Stephanie Keating and Ryan Fries Department of Veterinary Clinical Medicine, College of Veterinary Medicine, University of Illinois, 1008 West Hazelwood Drive, Urbana, IL, 61802, USA While there are many similarities in cardiovascular physiology and pathophysiology among mammalian species, the unique aspects of equine anatomy, temperament, pharmacodynamics, and pharmacokinetics result in markedly different anesthetic practices between horses and other domestic species. These differences limit the range of anesthetic and cardiovascular agents that are administered to horses, as well as the applicability of anesthetic methodologies reported in other species. An additional challenge is the paucity of literature evaluating anesthetic management of horses with many different cardiovascular conditions. Despite these limitations, knowledge of the underlying pathophysiology allows for targeted goals for key cardiovascular parameters, including heart rate, systemic vascular resistance (SVR), pulmonary vascular resistance (PVR), contractility, preload, and arrhythmias. Ultimately, anesthetizing a horse with cardiovascular disease should be performed with a solid understanding of the underlying pathophysiology and cardiovascular goals, while acknowledging the considerations specific to equine anesthesia. Horses possess a four‐chambered heart identical in function and anatomical layout with other mammals (Budras et al. 2012). In addition to mechanical pumping, the heart has inherent electrical properties that allow generation and propagation of action potentials through the cells. In the normal horse, spontaneous depolarization of the sinus node initiates an action potential within the right atrium. Conduction travels throughout both atria and the atrioventricular (AV) node. Subsequently the action potential enters the specialized conduction tissue, known as the His‐Purkinje system. This network is distributed extensively throughout both ventricles and penetrates the entire thickness of the walls. Once excited there is nearly simultaneous activation of both ventricles resulting in mechanical contraction and pumping of blood (Hamlin and Smith 1965). Specific arrhythmias and their consequences related to anesthesia will be discussed later in this chapter. The cardiac cycle is divided into ventricular systole and diastole. Mechanical ventricular systole begins immediately after the QRS complex, with contraction of the ventricular myocardium and closure of the AV valves. Pressure within the ventricles rises rapidly, without forward movement of blood (isovolumetric contraction), until the pressures exceed the aorta and pulmonary artery pressures causing the semilunar valves to open and blood to flow into their respective circulations. Blood flow peaks during the first third of ejection, after which time flow slowly decreases until ejection stops and the semilunar valves abruptly close marking the end of ventricular systole. Ventricular diastole begins at semilunar valve closure. The ventricular pressures, which have been declining due to relaxation of the myocytes, continue to decline rapidly during early diastole, but ventricular volume remains unchanged because all cardiac valves are closed (isovolumetric relaxation). Once ventricular pressure drops below atrial pressure, the AV valves open and blood passively moves from the atria to ventricles resulting in rapid filling of the ventricles. Temporarily the atrial and ventricular pressures equilibrate resulting in minimal or no changes in ventricular pressure or volume (diastasis). Finally, atrial contraction recreates an AV pressure gradient that produces augmented ventricular filling. In healthy resting horses, atrial systole has minimal effects on ventricular filling and cardiac performance. However, the absence of atrial contraction or loss of AV synchrony on exercising and anesthetized horses can have considerable effects on cardiac performance. A complete review of cardiovascular physiology is beyond the scope of this chapter, and readers are directed to medical physiology textbooks for more information. Alpha‐2 adrenergic agonists are the class of drug most widely used to provide profound, reliable sedation in mature horses for pre‐anesthetic sedation and general restraint, and include xylazine, detomidine, romifidine, dexmedetomidine, and medetomidine. Their sedative effects are accompanied by marked cardiovascular changes mediated both centrally and peripherally. Peripheral activation of alpha receptors on the vasculature increases both systemic and pulmonary vascular resistance. Peripheral reflexes and a reduction in sympathetic outflow result in a decrease in heart rate, contractility, stroke volume, and cardiac output (Wagner et al. 1991; Yamashita et al. 2000; Freeman et al. 2002). Changes in blood pressure are variable, but are classically characterized by an initial increase followed by a decrease back to or below baseline values, although mean arterial pressure remains above 80 mmHg following alpha‐2 administration in conscious, healthy horses (Yamashita et al. 2000). These physiologic changes have the potential to worsen valvular insufficiency in horses and should be considered (Buhl et al. 2007). Second‐degree atrioventricular block is also common following administration (Yamashita et al. 2000). The duration and degree of cardiovascular effects are influenced by the specific alpha‐2 agonist administered, dose, and route of administration, with detomidine having the most marked and longest lasting effects (Wagner et al. 1991; Bryant et al. 1996; Yamashita et al. 2000). Acepromazine is the primary phenothiazine used in horses, providing mild sedation for approximately 6–12 hours depending on the dose and route of administration (Parry et al. 1982). The predominant cardiovascular effect is a reduction in vascular tone and blood pressure mediated by alpha‐1 antagonism on the vasculature. Reductions in blood pressure are dose dependent and prolonged (Muir et al. 1979; Parry et al. 1982; Leise et al. 2007). Heart rate generally does not significantly change following administration, but can be highly variable with increases noted in individuals with lower blood pressure, likely due to the baroreflex (Muir et al. 1979; Parry et al. 1982; Marroum et al. 1994; Buhl et al. 2007). Despite changes in vascular tone, left ventricular internal diameter (an indicator of preload), fractional shortening and other cardiac indices remain unchanged in healthy horses (Buhl et al. 2007). The administration of opioid alone to healthy, pain‐free horses results in increases in heart rate, blood pressure, and cardiac index, without significant changes in stroke volume or SVR (Muir et al. 1978, Skarda and Muir 2003, Carregaro et al. 2006, Figueiredo et al. 2012). This is thought to be the result of increased sympathetic outflow accompanied by central excitatory effects, and is observed with a range of agents, including morphine, fentanyl, hydromorphone, buprenorphine, and butorphanol (Kamerling et al. 1985; Skarda and Muir 2003; Carregaro et al. 2006; Figueiredo et al. 2012; Reed et al. 2019). These affects may not be present when administered to painful horses, are minimal to absent when opioids are co‐administered with alpha‐2 adrenergic agonists or during inhalant anesthesia, which may be due to the suppression of central excitement and sympathetic outflow (Clarke and Paton 1988; Solano et al. 2009; Hofmeister et al. 2008). Benzodiazepines are commonly administered for the co‐induction of anesthesia and as part of total intravenous anesthesia protocols in horses. Benzodiazepines provide anesthetic benefits as centrally acting muscle relaxants with minimal cardiovascular effects. Even when administered above clinically recommended doses, diazepam did not result in any significant change in heart rate, blood pressure, or cardiac output (Muir et al. 1982). Guaifenesin is a centrally acting muscle relaxant, and is used similarly to benzodiazepines as an anesthetic adjunct in equine anesthesia. The administration of GG alone at doses that cause recumbency does not significantly affect heart rate, right atrial pressure, pulmonary artery pressure, or cardiac output, but does reduce arterial blood pressure (Hubbell et al. 1980). However, cardiovascular function was not significantly different in horses receiving either GG or diazepam in conjunction with xylazine and ketamine, to induce anesthesia (Brock and Hildebrand 1990). In addition to use as a co‐induction agent, GG has demonstrated cardiovascular advantages when combined with other agents to provide partial or total intravenous anesthesia compared to inhalant alone (Taylor et al. 1998; Yamashita et al. 2002). Ketamine is an N‐methyl‐D‐aspartate (NMDA) antagonist that provides analgesia and dissociative anesthetic effects. In horses, ketamine is routinely administered as an induction agent, and incorporated into partial and total intravenous anesthesia protocols. The cardiovascular effects of ketamine are variable depending on the dose, concurrent administration of other drugs, and physiologic status. In healthy dogs and humans, the administration of ketamine alone results in sympathetic stimulation with dose‐dependent increases in heart rate, cardiac output, arterial blood pressure, and coronary blood flow, which is met by increases in myocardial oxygen demand (Folts et al. 1975; Johnstone 1976). However, when administered to patients with compromised sympathetic outflow, the direct myocardial depression of ketamine predominates resulting in a reduction in ventricular contractility, cardiac output, and systemic blood pressure (Waxman et al. 1980; Christ et al. 1997). While the cardiovascular effects of a ketamine bolus alone have not been evaluated in horses, a favorable cardiovascular profile of ketamine was demonstrated in healthy anesthetized horses, with the infusion of ketamine resulting in higher heart rates and cardiac output compared to concurrent xylazine and ketamine infusions (Mama et al. 2005). Additionally, the administration of a ketamine infusion at 0.4 and 0.8 mg/kg/h in horses did not significantly affect heart rate or mean blood pressure until an infusion duration of six hours when mild decreases were noted (Fielding et al. 2006). The use of propofol alone to induce anesthesia in horses is associated with excitatory signs and elevated heart rate and blood pressure, and is not recommended (Mama et al. 1995). However, when propofol is administered following premedication with alpha‐2 agonists, changes in heart rate and blood pressure are minimal following induction (Nolan and Hall 1985; Matthews et al. 1999). The cardiovascular effects of propofol total intravenous anesthesia following premedication with an alpha‐2 adrenergic agonist are also minimal, with no significant changes in heart rate, SVR, systemic blood pressure, cardiac output, or stroke volume or just mild decreases in blood pressure reported in horses undergoing anesthesia without surgical stimulation (Nolan and Hall 1985; Umar et al. 2007). In equine surgical patients, the combination of propofol with other agents, including alpha‐2 agonists, results in clinically acceptable heart rates and blood pressures (Aguiar et al. 1993; Matthews et al. 1999; Bettschart‐Wolfensberger et al. 2005). In addition to the administration of lidocaine for its local anesthetic and antiarrhythmic effects, lidocaine can also be administered intravenously as an analgesic and anesthetic adjunct during general anesthesia or for analgesia in conscious horses (Robertson et al. 2005). When administered alone to conscious horses, lidocaine does not alter heart rate or blood pressure and electrocardiogram (ECG) values remain within clinically normal values, even when administered at supraclinical doses (Meyer et al. 2001). Although the cardiovascular effects are minimal in conscious horses, lidocaine does not improve cardiovascular performance in sevoflurane‐anesthetized horses despite a reduction in minimum alveolar concentration (MAC) (Wagner et al. 2011). The cardiovascular effects of the currently administered inhaled anesthetics, isoflurane, sevoflurane, and desflurane are characterized by dose‐dependent reductions in systemic blood pressure, cardiac output, and stroke volume (Steffey et al. 1987; Steffey et al. 2005; Wagner et al. 2011). While isoflurane and sevoflurane have similar cardiovascular effects in horses (Grosenbaugh and Muir 1998), cardiac output is better preserved with desflurane when delivered at clinically administered concentrations (Steffey et al. 2005). Dose‐dependent respiratory depression is an additional consideration with these agents, with intermittent positive pressure ventilation resulting in further cardiovascular depression (Steffey et al. 2005). Auscultation is the principal means to evaluate the equine heart (Bonagura 1990). Auscultation alone can diagnose mitral and tricuspid regurgitation with 53% (Naylor et al. 2001) to 100% (Young and Wood 2000) specificity, making auscultation an essential part of the pre‐anesthetic evaluation in horses. Before a diagnosis of a murmur can be made, it is first necessary to identify normal heart sounds. In all horses, the first two heart sounds (S1 and S2) are audible at the level of the apex beat. S1 corresponds to closure of the AV valves at the beginning of systole, while S2 corresponds to closure of the semilunar valves at the end of systole. Diastolic heart sounds are heard in Thoroughbred‐type horses and less commonly in ponies and other smaller horses. There are two diastolic heart sounds, early rapid ventricular filling (S3), and atrial contraction (S4). Murmurs heard between the first two heart sounds are classified as systolic, while murmurs that occur between S3 and S4 are classified as diastolic. Murmurs should be classified based on location (e.g. left apex, left base), intensity grade 1–6 (Levine and Harvey 1950), and timing: systolic, diastolic, or continuous (Littlewort 1962). Specific murmurs will be discussed in detail for each cardiac disease described in this chapter. In addition to normal heart sounds and murmur detection, auscultation is the primary means of detecting any dysrhythmias. Simply, the horse’s heart rate should be obtained and the rhythm characterized (regular, regularly irregular, or irregularly irregular). The normal adult horse has a sinus heart rate of 28–44 bpm at rest with a regular rhythm, while foals and neonates have average heart rates of 70–80 bpm (Patteson 1996). Once a dysrhythmia has been detected, further evaluation with an is indicated. Most horses with mild‐to‐moderate heart disease are subclinical, requiring precise auscultation and palpation to detect heart disease. However, some horses may present with symptoms of heart failure (HF). Clinical signs of HF can be attributed to a combination of reduced cardiac output and increased ventricular filling pressures. Depending on the etiology of the cardiac disease, clinical signs may include: tachycardia, weight loss, weakness, exercise intolerance, pale mucous membranes, weak arterial pulses, ataxia, syncope, tachypnea, dyspnea, nasal discharge, coughing, jugular pulsation, distention of peripheral veins, and peripheral edema of the ventral thoracic, limbs, or prepuce (Marr 2010a). Any horse with these clinical signs should be evaluated fully for cardiac disease, including echocardiography, thoracic radiographs, electrocardiography, and if available direct measurement of pulmonary artery and pulmonary capillary wedge pressure. Elective anesthetic procedures should be avoided or postponed and stabilization therapy administered prior to any necessary anesthetic procedure is required. An ECG is required to definitively diagnose any dysrhythmia. In horses, dysrhythmias can be associated with a wide range of cardiac and noncardiac diseases, including: valvular disease (Reef et al. 1998), congenital defects, pericardial disease (Reef 1993), myocarditis (Diana et al. 2007), myocardial ischemia (Dickinson et al. 1996), toxicosis (Alleman et al. 2007; Doonan et al. 1989), myocardial neoplasia (Delesalle et al. 2002), hypoxia, electrolyte disturbances (Maxon‐Sage et al. 1998), autonomic tone (Bright and Hellyer 2002), septicemia (Dolente et al. 2000), endotoxemia (Cornick et al. 1990), and various drugs (Reimer et al. 1992). An important diagnostic goal in horses with dysrhythmias is to identify any contributing cardiac or noncardiac disease. The relative risks associated with anesthesia will be determined by the underlying etiology of the arrhythmia, the hemodynamic consequences of the arrhythmia, and the ability to maintain normal heart rhythm during an anesthetic procedure. Proper position of electrodes for an ECG and specific anesthetic considerations for specific dysrhythmias are described elsewhere in this chapter. Echocardiography encompasses a number of specific imaging techniques. The two‐dimensional echocardiogram is used to identify lesions of the heart and great vessels, assess myocardial function, and provide a template for guiding contrast echocardiography, color‐coded Doppler echocardiography, and spectral Doppler studies. M‐mode echocardiography is used to measure cardiac size and ventricular function and can be combined with contrast or color‐coded Doppler studies for accurate timing of flow events. Pulsed wave and continuous wave Doppler echocardiography display the direction and velocity of red blood cells within the heart and circulation. Continuous wave Doppler studies are used to calculate pressure gradients in the circulation. Any of the Doppler techniques can be used to identify abnormal or high velocity flow responsible for pathologic heart murmurs. Each Doppler format is complementary to the others: color‐coded Doppler can pinpoint regions of abnormal flow; and continuous wave Doppler quantifies the maximum velocities of blood flow across cardiac lesions. Echocardiographic studies are very useful for the diagnosis and assessment of horses with cardiac murmurs, arrhythmias, or poor exercise performance. A number of cardiac disorders can be evaluated by echocardiography, including: cardiac malformation, valvular heart disease, cardiomyopathy, bacterial endocarditis, pericardial effusion, and congestive HF (Bonagura and Blissitt 1995). When combined with a careful clinical examination, exercise evaluation, and results of ECG, the echocardiogram provides the best overall clinical assessment of the equine heart. Monitoring the cardiovascular system during anesthesia is critical to promptly identify cardiovascular complications allowing appropriate goal‐directed therapies. The American College of Veterinary Anesthesia and Analgesia (ACVAA) guidelines for anesthesia in horses recommend minimum monitoring techniques, including digital pulse palpation, and evaluation of capillary refill time and mucous membrane color in all patients, with additional ECG and arterial blood pressure monitoring if indicated. Palpation of peripheral arteries is a rapid and qualitative method of assessing arterial blood pressure. The pulse pressure difference (systolic – diastolic arterial pressure) is evaluated by assessing how forceful the pulse feels (i.e.: how bounding or palpable the pulse is), while blood pressure can be gauged by how much digital pressure is required to occlude the pulse. Accessible sites for peripheral pulse palpation include the facial, transverse facial, greater palatine, metatarsal, digital, and coccygeal arteries. Direct blood pressure measurement is the most accurate technique and is strongly recommended in horses undergoing inhalational anesthesia. This technique requires the catheterization of a peripheral artery – most commonly the facial, transverse facial, and metatarsal arteries – which is connected to a transducer via a noncompliant fluid line. The pulse pressure waves are then converted to an electrical signal and displayed on the monitor as a pulse pressure waveform with measured systolic and diastolic pressures and a continuously calculated mean blood pressure. An alternative method is to connect the peripheral arterial catheter to an aneroid manometer to provide an ongoing measurement of mean blood pressure. The placement of the aneroid manometer or transducer should be at the level of the right atrium. While this technique provides the most accurate and rapid blood pressure measurement, there are disadvantages and considerations: arterial catheterization may not always be possible, complications can occur (i.e.: hematomas, thromboembolism, infection), catheterization creates a risk of inadvertent intra‐arterial drug administration, and accuracy can be reduced by mechanical factors that create under‐ or overdamping of the system. Non‐invasive blood pressure measurement techniques are possible in foals and mature horses, and include the Doppler flow method and oscillometric method. The accuracy of oscillometric blood pressure readings in anesthetized adult horses is variable and influenced by the specific monitor, cuff size, cuff site, position of the horse and whether the horse is hyper‐, hypo‐, or normotensive (Hatz et al. 2015; Heliczer et al. 2016; Tearney et al. 2016; Yamaoka et al. 2017). Furthermore, interpretation of study findings are confounded by comparison of oscillometric and direct blood pressure readings from different anatomical locations. While oscillometric monitors may offer the benefit of following blood pressure trends in mature horses, relying on oscillometric derived values to provide guidance on when to institute or discontinue hemodynamic therapies should be considered carefully. In foals, oscillometric readings are also variable based on the monitor used, cuff site, and hemodynamic status, but agreement with invasive blood pressure readings is generally acceptable (Nout et al. 2002; Giguère et al. 2005). Monitoring the ECG of the anesthetized horse provides information about heart rate and rhythm. Due to the extensive Purkinje system throughout the equine myocardium, simultaneous electrical activity within the left and right ventricular myocardium cancel each other out leaving the electrical activity within the interventricular septum and parts of the left ventricular free wall creating the vectors detectable on a surface ECG. Due to the electrical activity within the equine heart and its orientation within the thorax, cardiac rhythm is often evaluated in lead 1 using a base‐apex orientation, as opposed to the frontal plane orientation in humans and small animals. This requires placement of the left forelimb electrode (black) at the level of the apex on the left side of the thorax, with the right forelimb electrode (white) at the top of the right scapular spine, and the left hind limb (red) electrode placed at variable locations distant to the heart. These differences result in equine ECG morphology that has unique characteristics that differ from small animals. Specifically, the P wave may be simple positive, bifid, or biphasic, with a predominantly negative deflection of the QRS complex, and a T wave that is variable in size and orientation (Figure 5.1). Cardiac output is not routinely measured in clinical equine patients due to expense, practicality, and potential invasiveness, but provides a better measure of hemodynamic status than heart rate, blood pressure, and hematologic values. Various cardiac output measurement techniques have been evaluated in horses and foals. Indicator dilution techniques include transcardiac or transpulmonary thermodilution, lithium dilution, and ultrasound dilution techniques. Dilution techniques require the administration of an indicator substance into the venous circulation or right atrium and subsequent measurement of the indicator substance distal to the heart to determine flow based on versions of the Stewart‐Hamilton equation. These methods are intermittent and the most invasive, but are considered the most accurate in equine patients (Linton et al. 2000, Shih et al. 2009a). Both thermodilution and lithium dilution techniques can be used to calibrate pulse waveform analysis methods, which provide a beat‐by‐beat determination of cardiac output (Shih et al. 2009a,b). The indirect Fick principle can also be used to determine cardiac output in foals non‐invasively using the partial carbon dioxide rebreathing method (Valverde et al. 2007). While this technique is limited to use in foals and very small equine patients, it correlates well with lithium dilution measurements and provides a clinically practical option for cardiac output measurement. Imaging techniques, such as magnetic resonance imaging (MRI) and echocardiography are other possible techniques for evaluating cardiac output in equine patients, but require expertise in interpretation (Young et al. 1996). More comprehensive reviews of cardiac output monitoring techniques in equine patients have been previously published (Corley et al. 2003; Shih 2013). While capnography is not a substitute for cardiac output monitoring, changes in end‐tidal CO2 during stable ventilation may be reflective of changes in pulmonary perfusion and thus cardiac output, and can be helpful to trend during periods of cardiovascular change. Figure 5.1 Normal ECG of the horse. Systemic blood pressure is not a surrogate measure of tissue perfusion; however, hypotension can reflect low perfusion states and is associated with an increased risk of myopathy and other potential complications (Grandy et al. 1987; Johnston et al. 2002). Blood pressure monitoring is particularly important in horses undergoing inhalational anesthesia, in hemodynamically unstable patients, and in those at risk of cardiovascular complications. By identifying hypotension early, appropriate cardiovascular supportive therapies can be implemented with the goal of minimizing morbidity and mortality. The primary factors that determine arterial blood pressure are cardiac output and SVR. Cardiac output is determined by heart rate and stroke volume, while stroke volume is determined by preload, afterload, and contractility. Thus, hypotension may be due to bradycardia, decreased vascular tone, decreased preload, poor contractility, or a combination of these factors. Hypotension is often defined as a mean arterial blood pressure below 60 mmHg in anesthetized dogs (Ruffato et al. 2015). While there is no such consensus reported in equine patients, maintaining a mean arterial blood pressure of at least 70 mmHg reduces the incidence of myopathy in mature halothane‐anesthetized horses, with neonatal foals generally tolerating lower blood pressures (Grandy et al. 1987). Treatment should be directed at correcting the underlying cause of hypotension. Bradycardia, typically defined as a heart rate below 26 beats/minute in mature horses, is a common finding in horses that are physically fit and following administration of alpha‐2 adrenergic agonists. While anticholinergics effectively elevate heart rate, their administration to hypotensive and bradycardic equine patients should be considered carefully due to resulting reductions in gastrointestinal motility, but is often warranted with heart rates below 20 beats/minute. Reductions in vascular tone can often be improved by decreasing the end‐tidal concentration of inhalational anesthetic administered, which may require the use of partial or total intravenous anesthetic techniques to maintain appropriate anesthetic depth. Vasoactive agents, such as phenylephrine, can also be administered with the goal of improving vascular tone; however, they should be used judiciously as excessive increases in vascular tone may increase blood pressure at the expense of tissue perfusion (Dancker et al. 2018). A reduction in preload due to deficits in circulating volume or inadequate venous return is a common source of hypotension in horses undergoing anesthesia for colic surgery and other emergent procedures. Correcting volume deficits prior to anesthesia is ideal, but not always possible. Pre‐existing deficits and ongoing fluid losses should be replaced with a balanced electrolyte solution, with or without the addition of hypertonic saline, synthetic colloids, or blood products depending on the nature and severity of fluid losses, and the presence of other systemic diseases. In some cases of abdominal distention, dorsal recumbency will result in caval compression and dramatically reduce venous return. While fluid therapy can help improve preload in these instances, the most immediately effective treatment is often a change in position or abdominal decompression. Myocardial depression and compromised contractility can be caused by a number of factors, including the administration of inhalational anesthetics. Positive inotropes can effectively improve contractility and elevate blood pressure. Dobutamine is commonly infused to increase contractility in anesthetized horses due to its selectively for beta receptors. The slow infusion of calcium is also indicated to improve contractility if ionized calcium is marginal or low. Other agents with inotropic activity, such as dopamine, norepinephrine, and ephedrine, affect both contractility and vascular tone, and can be used when both are compromised (Dancker et al. 2018). The ductus arteriosus is an essential part of normal fetal circulation between the aorta and pulmonary artery. Persistence of the ductus arteriosus after birth with left‐to‐right shunting causes volume overload of the left atrium and ventricle, which leads to remodeling in the form of eccentric hypertrophy (dilatation) predisposing patients to the development of congestive HF (Buchanan 2001). Patent ductus arteriosus (PDA) occurs infrequently in horses, either alone or in combination with other artery defects (Huston et al. 1977). Auscultation findings in a foal with a PDA reveal a loud, continuous, left basilar murmur, and hyperkinetic arterial pulse quality with a rapid rise and decline (Fregin 1982). In most foals, physiologic closure of the PDA occurs within 16 hours post‐partum; however, trivial flow through the PDA has been identified in foals up to a week of age and rarely in adult horses (Hare 1931). Echocardiography can be used to definitively diagnose a PDA and assess the severity of myocardial changes. Foals with PDAs may develop signs of congestive HF within the first few weeks post‐partum or may reach maturity before signs develop. Rarely, PDAs can result in pulmonary hypertension resulting in a reversal of flow through the PDA causing systemic hypoxia. Primary closure of a PDA in a horse has not been reported (Figure 5.2). Figure 5.2 Echocardiographic image of a PDA in foal. Anesthetic considerations for foals with a PDA vary depending on the size of the communication as well as the presence of other congenital abnormalities and secondary cardiac changes. The majority of foals undergoing anesthesia in the first week of life will have a PDA in varying states of closure. In healthy neonatal foals, there is a degree of hemodynamic compromise under isoflurane anesthesia, characterized by a decrease in heart rate and cardiac index, with minimal changes in pulmonary and SVR, and only mildly reduced blood pressure compared with the conscious state (Lombard et al. 1984; Craig et al. 2007). These changes are expected due to age‐related cardiovascular physiology alone, and the contribution of PDA on cardiovascular performance is unquantified. Regardless, cardiovascular sparing protocols should still be selected in neonatal foals, with benzodiazepines providing better hemodynamic performance than alpha‐2 agonists, particularly in foals under two weeks of age (Kerr et al. 2009). Pathological or persistent PDA rarely occurs as a sole condition in foals, and is typically part of a complex congenital defect. When persistent PDA presents in isolation, anesthetic goals should include: maintaining heart rate in upper normal range, maintaining vascular tone and avoiding decreases in blood pressure (particularly diastolic), preserving or increasing contractility with dobutamine or other positive inotropes depending on the presence of HF or hypotension, and maintaining sufficient preload without volume excess. Alpha‐2 agonists should be avoided when typical left‐to‐right shunting is present within the PDA as the increase in afterload will increase the fraction of blood diverted from systemic circulation, and bradycardia will further compromise cardiac output. Acepromazine and high concentrations of inhalational anesthetics reduce SVR, and in the presence of high PVR, have the potential to create or worsen right‐to‐left shunting in addition to increasing the incidence of hypotension. Benzodiazepines and opioids are ideal agents for premedication in foals with PDA resulting in few cardiovascular changes. Induction agents should be titrated carefully to minimize any unwanted cardiovascular effects, and include ketamine used alone or in combination with propofol or alfaxalone. While the cardiovascular and endocrine effects of etomidate have not yet been evaluated in foals, the cardiovascular stability provided by this agent may justify its use in foals with HF despite reductions in steroidogenesis. Maintenance of anesthesia should focus on minimizing inhalational anesthetic requirements. Total intravenous anesthesia using propofol and opioid infusions has been used during canine PDA surgery (Musk and Flaherty 2007), and may also be a suitable option in foals; however, it is unknown if this approach offers cardiovascular advantages in foals with PDA. Supportive therapy should be provided as needed, including judicious manual ventilation and fluid therapy, as well as the administration of positive inotropes and vasoactive drugs in order to optimize cardiovascular performance. Atrial septation is a complex process occurring during embryologic development of the fetus (Kittleson and Kienle 1998). If septation is interrupted, defects within the atrial septum can arise. The size and location of the defect can vary, and, with it, the degree and direction of shunting. In the absence of right heart pressure overload, flow through an atrial septal defect (ASD) will move from the left atrium to the right atrium. Subsequently, extra blood volume will pass through the right ventricle and pulmonary circulation resulting in right ventricular dilatation and increased hydrostatic pressure in the pulmonary vasculature. Foals with a large ASD are at risk for right‐sided HF, pulmonary edema, and pulmonary hypertension. A large ASD can create an audible, left basilar, systolic heart murmur associated with physiologically increased pulmonic flow velocities. Most ASDs in foals are clinically insignificant, although atrial fibrillation (AF) and progressive HF has been reported (Taylor et al. 1991
5
Anesthetic Management for Interventional Cardiac Procedures
Introduction
Cardiovascular Physiology
Hemodynamic Variables in Normal, Standing Horses
Variable
Value
Unit
Heart rate
26–50
beats/min
Central venous pressure
5–10
mmHg
RV end‐diastolic pressure
10–20
mmHg
RV end‐systolic pressure
40–60
mmHg
Pulmonary artery systolic pressure
35–45
mmHg
Mean pulmonary artery pressure
25–30
mmHg
Pulmonary artery diastolic pressure
20–25
mmHg
Pulmonary capillary wedge pressure
13–15
mmHg
LV end‐diastolic pressure
12–24
mmHg
LV end‐systolic pressure
110–130
mmHg
Cardiac output
30–40
l/min
Cardiac index
60–80
ml/min/kg
Cardiovascular Effects of Common Anesthetic, Analgesic, and Sedative Agents
Alpha‐2 Adrenergic Agonists
Acepromazine
Opioids
Benzodiazepines
Guaifenesin (GG)
Ketamine
Propofol
Lidocaine
Inhalant Anesthetics
Pre‐anesthetic Cardiac Evaluation
Physical Examination
Electrocardiogram
Echocardiogram
Cardiovascular Monitoring
Blood Pressure
ECG
Cardiac Output
Hypotension
Common Drugs Used to Treat Hypotension in Anesthetized Equine Patients
Drug
Mechanism of action
Dose
Possible adverse effects
Atropine
Muscarinic receptor antagonist
0.01–0.02 mg/kg IV
Tachycardia
Reduced GI motility
Glycopyrrolate
Muscarinic receptor antagonist
0.002–0.005 mg/kg IV
Tachycardia
Reduced GI motility
23% Calcium gluconate
Increases intracellular calcium in cardiomyocytes
0.2–0.4 ml/kg IV over 15 minutes
Bradycardiawhen administered rapidly IV
Dobutamine
Beta receptor agonist
0.5–5 ug/kg/min IV
Brady‐ or tachycardia
Atrial or ventricular arrhythmias
Dopamine
Alpha, beta, and dopamine receptor agonist
0.5–5 ug/kg/min IV
Tachycardia
Atrial or ventricular arrhythmias
Norepinephrine
Alpha and beta receptor agonist
0.1–1.0 ug/kg/min IV
Atrioventricular block
Possible atrial or ventricular arrhythmias
Ephedrine
Direct beta receptoragonist,
stimulates norepinephrine release from nerve terminals for alpha and beta stimulation
0.02–0.1 mg/kgIV
Variable change inheart rate, tachyphylaxis
Congenital Cardiac Conditions in Horses
Patent Ductus Arteriosus (PDA)
Pathophysiology
Anesthetic Considerations and Management
Atrial Septal Defects (ASD)
Pathophysiology
Stay updated, free articles. Join our Telegram channel

Full access? Get Clinical Tree
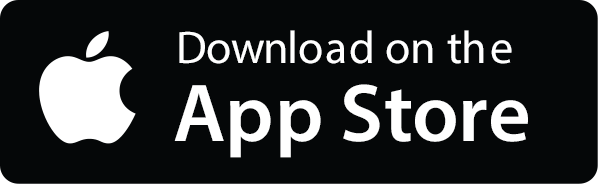
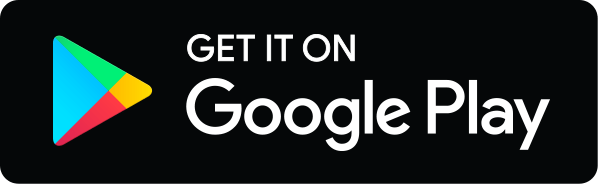