectomy) (Arnold et al. 2010; Adams and Hendrickson 2014). In mares, local infiltrate of anesthetic into the mesovarium is routinely performed for ovariectomies, whether they are removed via colpotomy, flank laparotomy, or laparoscopy (Virgin et al. 2010; Seabaugh and Schumacher 2014). While paravertebral anesthesia is most commonly performed in ruminant species, but may similarly be performed in horses. Blockade of the dorsal and ventral branches of spinal nerves T18, L1,, and L2 in the horse will provide flank desensitization, but because it can be technically more challenging, it is used less frequently (Moon and Suter 1993). That said, with knowledge of anatomical landmarks it can be readily performed (Delli‐Rocili et al. 2020). The main benefits of this technique are that a smaller dose of local anesthetic are typically required and there are no direct impacts on the healing of the surgical incision site (Skarda et al. 2009). However, the landmarks can be difficult to palpate in heavily muscled horses, and if anesthetic is deposited inadvertently around the third lumbar nerve, there is a potential for motor deficits of the hindlimb (Skarda et al. 2009). Anesthesia and analgesia can be accomplished by either epidural or subarachnoid injection (Natalini 2010). These techniques can be used to anesthetize the perineal or flank regions depending on where the anesthetic or analgesic medications are deposited. The most commonly used technique is the caudal epidural injection, but caudal subarachnoid injection, segmental dorsolumbar epidural injection, and thoracolumbar subarachnoid injection have also been reported (Skarda et al. 2009). (Figure 12.2) Caudal epidural injection can be used to provide anesthesia and analgesia for surgical procedures of the urogenital tract, specifically for the perineal region, caudal reproductive tract (vulva, vestibule, vagina), and the caudal urogenital tract (urethra and bladder) (Natalini 2010). When properly performed, this technique should desensitize several nerves, including the caudal rectal, middle rectal, and pudendal nerves (Skarda et al. 2009). The goal of this technique is to desensitize the surgical site while allowing the horse to stand and maintain function of the hindlimbs (Natalini 2010). Most surgical procedures can be safely performed using a single injection into the sacrococcygeal or coccygeal epidural space. However, if a procedure is anticipated to be prolonged or if additional analgesics may be required in the peri‐operative period, an epidural catheter may be placed. Placement of an epidural catheter can be used to allow repeated injection of anesthetic solution without the need for interruption of the surgical procedure to re‐prep and re‐administer anesthetics by a second epidural puncture (Green and Cooper 1984). Placement of an epidural catheter could also facilitate administration of analgesic medications post‐operatively. There are a number of anesthetics and analgesics that can be administered either on their own or in combination into the epidural space. These medications are commonly chosen based on clinician preference/familiarity and the desired length of anesthesia or analgesia desired. A list of commonly utilized medications, dosages, and anticipated duration of activity are listed in Table 12.3. In general, for surgical procedures, combination therapy with a local anesthetic and either an alpha‐2 agonist or opioid is often utilized. Length of analgesia and anesthesia is typically longer than for the local anesthetic alone. Except for xylazine, most alpha‐2 agonists or opioids do not provide sufficient local anesthesia to be able to perform surgery. As a group, alpha‐2 agonists block C‐fiber conduction through the blockade of substance P (Riedl et al. 2009; Valverde 2010). Additionally, xylazine blocks A‐delta fibers with a more profound effect than other alpha‐2 agonists, giving it the ability to work as an anesthetic (Valverde 2010). Combinations of alpha‐2 agonists and local anesthetics can cause substantial ataxia and occasionally recumbency can result (Robinson and Natalini 2002). This most commonly occurs when too large a volume is used and paralysis is induced from cranial spread of the anesthetic. If there is only partial paralysis, the horse can be supported using a tail‐tie, but if the horse becomes recumbent and distressed, inducing and maintaining anesthesia until the motor effects dissipate may be beneficial (Robinson and Natalini 2002). Alpha‐2 agonists, such as detomidine and xylazine, also cause additional side effects, including perineal edema, perineal sweating, bradycardia, second‐degree atrioventricular blockade, significant sedation, and/or ataxia (Robinson and Natalini 2002). In horses where multiple epidural injections have been performed, incomplete blockade can occur, which may be secondary to presence of fibrous tissue, adhesions, or inability to inject due to changes in anatomy (Natalini 2010). Figure 12.2 Caudal epidural. (a) The site for caudal epidural injection is located by moving the tail dorsal and ventral, to locate the sacrococcygeal or first intercoccygeal space. (b) A 20‐ or 18‐gauge 3.8 cm hypodermic or spinal needle is placed through the skin at the site of the first coccygeal space. (c) 0.9% saline has been placed in the hub of the needle prior to advancement into the epidural space. (d) Once the 0.9% saline has been aspirated into the epidural space by the negative pressure present in the epidural space, the medication volume is injected. There should be no resistance to injection, which can be tested by placing a small air bubble within the syringe. Opioids may also be administered via caudal epidural injection. Methadone, hydromorphone, meperidine and morphine have been used and providing varying onset and duration of effect. Meperidine has been associated with motor weakness whereas morphine is associated with pruritis, which is thought to be related to the local release of histamine (Robinson and Natalini 2002).
12
Anesthetic Management for Urogenital Interventions
Anatomy of Urogenital Systems, Male and Female
The Female Reproductive Tract
The Male Reproductive Tract
Physiological Considerations for Urogenital Interventions
Organ
Innervation
Blood supply
Ovary
Sympathetic innervation through the renal and abdominal aortic plexuses
Ovarian artery (direct branch from the abdominal aorta)
Uterine tube
Same as ovary
Uterine branch of the ovarian artery and cranial branch of the uterine artery
Uterus
Parasympathetic innervation comes from the sacral outflow and reaches the genital tract via the pelvic nerves.
Sympathetic innervation comes from the caudal mesenteric ganglion and plexus and goes to the organs via the hypogastric nerves and pelvic plexus
Uterine branch of the ovarian artery and cranial branch of the uterine artery supply the cranial uterine horn
Uterine artery (branch of the external iliac artery) extends acaudal branch to anastomose with the uterine branch of the vaginal artery (from the internal pudendal artery) to supply remainder of horns and body of the uterus. Satellite veins accompany arteries, but the main venous drainage of the uterus is via the ovarian vein
Vagina
Nerves are derived through the sympathetic plexus and numerous ganglia are present in the adventia
Vaginal artery (branch of internal pudendal artery)
Vestibule and vulva
Pudendal (branches include – deep perineal and superficial perineal nerves) and caudal rectal nerves provide motor innervation to the muscles of the vestibule and vulva, and also sensory fibers to the mucous membrane of the vulva and the skin of the labia
Branches of internal pudendal artery (vestibular branch and ventral perineal artery)
A second blood supply to this region, the obturator artery, terminates by entering the root of the clitoris
Perineum
Pudendal (S2–S4) and caudal rectal nerves (S4–S5)
Internal pudendal artery
Udder
Cutaneous innervation is divided between nerves of the flank and descending (mammary) branch of the pudendal nerve (S2–S4); iliohypogastric (L1), and the substance of the gland by the genitofemoral (L3–L4) nerves
Sympathetic system caudal mesenteric plexus
External pudendal artery
Penis
Pudendal nerve (dorsal nerve of the penis)
Deep perineal nerve supplies ischiocavernosus, bulbocavernosus, urethralis, retractor penis muscle
Sympathetic fibers of the pelvic plexus supply the smooth muscle of the vessels and erectile tissue
External pudendal artery passes through the inguinal canal and supplies the cranial artery of the penis, which anastomoses on the dorsal surface of the penis with the middle artery of the penis (from the obturator) and dorsal artery of the penis (from the internal pudendal)
The veins form an extensive plexus dorsal and lateral to the penis whose blood enters the accessory external pudendal vein but also the obturator and internal pudendal veins
Prepuce
Pudendal nerve
Iliohypogastric nerve (medial branch) supplies skin on ventral abdomen, udder, and prepuce
Genitofemoral nerve (genital branch) cremaster; vaginal tunic; skin of the prepuce, scrotum, and udder
External pudendal artery
Testis
Plexus of autonomic and visceral sensory nerves
Nerves derived from the renal and caudal mesenteric plexuses, form the testicular plexus around the vessels, to which they are chiefly distributed
Testicular artery (from aorta) and its branch, the epididymal artery most of the vascular supply, some from the cremaster artery and the deferential artery also contribute to the testis (these three arteries are connected by numerous anastomosing vessels)
Testicular veins divide and convolute to form the pampiniform plexus, which lies around the coiled testicular artery. The right testicular vein joins the caudal vena cava, and the left testicular vein joins the left renal vein
Scrotum
Genitofemoral nerve, pudendal nerve
Branch of external pudendal artery
Kidney
Sympathetic nerves to kidney are routed through the celiacomesenteric plexus. The vagus contributes to the parasympathetic supply
Renal artery which is a branch of the abdominal aorta. Veins are satellite and ultimately lead to the caudal vena cava
Bladder
Autonomic fibers – the sympathetic hypogastric and parasympathetic pelvic nerves. Sensory fibers are routed from the pudendal nerve
Vaginal (or prostatic artery) and also supplemented by the reduced umbilical arteries
Urethra
Pudendal nerve
Inferior vesicular artery, middle rectal artery, and internal pudendal vein
Maternal Physiological Changes
The Equine Placenta
Drug Transfer Across the Placenta
Sedation and Analgesia for Standing Interventions
Patient Preparation for Standing Urogenital Procedures
Sedation Protocols
Local Anesthesia/Analgesia
Medication(s)
Loading dose (L)
CRI dose (C)
Effects, Comments
Reported procedures
References
Dexmedetomidine
3.5 μg/kg (L)
5 μg/kg/h (C)
Significant decrease in head height during 90 minutes CRI, maximum ataxia was within first 15 minutes and then gradually decreased, significant decrease in tactile stimulation at 30 minutes and significant decrease in auditory stimulation up to 60 minutes after CRI started
None
Medeiros et al. (2017)
Dexmedetomidine + Butorphanol
3.5 μg/kg (L)
3.5 μg/kg/h (C)
20 μg/kg (L)
24 μg/kg/h (C)
Significant decrease in head height during 90 minutes CRI, maximum ataxia was within first 15 minutes and then gradually decreased, significant decrease in tactile and auditory stimulation at 30 minutes after CRI started
None
Medeiros et al. (2017)
Acepromazine + Detomidine + Buprenorphine
0.02 mg/kg (L)
10 μg/kg (L)
~0.6 μg/kg/min (C)
0.01 mg/kg (L)
CNS excitement (attributed to buprenorphine)
Good degree of sedation
Dental and sinus procedures
Potter et al. (2016)
Acepromazine + Detomidine + Morphine
0.02 mg/kg (L)
10 μg/kg (L)
~0.6 μg/kg/min (C)
0.1 mg/kg (L)
Good degree of sedation
Dental and sinus procedures
Potter et al. (2016)
Romifidine
80 μg/kg (L)
30 μg/kg/h (C)
Good degree of sedation, ~ 1 hour recovery from sedation
Redosing of romifidine required (20 μg/kg) for 5/11 dental procedures, 2/11 needed rescue analgesia (butorphanol)
None
Dental or ophthalmologic procedures
Ringer et al. (2012b), Marly et al. (2014)
Romifidine + Butorphanol
80 μg/kg (L)
29 μg/kg/h (C)
18 μg/kg (L)
25 μg/kg/h (C)
Good degree of sedation, more ataxic than romifidine alone, ~ 1 hour recovery from sedation
1/10 needed bolus romifidine (20 μg/kg) for each a dentistry and ophthalmologic procedure, 0 needed rescue analgesia (butorphanol)
None
Dental or ophthalmologic procedures
Ringer et al. (2012b), Marly et al. (2014)
Xylazine
1 mg/kg (L)
0.69 mg/kg/h (C)
Constant plasma concentrations of xylazine at 45 minutes
None
Ringer et al. (2012a)
Xylazine + Butorphanol
1 mg/kg (L)
0.65 mg/kg/h (C)
18 μg/kg (L)
25 μg/kg/h (C)
Constant plasma concentrations of xylazine at 45 minutes
3/10 horses fell (10–15 minutes after loading dose)
4/10 horses were insufficiently sedated (during second hour of sedation)
None
Ringer et al. (2012a)
Detomidine + Buprenorphine
10 μg/kg (L)
0.16 μg/kg/min (C)
6 μg/kg (L)
Good sedation for surgery
Laparoscopic sterilization
Van Dijk et al. (2003)
Xylazine
Butorphanol
Detomidine
0.33 mg/kg (L)
5 mg (L)
20 mg in 1 l polyionic fluids (titrate to effect) (C)
Total detomidine dose for the procedure 9–18 mg
Bilateral laparoscopic ovariectomy
Virgin et al. (2010)
Infiltrative Blocks
Paravertebral Blocks
Epidural and Spinal Anesthesia and Analgesia
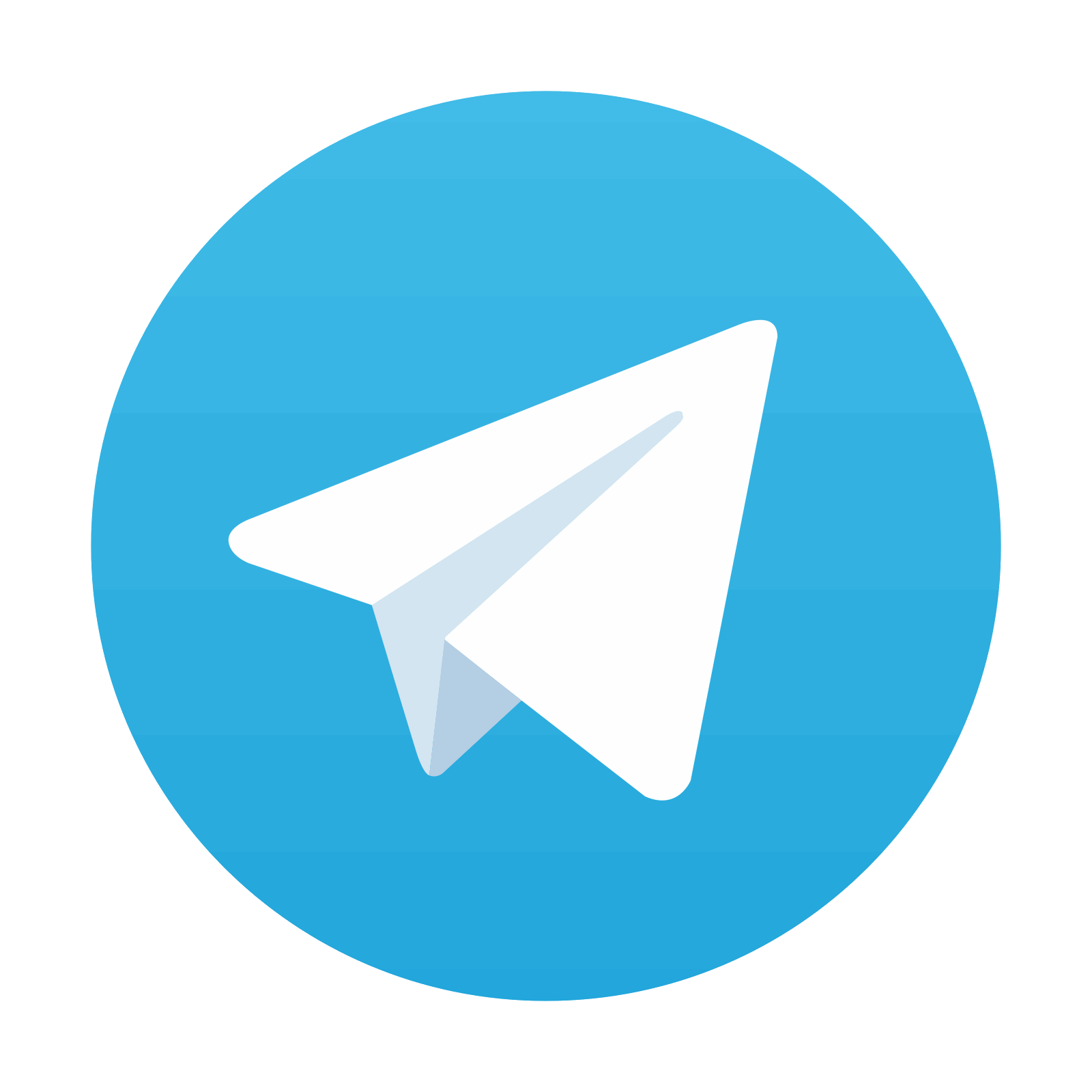
Stay updated, free articles. Join our Telegram channel

Full access? Get Clinical Tree
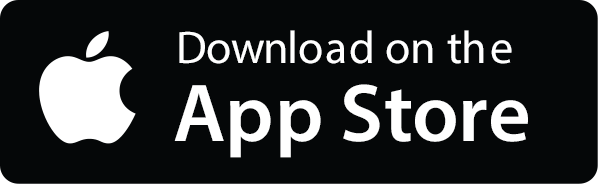
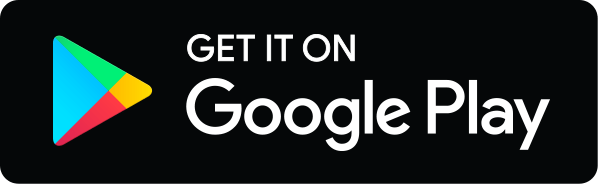