Introduction
As the science of veterinary medicine advances life-prolonging therapies, clinicians will be exposed to increasing numbers of older patients that will require care. Along with the advancing age of the patients, the prevalence of diseases associated with advanced age will increase. The kidney is an organ commonly associated with disease (i.e., chronic kidney disease) in geriatric patients. In fact, up to 20% of cats will be affected with chronic kidney disease during their lifetime.1
In cats greater than 15years of age, 31% have evidence of renal disease.1 Although the total prevalence of renal disease in dogs is less than cats, advanced age plays a role in this species as well. Of dogs with renal failure, 45% are older than 10years of age.2 It is therefore reasonable to assume that a veterinary practitioner will anesthetize animals with renal diseases in the regular course of daily practice. It is imperative that the veterinarian providing anesthesia have an understanding of renal physiology, renal pathophysiology, and the effects of sedative, analgesic, and anesthetic drugs on the kidney.
The kidneys have three primary functions: filtration, reabsorption, and secretion. To accomplish these functions, they receive about 25% of the cardiac output. The renal tubules and collecting ducts reabsorb up to 99% of filtered solutes, indicating that the total filtration volume is much greater than daily urine production. Neurohumoral substances and physiological factors that affect reabsorption of the filtered water and sodium include aldosterone, antidiuretic hormone (ADH), arterial blood pressure (ABP), atrial natriuretic factor, catecholamines, prostaglandins, renin-angiotensin, and stress.
Renal blood flow (RBF) is regulated by extrinsic nervous and hormonal control and by intrinsic autoregulation. Renal vasculature is highly innervated by sympathetic constrictor fibers originating in the spinal cord segments between T4 and L1. The kidneys lack sympathetic vasodilating fibers and parasympathetic innervation. Intrinsic autoregulation of RBF is demonstrated by a constant flow when mean ABP ranges from 80 to 180 mm Hg. When the mean ABP is between 80 and 180 mm Hg, the kidney can control blood flow by altering resistance in the glomerular afferent arterioles. Although the exact mechanism of renal autoregulation is not known, the significance of this phenomenon relates to protection of glomerular capillaries during hypertension and preservation of renal function during hypotension. However, even within the range of blood pressure described for function of renal autoregulation, extrinsic forces (e.g., neural, hormonal, and pharmacological) and intrinsic forces (e.g., renal insufficiency/failure) may cause alterations in RBF and glomerular filtration rate (GFR).
Catecholamines are the major hormonal regulators of RBF. Epinephrine and norepinephrine cause dose-dependent changes in RBF and the GFR. Low doses increase ABP and decrease RBF with no net change in the GFR. Higher doses cause decreased RBF and GFR. The renal vascular anatomy is unique in its distribution to cortical and medullary zones. Because of this vascular dichotomy, local tissue ischemia and hypoxia may occur even though total organ blood flow is normal. Oxygen delivery to the kidney is complex, and selective regional hypoxia is a possible source of renal injury during renal hypoperfusion. Experimental evidence indicates that the medullary thick ascending limb of Henle’s loop, because of its high metabolic rate associated with active transport of electrolytes, is selectively vulnerable to hypoxic injury.3
The pathophysiology of the kidney can be divided into acute and chronic renal failure. Acute renal failure is determined by a rapid onset of a decrease in GFR over a period of hours to days that manifests as an inability to excrete wastes (i.e., blood urea nitrogen [BUN] and creatinine) and maintain fluid and electrolyte homeostasis.4 Failure of the sodium–potassium ATPase pump in the renal tubules during acute tubular necrosis is the most common cause of acute renal failure with interstitial nephritis and acute glomerulonephritis comprising the other causes.5 Acute renal failure can be further classified into prerenal azotemia, intrinsic renal failure, and postrenal obstructive nephropathy.5 A thorough history, signalment, physical exam, serum chemistry panel, and urinalysis can be used to help differentiate between the three classes and direct treatments.
Chronic renal failure can be defined as the progressive loss of nephron function and decreased GFR over a prolonged period of time.4 Only when 60% or greater of the total number of nephrons become nonfunctional will clinical and/or laboratory signs of renal insufficiency become evident. At this point, the remaining functional renal mass is no longer able to appropriately eliminate waste, and nitrogenous compounds accumulate. The ability to concentrate filtrate and conserve free water is lost and patients may enter a state of hypovolemia. Additionally, the ability to balance electrolytes may be lost with derangements, eventually becoming severe enough to threaten physiological function of other organ systems (i.e., cardiovascular).
Patients with chronic renal failure may be anemic because of bone marrow suppression, chronic gastrointestinal tract blood loss, reduced red blood cell life span, and decreased secretion of enzymes that are responsible for the formation of erythropoietin with a resulting understimulation of bone marrow. In response to anemia, the cardiovascular system may become hyperdynamic in an attempt to maintain oxygen delivery. Chronic renal disease may therefore be associated with hypertension and increased cardiac output but reduced cardiac reserve.
Anesthetic effects on renal function
Effects of anesthetics on RBF can be summarized with the following generalization: All anesthetics are likely to decrease the rate of glomerular filtration. Anesthetics may directly affect RBF, or they may indirectly alter renal function via changes in cardiovascular and/or neuroendocrine activity. Most anesthetics decrease the GFR as a consequence of decreased RBF (Table 14.1). Anesthetics that cause catecholamine release (e.g., ketamine, tiletamine, and nitrous oxide) have variable effects on RBF.
Sedative and analgesic drugs have varying effects on RBF and GFR and generally relate to individual drug effects on cardiac output and vasomotor tone. Phenothiazine tranquilizers (e.g., acepromazine) produce dose-dependent hypotension through blockade of vascular alpha adrenergic receptors. Phenothiazines also antagonize dopamine receptors. Dopamine receptor blockade by acepromazine premedication may prevent dopamine-induced increases in RBF during surgery. However, RBF and GFR do not change significantly even in the face of mild hypotension and may impart protection of renal function after low-dose acepromazine administration.6 The use of opioids in patients with renal disease has been recommended.7 Opioids will provide sedation and analgesia to patients with minimal impact on cardiac output and thus RBF.8However, clinicians should be cognizant of the fact that opioids can cause urine retention when administered systemically or as an epidural injection. Alpha2 agonist drugs such as dexmedetomidine and xylazine are well known to have significant dose-dependent depressant effects on heart rate and cardiac output, and increase systemic vascular resistance. Additionally, alpha2 agonist drugs have a diuretic effect through antidiuretic antagonism and can increase urine volume that may be detrimental in patients with postrenal urinary tract obstruction.9 It has been recommended that use of this class of drugs should be avoided in patients with renal disease.7
Table 14.1. Effects of anesthetics on renal blood flow (RBF) and glomerular filtration rate (GFR)
Source: Adapted from Greene S.A., Grauer G.F. 2007. Renal disease. In: Lumb and Jones’ Veterinary Anesthesia and Analgesia, 4th ed. W.J. Tranquilli, J.C. Thurmon, and K.A. Grimm, eds. Ames, IA: Blackwell, pp. 915–919.
Drug | RBF | GFR |
Isoflurane | Slight decrease | Decrease |
Sevoflurane | Slight decrease | Decrease |
Thiopental | No change | No change or slight decrease |
Ketamine | Increase | Decrease or no change |
Propofol | No change | No change |
Etomidate | No change | No change |
Injectable anesthetic agents can also have an effect on renal parameters. Thiobarbiturates increase systemic vascular resistance but decrease renal vascular resistance with no net change in RBF. In contrast, ketamine increases RBF and renal vascular resistance.10 However, ketamine administration may result in uneven distribution of blood flow within the kidney. Ketamine is also partially dependent on renal excretion in cats and therefore should be avoided, or used carefully in cats with renal insufficiency.11 Propofol at moderate to low doses has minimal effect on RBF and GFR and is often used for induction of anesthesia in renal patients.12,13 Etomidate is an anesthetic agent known for its minimal effects on heart rate, blood pressure, and cardiac output. Etomidate has also been shown to have no significant effect on renal function and urine output in anesthetized rats.14
Inhalant anesthetics cause systemic hypotension, especially during excessive depth, which can result in renal ischemia. This is a result of one of the major side effects of potent volatile anesthetics, peripheral vasodilation. Inhalant anesthetics also depress myocardial contractility and cardiac output in a dose-dependent manner. Concurrently, inhalation anesthetics tend to decrease RBF and GFR in a dose-dependent manner as well. Light planes of inhalation anesthesia preserve renal autoregulation of blood flow, whereas deep planes are associated with depression of autoregulation and decreases in RBF. Although isoflurane has little effect on RBF, it decreases GFR and urine output.15 Sevoflurane, although not well studied, seems to have similar effects on RBF when compared to isoflurane.16 However, when in contact with carbon dioxide absorbents, sevoflurane degrades to a nephrotoxic substance called compound A. Compound A has been shown to cause permanent damage to the kidneys of rats but has not been shown to cause problems in humans with renal insufficiency or in dogs with normal renal function.17,18 Desflurane has no effect on RBF at concentrations up to twice the minimal alveolar concentration (MAC); however, it decreases renal vascular resistance at concentrations greater than 1.75 MAC.19 For most patients, the effects of inhaled anesthetics on renal function are reversed at the termination of anesthesia. Some patients, however, may not regain the ability to regulate urine production for several days.20 Any patient that demonstrates postanesthetic oliguria should be evaluated immediately for renal insufficiency/failure.
Most anesthetics, whether injectable or inhalant, cause less disruption of renal autoregulation of blood flow at lower doses (lighter anesthetic planes). Renal responses to anesthetics also depend on the preexisting hydration status and quantity of perioperative fluids administered, as well as preexisting renal disease.
Anesthesia and the stress associated with surgery can cause release of aldosterone, vasopressin, renin, and catecholamines. Accordingly, RBF and GFR (and therefore urine production) are generally decreased with surgery in any patient. In fact, in the face of appropriate intravenous (IV) fluid administration during (10mL/kg/h) anesthesia, dogs with normal kidney function will have urine output less than the usual values of 1–2 mL/kg/h of awake animals.21 Additionally, these dogs will have evidence of fluid retention that resolves over time after anesthesia. It is therefore recommended to use additional parameters rather than just urine output as an indicator of fluid balance and renal function in anesthetized animals.21
Effects of renal disease on anesthesia
Renal insufficiency/failure and azotemia in patients with renal insufficiency can alter the individual patient’s response to anesthetic medications. Azotemia can alter the blood–brain barrier, leading to increased drug penetration into the central nervous system. Azotemia can also alter the binding of drugs to carrier proteins and receptors, resulting in increased levels of circulating free drug and an increase or decrease in the expected response of the patient to drug administration. Patients with renal insufficiency/failure may be acidotic, which can lead to increased fractions of unbound injectable drugs in the plasma, thus having a similar effect as azotemia. It may be necessary to decrease the doses of highly protein-bound injectable anesthetics in azotemic and/or acidotic patients.
Serum electrolyte imbalance is common in patients with renal disease. Hyperkalemia may be present in animals with renal insufficiency/failure, obstructed urethra, or rupture of the urinary bladder and can be extremely dangerous. In general, patients presenting for nonemergency anesthesia and surgery should not be anesthetized if they have a serum potassium concentration greater than 5.5mEq/L. Any patient with a serum potassium concentration greater than 6.0 mEq/L should not be anesthetized until the hyperkalemia can be addressed. Electrocardiographic (ECG) abnormalities are commonly observed with potassium concentrations exceeding 7 mEq/L and during the stress of anesthesia patients with renal disease can quickly elevate their serum potassium concentrations. The resting membrane potential of cardiac muscle depends on the permeability and extracellular concentration of potassium. During hyperkalemia, the membrane’s resting potential is raised (partially depolarized), and fewer sodium channels are available to participate in the action potential. As the serum potassium concentration increases, repolarization occurs more rapidly and automaticity, conductivity, contractility, and excitability are decreased. These changes produce the classic ECG appearance of a peaked T wave with a prolonged PR interval progressing to wide QRS complexes and loss of P waves. In cases of prolonged renal disease and chronic hyperkalemia, serum potassium should be lowered gradually to allow intracellular potassium time to reestablish physiological transmembrane concentration gradients. If hyperkalemia is acute or ECG abnormalities are noted, treatment should be initiated prior to induction of anesthesia. The most rapid treatment for the cardiac effects associated with hyperkalemia is 10% calcium chloride (0.1mg/kg IV). Calcium will increase the membrane’s threshold potential, resulting in increased myocardial conduction and contractility. Because increased serum potassium concentration causes the resting potential to be less negative (partially depolarized), the calcium ion-induced increase in threshold potential temporarily restores the normal gradient between resting and threshold potentials. It should be recognized that administration of calcium will not affect the serum potassium concentration, and its effects will therefore be short lived. Regimens to decrease the serum potassium concentration by shifting potassium intracellularly include infusion of 2.5–5% dextrose solutions and, in severe cases, addition of insulin to the dextrose. Often, acidosis is associated with the concurrent increase in serum potassium. Sodium bicarbonate can be administered to enhance hydrogen ion exchange with intracellular potassium. Additionally, during anesthesia, because acidemia favors extracellular movement of potassium and worsens hyperkalemia, intermittent positive pressure ventilation may be required to prevent anesthetic-induced hypercapnia and respiratory acidosis. Patients in renal failure with hypocalcemia are at even greater risk of the arrhythmic effects of hyperkalemia, because hypocalcemia potentiates the myocardial toxicity of hyperkalemia.
Anesthetic management of patients with renal disease
Patients with suspected or known renal disease should have a thorough workup prior to any anesthetic event. This should include a physical exam, complete blood count, serum biochemical profile, and urinalysis. Measurements of the GFR and renal tubular function, such as urine specific gravity and BUN, are not specific for renal disease. Serum creatinine is a more specific indicator of the GFR than BUN because it is influenced by fewer extrarenal variables. Patients with mild renal insufficiency may not have elevated serum creatinine, thus it is important to carefully evaluate other parameters that can be affected. In addition to azotemia, effects of renal system dysfunction can manifest as abnormalities in acid–base balance, electrolyte concentrations (especially potassium), exercise intolerance, hematocrit, hydration status, and urine production. Persistent proteinuria and/or cellular or granular cylinduria may indicate renal damage prior to the onset of renal azotemia.
Preanesthetic stabilization of patients with renal disease may be more critical to a successful outcome than the specific anesthetic drugs selected. By and large, the most important parameter for a patient with renal disease is hydration status and circulating blood volume. Maintaining RBF and GFR through adequate hydration will reduce the likelihood of further renal injury and preserve renal function.22 Hydration has been shown to be effective in the treatment of renal injury and is a good strategy to prevent the progression of renal insufficiency to frank renal failure.22 A patient can be admitted 12–24 hours prior to anesthesia and administered IV fluids to achieve hydration and diuresis. When renal function is questioned, the urinary bladder can be catheterized and urine production monitored via a closed, sterile urine collection system. Urine production is an indirect measure of renal perfusion, and normal urine output for awake dogs is 0.5–2.0mL/kg/h. Animals administered high rates of IV fluids should have urine outputs similar to the amount of fluids administered. Fluid therapy prior to anesthesia can also be used to correct electrolyte and acid–base imbalances. Anemic patients undergoing anesthesia should have a red blood cell transfusion if the hematocrit is less than 18% (cats) or 20% (dogs).
Once the patient is stabilized, anesthesia can be performed. Anesthetic and analgesic drugs that have minimal effects on cardiac output, blood pressure, and perfusion are recommended.7 Premedication can be achieved with an opioid/benzodiazepine combination and induction of anesthesia can be accomplished with propofol, thiopental, etomidate, diazepam–ketamine, or diazepam–opioid combinations (Table 14.2). It is important to remember that all of these anesthetic drugs can cause some degree of reduced RBF and/or GFR and that using these drugs to effect is recommended. Anesthesia can be maintained with either isoflurane or sevoflurane.
Continuing IV fluid therapy throughout the anesthetic period is recommended to maintain fluid volume and hydration. Initially, a rate of up to 20 mL/kg for the first hour can be provided and thereafter a rate of 10/mL/kg/h can be used. Lower fluid rates can be used if the patient has hypoproteinemia, severe anemia, or cardiovascular disease. The choice of IV fluid is based on the animal’s electrolyte and acid–base status. In general, animals with mild to moderate renal insufficiency/failure that are well prepared for surgery or anesthesia are given lactated Ringer’s solution. If there is potential for urinary tract obstruction or the patient is anuric, IV fluids should be used cautiously to prevent fluid overload until the obstruction can be addressed.
Table 14.2. Example of an anesthetic plan for patients with renal disease
Premedication: | ||
Opioid of choice | ||
Butorphanol | 0.2–0.4 mg/kg | Intramuscular |
Hydromorphone | 0.1 mg/kg | Intramuscular |
Morphine | 0.1 mg/kg (cats) | Intramuscular |
0.25 mg/kg (dogs) | Intramuscular | |
Midazolama | 0.2–0.4 mg/kg | Intramuscular |
Induction: | ||
Propofol | 4 mg/kg (to effect) | IV |
Etomidate | 2 mg/kg (to effect) | IV |
Maintenance: | ||
Isoflurane | 1–2% (to effect) | Inhalation |
Sevoflurane | 2–3% (to effect) | Inhalation |
Supportive treatments: | ||
Lactated Ringer’s solution | 10–20 mL/kg for first hour, 10 mL/kg/h thereafter | |
Mannitol (20% solution) | Loading dose 500 mg/kg IV Infusion 1 mg/kg/min |
a I n healthy cats, midazolam may elicit aggressive behavior and can be removed from plan.
Vigilant monitoring of a patient’s vital signs will help the anesthetist to identify hypotension, arrhythmias, hypoxemia, or hypoventilation that could negatively impact renal function. Continuous electrocardiography (ECG) can detect changes in cardiac electrical activity that can be associated with electrolyte abnormalities such as hyperkalemia. ABP should be measured to detect systemic hypotension and decreased renal perfusion pressure. Mean ABP should be maintained above 70–80mmHg, and in some cases higher if preanesthetic hypertension was present. Pulse oximetry (SpO2) can be used to rapidly detect hemoglobin desaturation and alert the anesthetist to the potential for a decrease in tissue oxygen delivery. Continuous end-tidal carbon dioxide (ETCO2) measurement can be used to detect hypoventilation and the need for assisted ventilation. Excessive arterial carbon dioxide can lead to acidemia, which can worsen renal disease. Periodic arterial blood gas analysis can be useful for following trends in pH, oxygen content, and electrolytes. Finally, central venous pressure (CVP) can be measured via a jugular catheter as an indirect measurement of blood volume to evaluate the rate of IV fluid administration. Normal CVP should be between 3 and 5 cm H2O. If the CVP rises to more than 10 cm H2O, fluid administration should be slowed or stopped. If the CVP falls in response to the fluids being stopped, they may be resumed at a slower rate. An elevated CVP of more than 10 cm H2O suggests inadequate myocardial function or volume overload.
Adjunctive treatments for patients with renal disease
During the perianesthetic period, pharmacological manipulation of cardiovascular and renal physiology may be beneficial in renal disease patients. Dopamine infusions (1–10 mcg/kg/min) have long been considered useful in improving myocardial function and cardiac output. In human patients with renal disease, renal doses of dopamine (2mcg/kg/min) have been shown to increase urinary output but does not improve overall outcome when compared to IV fluid therapy.23 In dogs, low doses (1–3 mcg/kg/min) are used to promote RBF and GFR, but studies showing benefit are lacking. Controversy exists as to the use of dopamine to improve renal function in cats. Questions remain as to whether or not cats have appropriate dopamine receptors in their kidneys and low-dose dopamine has not been shown to have a diuretic effect in cats.24 Doses of dopamine above approximately 10 mcg/kg/min may cause α-adrenergic renal vasoconstriction and decreased RBF and should be avoided.
Furosemide has also been investigated as to its use during anesthesia in patients with renal dysfunction. As a loop diuretic, furosemide decreases the metabolic activity of the renal tubules; however, furosemide infusion has been shown to result in elevated creatinine levels in anesthetized human patients and its use is not recommended.23,25
The osmotic diuretic mannitol has many potentially beneficial effects on the kidney. Mannitol is freely filtered and non-reabsorbed by the kidney and therefore acts as an osmotic agent in the renal tubules as well as in the systemic circulation. Administration of mannitol can induce renal arteriole dilation, decrease vascular resistance and blood viscosity, and scavenge oxygen free radicals.26 RBF in cats may be improved by administering an IV loading dose of mannitol (500 mg/kg) and continuing a constant rate infusion (1 mg/kg/min) during the anesthetic period.27 The author uses mannitol infusions in both cats and dogs with known or suspected renal impairment during anesthesia.
Fenoldopam is a dopamine receptor agonist at the DA1 receptor that has renal vasodilating properties. Fenoldopam has no effect on DA2 or alpha receptors that can cause vasoconstriction and result in decreased RBF and GFR. In fact, fenoldopam increases RBF and may assist in preserving renal function. Fenoldopam has been shown to decrease creatinine and improve renal function at a dose of 0.1 mcg/kg/min when compared to dopamine infusion and may be effective in decreasing renal hypoperfusion.28
Postanesthetic care of any patient that has had a procedure associated with a painful condition should be treated with analgesic medications. In renal patients, analgesic drugs, including nonsteroidal anti-inflammatory drugs (NSAIDs), that are potentially nephrotoxic should be avoided. Pain can usually be managed with opioid analgesics.
Management of patients with post-renal disease
Patients with urethral obstruction often present with metabolic derangements. These include hyperkalemia, azotemia, acidosis, and hyperphosphatemia. Cats may also develop hyperglycemia, hypocalcemia, and hyponatremia. If the disease condition included rupture of the urinary bladder, the animal may develop more severe electrolyte abnormalities and acidosis. In these cases, potassium enters the abdominal cavity from the ruptured bladder and is reabsorbed into the circulation, causing an increased serum potassium concentration. It is important to recognize that acute urethral obstruction and ruptured bladder are medical emergencies and that any metabolic abnormalities should be evaluated and addressed prior to anesthesia and surgical correction. Hyperkalemia is the primary concern in most cases of urethral obstruction, and an electrocardiogram assessment is warranted. Treatment of hyperkalemia has been discussed earlier.
In animals with urethral obstruction, fine-needle centesis of the urinary bladder may be performed prior to anesthesia, although bladder injury is a potential concern. In animals with uroperitoneum, abdominocentesis can be performed. Removal of urine can help reduce the potassium load and relieve patient discomfort. Once the patient is stabilized, anesthesia can be induced by the previously described techniques. IV ketamine with a benzodiazepine has been used in obstructed cats even though active metabolites of the drug are excreted by the kidney. The rationale is that, once the obstruction is relieved, excretion of the anesthetic will proceed normally. However, cats with a long-term urethral obstruction may develop metabolic disturbances and renal insufficiency such that elimination of drugs is slowed even after the obstruction has been removed. As with other patients with renal disease, IV fluid therapy should be continued throughout the anesthetic period and into the postanesthetic period until ongoing acid-base and electrolyte disturbances are corrected.
Revised from “Renal Disease” by Stephen A. Greene and Gregory F. Grauer in Lumb and Jones’ Veterinary Anesthesia and Analgesia, Fourth Edition.
1. Boyd L.M., Langston C., Thompson K., et al. Survival in cats with naturally occurring chronic kidney disease (2000–2002). J Vetlntern Med 22: 1111–1117, 2008.
2. Polzin D.J., Osborne C.A., Jacob F., et al. Chronic renal failure. In: Textbook of Veterinary Internal Medicine, 5th ed. S.J. Ettinger and E.C. Feldman, eds. Philadelphia, PA: W.B. Saunders Company, 2000, pp 1634–1662.
3. Brezis M., Rosen S. Hypoxia of the renal medulla: its implications for disease. N Engl J Med 332(10): 647–655, 1995.
4. Stoelting R.K., Hillier S.C. Kidneys. In: Pharmacology & Physiology in Anesthetic Practice, 4th ed. Philadelphia, PA: Lippincott Williams & Wilkins, 2006, pp 817–830.
5. Thadhani R., Pascual M., Bonventre J.V. Acute renal failure. N Engl J Med 334: 1448–1460, 1996.
6. Bostrom I., Nyman G., Kampa N., et al. Effects of acepromazine on renal function in anesthetized dogs. Am J Vet Res 64(5): 590–598, 2003.
7. Weil A.B. Anesthesia for patients with renal/hepatic disease. Top Companion Anim Med 25(2): 87–91, 2010.
8. Lamont L.A., Mathews K.A. Opioids, nonsteroidal antiinflammatories, and analgesic adjuvants. In: Lumb & Jones’ Veterinary Anesthesia and Analgesia, 4th ed. W.J. Tranquilli, J.C. Thurmon, and K.A. Grimm, eds. Ames, IA: Blackwell Publishing, 2007, pp 241–271.
9. Saleh N., Aoki M., Shimada T., et al. Renal effects of medetomidine in isoflurane-anesthetized dogs with special reference to its diuretic action. J Vet Med Sci 67(5): 461–465, 2005.
10. Priano L.L. Alteration of renal hemodynamics by thiopental, diazepam, and ketamine in conscious dogs. Anesth Analg 61(10): 853–862, 1982.
11. Flecknell P.A. Injectable anaesthetics. In: Anaesthesia ofthe Cat. L.W. Hall and P.M. Taylor, eds. London, UK: Bailliere Tindall, 1994, pp 129–156.
12. Wouters P.F., Van de Velde M.A., Marcus M.A., et al. Hemodynamic changes during induction of anesthesia with eltanolone and propofol in dogs. Anesth Analg 81(1): 125–131, 1995.
13. Shiga Y., Minami K., Uezono Y., et al. Effects of the intravenously administered anaesthetics ketamine, propofol, and thiamylal on the cortical renal blood flow in rats. Pharmacology 68(1): 17–23, 2003.
14. Petersen J.S., Shalmi M., Christensen S., et al. Comparison of the renal effects of six sedating agents in rats. Physiol Behav 60(3): 759–765, 1996.
15. Gelman S., Fowler K.C., Smith L.R. Regional blood flow during isoflurane and halothane anesthesia. Anesth Analg 63(6): 557–565, 1984.
16. Bernard J.M., Doursout M.F., Wouters P., et al. Effects of sevoflurane and soflurane on hepatic circulation in the chronically instrumented dog. Anesthesiology 77(3): 541–545, 1992.
17. Conzen P.F., Kharasch E.D., Czerner S.F.A., et al. Low-flow sevoflurane compared with low-flow isoflurane anesthesia in patients with stable renal insufficiency. Anesthesiology 97: 578–584, 2002.
18. Sun L., Suzuki Y., Takata M., et al. Repeated low-flow sevoflurane anesthesia: effects on hepatic and renal function in beagles. Masui 46(3): 351–357, 1997.
19. Merin R.G., Bernard J.M., Doursout M.F., et al. Comparison of the effects of isoflurane and desflurane on cardiovascular dynamics and regional blood flow in the chronically instrumented dog. Anesthesiology 74(3): 568–574, 1991.
20. Hayes M.A., Goldenberg I.S. Renal effects of anesthesia and operation mediated by endocrines. Anesthesiology 24: 487–499, 1963.
21. Boscan P., Pypendop B.H., Siao D.T., et al. Fluid balance, glomerular filtration rate, and urine output in dogs anesthetized for an orthopedic surgical procedure. AJVR 71(5): 501–507, 2010.
22. Wagener G., Brentjens T.E. Anesthetic concerns in patients presenting with renal failure. Anesthesiol Clin 28: 39–54, 2010.
23. Lassnigg A., Donner E., Grubhofer G., et al. Lack of renoprotective effects of dopamine and furosemide during cardiac surgery. J Am Soc Nephrol 11: 97–104, 2000.
24. Wohl J.S., Schwartz D.D., Flournoy S. et al. Renal hemodynamic and diuretic effects of low-dosage dopamine in anesthetized cats. J Vet Emerg Crit Care 17(1): 45–52, 2007.
25. Cowgill L.D., Elliott D.A. Acute renal failure. In: Textbook of Veterinary Internal Medicine, 5th ed. S.J. Ettinger and E.C. Feldman, eds. Philadelphia, PA: WB Saunders Co., 2000, pp 1615–1633.
26. Ho K.M., Power B.M. Benefits and risks of furosemide in acute kidney injury. Anaesthesia 65: 283–293, 2010.
27. McClellan J.M., Goldstein R.E., Erb H.N., et al. Effects of administration of fluids and diuretics on glomerular filtration rate, renal blood flow, and urine output in healthy awake cats. AJVR 67(4): 715–722, 2006.
28. Brienza N., Malcangi V., Dalfino L.i et al. A comparison between fenoldopam and low-dose dopamine in early renal dysfunction of critically ill patients. Crit Care Med 34(3): 707–714, 2006.
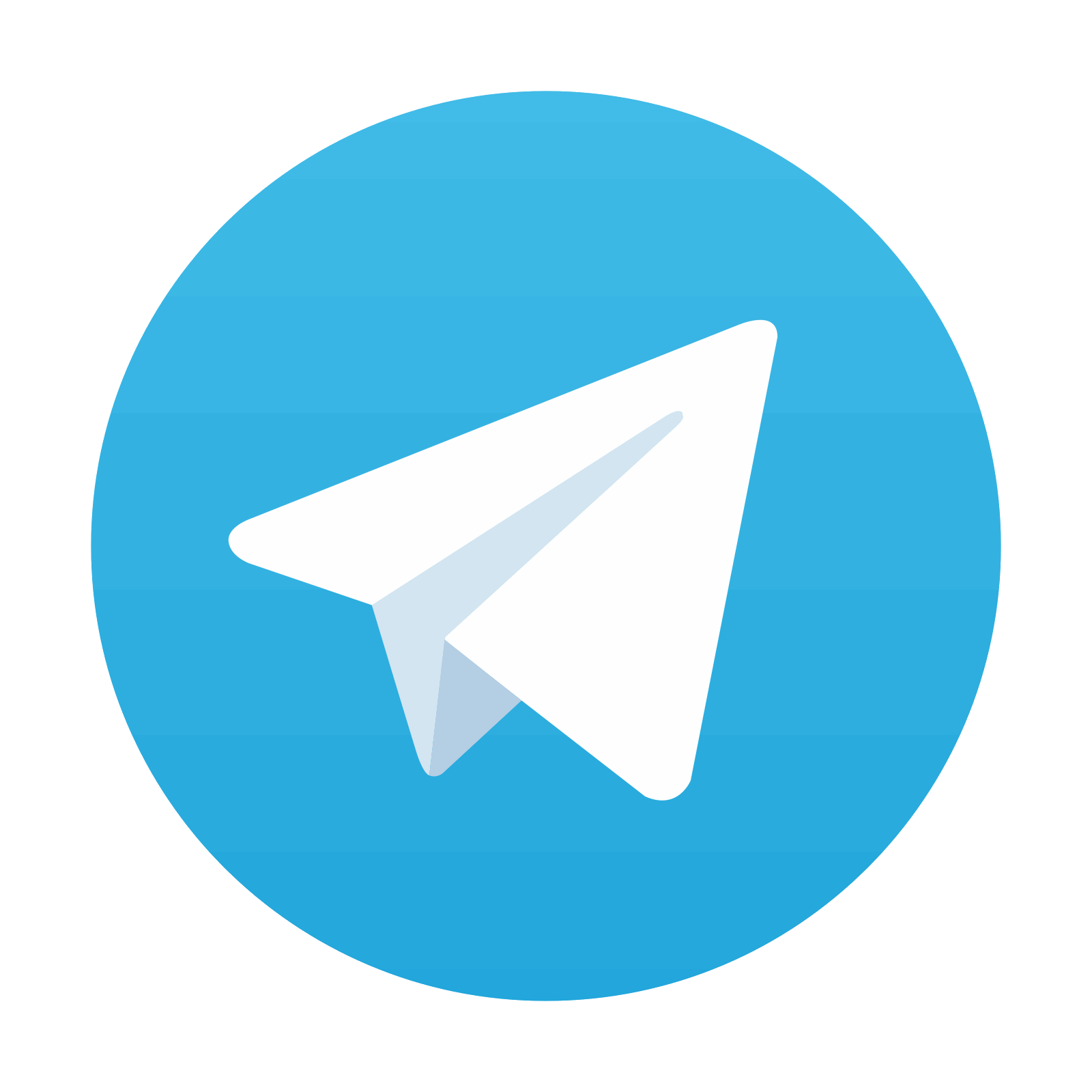
Stay updated, free articles. Join our Telegram channel

Full access? Get Clinical Tree
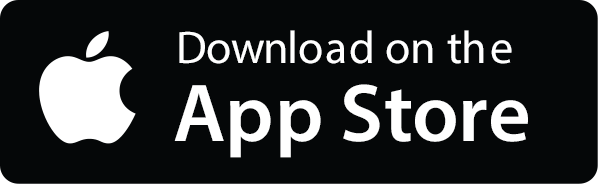
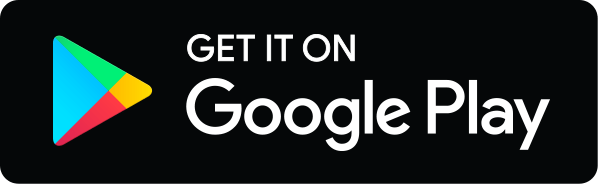