Bianca Martins1 and Manuel Martin‐Flores2 1 Department of Surgical and Radiological Sciences, School of Veterinary Medicine, University of California, One Garrod Drive, Davis, CA, 95616, USA 2 Department of Clinical Sciences, College of Veterinary Medicine, Cornell University, 930 Campus Road, Ithaca, NY, 14853, USA Resolution of ocular procedures in horses can present several particular challenges to the anesthesia provider: many surgeries that are performed under general anesthesia require a central and immobile eye, which is best achieved by the use of neuromuscular blockers. The introduction of these agents to the anesthetic protocol adds a new level of complexity, as it will be reviewed in following paragraphs. In some instances, the procedure might be completed with the horse awake and standing, facilitated by the use of sedatives and locoregional anesthesia. In that case, the anesthetist must be experienced in the use of sedatives to provide a state of “conscious sedation” that allows sufficient cooperation from the animal (or tolerance to the surgery) while retaining the ability to stand with as minimal ataxia as possible. For this approach, knowledge of the relevant anatomy and locoregional techniques is necessary. Aside from these technical challenges, complications might arise from manipulation of the eye during surgery (i.e. cardiac dysrhythmias), or secondary to topical ophthalmic medication. Therefore, a basic understanding of ocular physiology and therapeutics is also necessary. Several ocular pathologies are resolved through surgical interventions in horses. These involve extraocular procedures, such as those involving the eyelids, removal of the globe, and ocular/intraocular surgeries. The globe is located within the orbit and surrounded by periorbital tissues (extraocular muscles [EOMs], retrobulbar fat, among others) and periocular structures (such as the conjunctiva and eyelids). The eyelids are modified upper and lower folds of skin, which form the palpebral fissure (opening), provide mechanical protection for the globe, and spread the tear film. Several muscles are responsible for the closure and opening of the eyelids (Table 2.1). However, in a simplistic way, closure of the eyelids is achieved by contraction of the orbicularis oculi muscle, while the opening of the eyelids is done by a combination of relaxation of the orbicularis oculi muscle, and contraction of the levator palpebrae superioris muscle. All other muscles aid in those movements. In general, motor innervation to the eyelids is provided by the oculomotor nerve (CN III) and facial nerve (CN VII), while the sensory innervation to all aspects of the eyelids is provided by the ophthalmic branch of the trigeminal nerve (CN V). Table 2.1 Muscles of the eyelids, associated function and cranial nerve (CN) innervation. The orbit is a bony fossa that separates the globe from the cranial cavity. In addition to mechanical protection, the orbit provides several foramina and fissures, which are pathways for blood vessels and nerves involved with the ocular maintenance. Within the orbit, a total of seven EOMs are responsible for providing ocular motility – four recti (dorsal, ventral, lateral, and medial rectus), two obliques (dorsal oblique and ventral oblique), and one retractor bulbi muscle (Table 2.2). The four rectus muscles (dorsal, ventral, lateral, and medial rectus) move the globe in the direction of their respective names; the dorsal oblique muscle pulls the dorsal aspect of the globe medially and ventrally; the ventral oblique muscle moves the globe medially and dorsally; and the retractor bulbi, which forms a “cone shape” behind the eye, is responsible for retracting the globe into the orbit. The dorsal, ventral, and medial rectus muscles, as well as the ventral oblique muscle, are innervated by cranial nerve III (oculomotor). The dorsal oblique muscle is innervated by cranial nerve IV (trochlear), while the lateral rectus and the retractor bulbi muscles are innervated by cranial nerve VI (abducens). Table 2.2 Extraocular muscle responsible for movement of the globe, associated movement and cranial nerve (CN) innervation. The globe itself is separated into three layers or tunics. The external or outmost wall of the globe consists of the fibrous tunic, composed by the cornea and sclera, which provides the shape and mechanical support for the intraocular structures. The second, middle layer is the highly vascular uveal tunic or tract, composed by the iris, ciliary body, and the choroid. The third and innermost layer is the neural tunic of the eye, composed by the retina. The lens is located just posterior to the iris and is attached to the ciliary body by the lenticular zonules. The anterior segment comprises all structures from the cornea to the lens (including the cornea itself, the iris, ciliary body, and the lens), and is bathed by the aqueous humor. The anterior segment is divided into two spaces: the anterior chamber (from the cornea to the iris), and the posterior chamber (from the iris to the lens). The posterior segment comprises all structures from the lens to the retina, including the vitreous humor, the choroid, and the retina itself. The cornea, the anterior outermost structure of the globe, is one of the most densely innervated tissues in the body, richly supplied in sensory nerves (mainly pain receptors) to provide corneal protection, with a neuronal density that is 300–600 times greater than the skin epithelium (Rozsa and Beuerman 1982; Brooks et al. 2000). Sensory innervation of the cornea originates from the long ciliary nerves, which are derived from the ophthalmic branch of the trigeminal nerve (CN V). Most of the nerve endings are located on the subepithelial and anterior stromal layers of the cornea and, in the horse, the cornea appears to be most sensitive in the center and less so toward the periphery. The innervation of the uveal tract is made by both sympathetic and parasympathetic fibers. The iris sphincter muscle is partly responsible for controlling the pupil size, while the ciliary body muscle is partly responsible for the lens accommodation and focus. The ciliary body muscle and iris sphincter muscle are supplied by parasympathetic fibers from the oculomotor nerve (CN III) via the short ciliary nerves, and by sympathetic nerve fibers via the long ciliary nerves. Medications that may stimulate or paralyze those neuronal pathways may alter the pupil size, either facilitating or precluding some intraocular procedures, such as cataract removal. The preocular tear film (POTF) is responsible for maintaining an optically uniform ocular surface, lubricating the cornea and conjunctiva, and providing nutrients to the cornea. The tears are mainly composed of three layers: (i) the outer lipid layer, produced by the meibomian glands, and responsible for preventing the evaporation of the tear film; (ii) the intermediate aqueous layer, produced by the orbital (or principal) gland and by the third eyelid gland, composed mainly by water, plus electrolytes, glucose, urea, globulins, lysozymes, and other solids, and responsible for providing the lubrication and nutrients to the cornea; (iii) the inner mucin layer, produced by the conjunctival goblet cells, responsible for anchoring the aqueous film to the corneal epithelium. Also, the POTF contains antibacterial cytokines and exerts some control over the ocular surface flora; therefore, the incidence of ocular surface infections is elevated in tear‐deficient patients. Even though the innervation to the lacrimal gland is not yet completely understood, it is known that the lacrimal gland receives sensory input from the lacrimal nerve, which is a branch of the trigeminal nerve (CN V). Also, the lacrimal branch of the facial nerve (CN VII), sympathetic and parasympathetic fibers are involved in the process. In general, some cholinergic drugs stimulate lacrimation, while anticholinergic drugs decrease the tear production. Even though keratoconjunctivitis sicca (“dry eye”) is rare in horses (and usually related to trauma), it is important to remember that general anesthesia may decrease or completely temporarily stop tear production in horses, increasing the risk of corneal erosions and ulcers. Tear replenishment with lacrimomimetics during anesthetic procedures is, therefore, recommended. Artificial tears in ointment or gel formulations are preferable over drops or solutions since those forms stay longer on the eye. The aqueous humor is a transparent fluid that resembles an ultrafiltration of plasma and fills and nourishes the anterior segment structures. This fluid is produced by the ciliary body, flows from the posterior chamber through the pupil, into the anterior chamber, and drains out of the eye via the iridocorneal angle into the intrascleral venous plexus (conventional outflow) or via the supraciliary‐suprachoroidal space into the scleral vessels (unconventional outflow). In healthy conditions, aqueous humor is constantly produced and drained, establishing a normal intraocular pressure (IOP). Decreased IOPs are usually associated with intraocular inflammation (uveitis), while elevated IOP is one of the main and most devastating components of the glaucoma syndrome, a progressive condition that leads to blindness. The aqueous humor is formed by three mechanisms: (i) diffusion of solutes following a down concentration gradient; (ii) ultrafiltration from plasma by hydrostatic force; (iii) active secretion by the ciliary body epithelium, utilizing energy to secrete solutes against a concentration gradient. This active process is catalyzed by the carbonic anhydrase enzyme, leading to entry of sodium (Na+) and bicarbonate (HCO3−) into the aqueous, establishing an osmotic gradient leading to the entry of water into the posterior chamber. Topical and systemic carbonic anhydrase inhibitors will thus decrease the rate of aqueous humor formation, resulting in lower IOP values. Even though no significant diurnal IOP variation has been detected in horses, the effect of head position on IOP is well documented, with increased IOPs detected when the head is below heart level (Komaromy et al. 2006). This phenomenon is particularly relevant during hoisting after induction of anesthesia, with IOP values up to 80 mmHg obtained while the horse is hoisted. This should be taken into consideration when anesthetizing and hoisting patients where an increase in IOP could be detrimental, such as those suffering from glaucoma, deep corneal ulcers, descemetocele, or corneal rupture (Monk et al. 2017). Any sensory stimulation to the eye and its periocular structures may result in stimulation of the vagal nucleus, leading to a slowing of the heart. This oculocardiac reflex has been documented secondary to traction of the globe during enucleations, and during intraocular surgeries in the dog, cat, horses, humans, and other species. Sensory fibers of the ophthalmic branch of the trigeminal nerve (CN V) to its nucleus make up the afferent pathway of this reflex, while the efferent pathway is via the vagus nerve (CN X) to the heart. The most common clinical sign associated with this phenomenon is bradycardia; however, cardiac arrest and ventricular fibrillation have also been documented (Raffe et al. 1986; Oel et al. 2014). Several ophthalmic procedures, such as surgical correction of defects in the eyelids (often of traumatic origin), placement of episcleral cyclosporine implants for immune‐mediated keratitis, intra‐stromal injections for corneal abscesses, and even removal of the globe, can be performed under sedation and regional anesthesia. Sedation is tailored to produce a cooperative horse that can tolerate surgical manipulation while standing as stable as possible. Regional anesthesia is also provided to achieve sensory blockade, motor blockade, or both. Sedation in horses is most commonly achieved with the use of ɑ2‐agonist agents. These agents provide reliable sedation, analgesia, and result in an animal that typically stands with minimal ataxia. While some minor clinical differences are reported between the use of different agents [e.g. less ataxia reported with romifidine (England et al. 1992)], all ɑ2‐agonists in clinical use are suitable sedatives for these purposes, and all can be administered either as repeated boluses or infused over time to prolong the duration of sedation. If sedation is insufficient during stimulating periods of the procedure, boluses of the agent can be administered without altering the infusion rate. Alternatively, the infusion can be titrated according to a horse’s response to surgical stimuli. Doses for constant rate infusions of these agents are summarized in Table 2.3. The author commonly uses opioids such as morphine (0.05–0.1 mg/kg) or butorphanol (0.01–0.02 mg/kg), or the phenothyazine agent acepromazine (0.005–0.01 mg/kg), to enhance sedation from ɑ2‐agonists in horses that respond to surgical stimulation. Table 2.3 Alpha 2‐agonist drugs loading dosages and infusion rates for standing sedation for ophthalmic procedures. a The range has been expanded based on the dosages and experiences at the author’s institution. When choosing which a2‐agonist to use, it is important to discuss with the ophthalmologist or surgeon the expected duration of the procedure. In general, detomidine and romifidine are most commonly used for longer procedures (>60 minutes), while xylazine is usually chosen for shorter procedures. While ketamine can be utilized at lower dosages to provide standing sedation, it has been shown to increase the IOP in horses (Ferreira et al. 2013). On the other hand, sedation with xylazine and detomidine decreases the IOP (Holve 2012) and are likely safer options for patients with increased IOP, such as those with glaucoma or deep corneal ulcers. Anesthesia of the eye and/or surrounding structures is also necessary, in addition to sedation, for performing ocular procedures in the standing horse. Moreover, a combination of sedation and local anesthesia might be used to facilitate ocular exams by decreasing muscular tone of the eyelid and allowing better exposure of the globe. Akinesia of the eyelids is achieved by the desensitization of the auriculopalpebral nerve, a branch of the facial nerve, which innervates the orbicularis oculi muscle. Desensitization of this nerve facilitates examination of the eye and improves the surgical field, but does not provide anesthesia (sensory block) to the lids. The auriculopalpebral nerve runs superficial over the temporalis muscle and dorsal orbit and can be palpated dorsal to the zygomatic process of the temporal bone (Figure 2.1). It can be desensitized at this location, or alternatively, at the depression found caudally to the mandible and ventral to the temporal portion of the zygomatic arch. Surgical procedures involving the lids that are expected to produce pain also require sensory blockade, which may be achieved by desensitizing four nerves that surround the eye (Figure 2.2). The supraorbital (or frontal) nerve can be desensitized as it emerges from the supraorbital foramen, on the dorsolateral aspect of the orbit (Figure 2.1). Desensitization of this nerve provides sensory block to the central portion of the upper eyelid. Anesthesia of the lacrimal nerve, located dorsolateral to the lateral canthus, provides sensory block to the lateral canthus and lateral portion of the eyelid. The medial canthus and medial aspect of the lids are desensitized by anesthetizing the infratrochlear nerve, located dorsomedial to the medial canthus of the eye. The zygomatic nerve, located on the ventrolateral aspect of the orbit, provides sensory block to the central portion of the lower lid. Each nerve may be blocked individually to provide complete sensory block of these structures. Alternatively, a ring block may be performed so that all four nerves are involved. Excessive use of local anesthetics during infiltration, however, can disrupt the anatomy and result in edema, which may complicate the conditions of the surgical field. Figure 2.1 Lateral view of an equine skull. The blue line indicates where the auriculopalpebral nerve can be palpated and blocked as it courses over the zygomatic arch. The red arrow indicated the supraorbital foramen where the supraorbital (frontal) nerve emerges and can be blocked. The green circle indicated the bony orbit. Procedures involving not only the surrounding structures but also the globe itself can be performed in many horses under sedation. Foreign bodies might be removed under a combination of sedative and locoregional techniques as mentioned above. In addition, topical desensitization of the cornea should be performed and can be achieved with topical ophthalmic anesthetics, such as tetracaine 0.5% and proparacaine 0.5% ophthalmic solutions, applied directly to the ocular surface. In general, the maximum anesthetic effect is achieved within 5–10 minutes when either tetracaine or proparacaine are used, and last approximately 20–25 minutes. If one drop is used, full desensitization of the cornea is not achieved with aqueous solutions of proparacaine and tetracaine, and some sensation (though minimal) might still be present. However, the instillation of two drops of aqueous 0.5% tetracaine, one minute apart, results in full desensitization of the cornea, with the same duration of action (Monclin et al. 2011). A viscous preparation of 0.5% tetracaine is currently available. It provides complete desensitization of the cornea within 10 minutes, with a duration of approximately 30 minutes after initial administration (Kalf et al. 2008; Sharrow‐Reabe and Townsend 2012). A Subconjunctival injection of anesthetic agents is also a viable option for ocular surface anesthesia, and usually increases the duration of the anesthetic effect. The administration of 2% mepivacaine into the subconjunctival space leads to almost complete corneal anesthesia in around 10 minutes, for a total duration of 120 minutes. Bupivacaine and lidocaine can also be used, with maximum effect reached between 15 and 25 minutes after administration, and total duration of around 70–80 minutes (Jinks et al. 2018). Topical 1% morphine sulfate, and topical 1% nalbuphine solution do not result in analgesia of the cornea in horses (Gordon et al. 2018; Wotman and Utter 2010). Figure 2.2 Lateral view of an equine head. Colored dots indicate the location where blockade can be performed to provide local anesthesia of the skin and structures surrounding the eye. Green is the supraorbital (frontal) nerve, blue is the lacrimal nerve, orange is the infratrochlear nerve, and red is the zygomatic nerve. In addition to procedures involving the surface of the globe, removal of the eye can also be performed in the standing horse. There are different techniques for desensitizing the relevant structures required for enucleation. Several nerves must be desensitized if complete akinesia and anesthesia of the eyeball is to be achieved, including the optic, oculomotor, abducens, and trochlear nerves, and the maxillary an ophthalmic branches of the trigeminal nerve. In addition, the surrounding structures may also be desensitized, as described above. A retrobulbar block is likely the most commonly used locoregional technique for achieving anesthesia of the globe. Retrobulbar anesthesia requires injection of the anesthetic agent into the extraocular muscle cone (EOMC), that is, the EOMC needs to be punctured (Figure 2.3
2
Anesthetic Management for Ocular Interventions
Introduction
Anatomy and Physiology of the Eye
Eyelid muscle
Function
Innervation
Orbicularis oculi
Closure of eyelids
Facial (CN VII)
Levator palpebrae superioris
Elevation of upper eyelid
Oculomotor (CN III)
Retractor anguli oculi
Lengthening palpebral fissure
Facial (CN VII)
Corrugator supercilli
Assist in elevation of upper eyelid
Facial (CN VII)
Malaris
Depression of lower eyelid
Facial (CN VII)
Levator anguli oculi medialis
Lengthening palpebral fissure
Facial (CN VII)
Müller
Elevate upper eyelid
Sympathetic fibers
Extraocular muscle
Function
Innervation
Dorsal rectus
Pull globe upward
Oculomotor (CN III)
Ventral rectus
Pull globe downward
Oculomotor (CN III)
Medial rectus
Pull globe medially
Oculomotor (CN III)
Lateral rectus
Pull globe laterally
Abducens (CN VI)
Dorsal oblique
Move globe medially and ventrally
Trochlear (CN IV)
Ventral oblique
Move glove medially and dorsally
Oculomotor (CN III)
Retractor bulbi
Retract globe
Abducens (CN VI)
Tear Production
Aqueous Humor and Intraocular Pressure
Oculocardiac Reflex
Sedation for Ocular Procedures
Drug
Loading dosage
Infusion rate
References
Xylazine
0.5–1.0 mg/kg
0.7 mg/kg/h
Ringer et al. (2012)
Detomidine
0.008–0.01 mg/kg
0.006–0.02 mg/kg/h
Gozalo‐Marcilla et al. (2019)a
Romifidine
0.08 mg/kg
0.03 mg/kg/h
Marly et al. (2014)
Locoregional Anesthesia of the Eye and Surrounding Structures
Locoregional Anesthesia of the Eyelid
Locoregional Anesthesia of the Globe
Retrobulbar Block
Stay updated, free articles. Join our Telegram channel

Full access? Get Clinical Tree
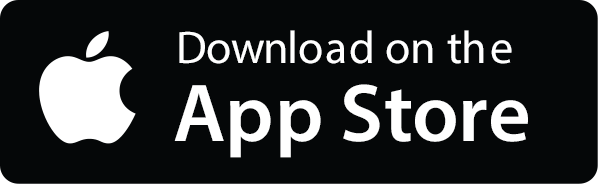
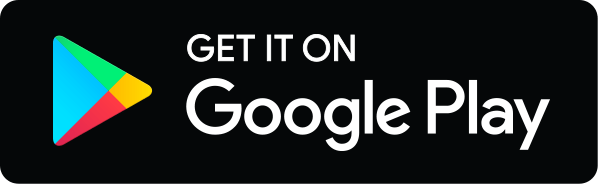