Introduction
Because the airway extends from the oral or nasal cavity to the alveoli, airway compromise or respiratory disease has numerous manifestations. Complications can be encountered in both the upper and lower airways and can encompass a vast range of problems, including laryngeal paralysis, collapsing trachea, pneumonia, pulmonary edema, pneumothorax, intrathoracic masses, and diaphragmatic hernias. Anesthesia can cause further complications since anesthetic drugs and equipment can exacerbate, or even cause, airway difficulties and respiratory compromise. When anesthetizing patients with respiratory disease or airway compromise the choice of anesthetic drugs is not necessarily dictated by the presence of the respiratory condition, but rather by the overall health of the patient and the risks to be managed. The choice of anesthetic technique (e.g., method of induction, method of intubation, use of positive pressure ventilation, etc.), on the other hand, is often the critical component of a successful outcome. Patient monitoring and support are critical and should be directed toward normalizing respiratory function and supporting the job of the respiratory system, which is to deliver oxygen (O2) to, and remove carbon dioxide (CO2) from, cells throughout the body.
Physiology of the respiratory system
Control of respiratory function includes an integrated series of complex feedback loops made up of sensors, controllers, and effectors. Dysfunction of any one of these components can lead to impaired respiratory system function.1 The end result of this concert of sensors, controllers, and effectors is regulation of alveolar ventilation (VA), which is the volume of inhaled gas that reaches the alveoli and participates in gas exchange. VA is often reported as minute ventilation (VE) which is the volume of inhaled gas participating in gas exchange multiplied by the respiratory rate. VA is determined by the ratio of the tidal volume (VT), or the amount of fresh gas inhaled and exhaled with each normal breath, to the dead space volume (VD), or the amount of inhaled fresh gas that does not participate in gas exchange. Physiological dead space (VD) is the volume of gas ventilating alveoli that are not perfused, (ventilation/perfusion [V/Q] mismatch; alveolar dead space), combined with the amount of gas ventilating the conducting airways (nasal passages, nasopharynx, larynx, trachea, and bronchi; anatomical dead space). Anesthetic drugs and equipment and the events of anesthesia can increase VD and decrease VT, thereby contributing to hypoventilation, which is defined simply as inadequate gas exchange or inadequate VA.
Hypoventilation results in hypercarbia (partial pressure of arterial CO2 [PaCO2] > 45–55 mm Hg), and when severe, hypoxemia (partial pressure of arterial O2 [PaO2] <60 mm Hg; partial pressure of arterial oxygen–hemoglobin saturation [SaO2] <90%). Because CO2 is extremely soluble and crosses the alveolar–arterial interface easily, CO2 that arrives at ventilated alveoli is readily delivered to the airway and exhaled. In fact, PaCO2, and thus, PACO2 (the partial pressure of CO2 in the alveoli), is related (but inversely) to VA. Most anesthetic drugs blunt the ventilatory response initiated by rising CO2 concentrations.
Hypoxemia, on the other hand, is not always caused by hypoventilation. Hypoxemia can also be caused by ventilation/perfusion (V/Q) mismatch, inadequate fraction of inspired oxygen (FIO2), diffusion impairment, and true anatomic shunt (Table 12.1). Two calculations used to determine the severity of hypoxemia, the alveolar-arterial oxygen difference ([A − a]DO2) and the partial pressure of arterial oxygen to the percent fraction of inspired oxygen ratio (PaO2/FiO2) are presented in Table 12.2.
Pharmacology of anesthetic drugs
Most anesthetic drugs produce some degree of respiratory depression. The degree of dysfunction is generally dose dependent and normally manageable when dosed appropriately in healthy patients. However, the degree of dysfunction may be significant in patients with preexisting disease or pathology of the respiratory or nervous system and cautious dosing and careful monitoring of the patient are imperative.
Sedatives and tranquilizers
Sedatives and tranquilizers are routinely used in patients with airway dysfunction, particularly upper airway dysfunction, to manage respiratory distress that can occur with excitement, anxiety, or pain. Commonly used sedatives include the phenothiazine derivatives (acepromazine), the benzodiazepines (diazepam and midazolam), and the opioids (morphine, hydromorphone, oxymorphone, fentanyl, methadone, butorphanol, buprenorphine, nalbuphine, etc.). Occasionally, the alpha2 adrenergic agonists (xylazine, medetomidine, and dexmedetomidine) are appropriate for patients with airway disease. The phenothiazine derivatives cause minimal respiratory dysfunction when used alone but can exacerbate respiratory depressant effects caused by other drugs.2 Acepromazine at low dosages causes a decrease in respiratory rate with a compensatory increase in VT, resulting in no changes in PaCO2, pH, PaO2, or SpO2.3 Because acepromazine produces mild sedation and has a fairly long duration of action, it is often used to calm anxious patients and is often appropriate for patients with airway disease/dysfunction. However, acepromazine is a muscle relaxant and relaxation of the muscles in the pharyngeal region can lead to further airway dysfunction. Acepromazine is not reversible and does not provide analgesia.
Table 12.1. The five causes of hypoxemia, contributing factors, and response to supplemental oxygen therapy
Cause of hypoxemia | Contributing factor | Response to supplemental oxygen and further diagnostics |
Hypoventilation | Inadequate alveolar ventilation (VA). Diagnosed by concurrent hypercarbia. | PaO2 will increase. Greatest increase will occur with increased VA. (A − a)DO2 is normal. |
Ventilation/perfusion (V/Q) mismatch also called physiological shunt | Any cause of pulmonary atelectasis: dorsal recumbency, GI distension, and pregnancy all cause compression atelectasis; ventilation with 100% oxygen can cause absorption atelectasis; other causes include pneumothorax and external compression of the thorax. | PaO2 will increase by varying degrees, depending on the magnitude of the mismatch. (A − a)DO2 is high. PaCO2 is normal. |
Decreased FiO2 | Primarily occurs only with supplementation of gases other than oxygen (e.g., supplementation with nitrous oxide) or with interruption of oxygen supply when increased FiO2 is expected. PaCO2 may be low due to hypoxemic drive. | PaO2 will increase. Greatest increase will occur when “o ffending” gas is discontinued and the patient is allowed to breathe an increased FiO2. (A − a)DO2 is normal. |
Diffusion abnormalities | Rarely a cause of hypoxemia in our patients, since red blood cells (RBCs) spend a large portion of time at the alveolar–arterial interface. However, could occur with any disease of this interface, for example, pulmonary edema or fibrosis. | PaO2 will increase. (A − a)DO2 is normal or increased. PaCO2 is normal. |
True anatomical shunt | Any anomaly that causes blood to be shunted from the right side of the heart into the systemic circulation, bypassing the lungs. Patent ductus arteriosus (PDA), tetralogy of Fallot, intrapulmonary shunts. | No change in PaO2 because the blood does not flow through the pulmonary circulation and thus cannot participate in gas exchange. (A − a)DO2 is high. PaCO2 is normal. |
The benzodiazepines (midazolam and diazepam) cause minimal to no respiratory depression4 and are also appropriate for patients with airway disease/dysfunction. As with acepromazine, the benzodiazepines can cause relaxation of muscles of the upper airway, but this is generally minimal. The benzodiazepines used alone rarely provide adequate sedation in distressed patients; thus, benzodiazepines are often combined with a member of the opioid class. Flumazenil can be used to reverse the effects of the benzodiazepines.
Table 12.2. Calculations used to determine the severity of hypoxemia, the alveolar–arterial oxygen difference ([A − a]DO2) and the oxygen partial pressure/fraction of inspired oxygen ratio (PaO2/FiO2)
Formula | Alveolar–arterial oxygen difference, (A − a)DO2; also called Alveolar–arterial gradient; also described as P(A − a)O2 | Oxygen partial pressure/fraction inspired oxygen ratio, PaO2/FiO2 |
Components of the formula | Alveolar oxygen (PAO2) equation: PAO2 = ([atmospheric pressure − water vapor pressure] × fraction inspired oxygen) − A lveolarCO2a/0.8b PaO2 is measured using an arterial blood gas. | PaO2 is measured using an arterial blood gas. FiO2 is measured or assumed (e.g., assumed to be 0.21 if a patient is breathing room air at sea level). |
Values at sea level in patient with normal lung function and hemoglobin concentration breathing FiO2 of 21%. | PAO2 = ([760 mm Hg − 47 mm Hg] × 0.21) − 40/0.8 = 100 mm Hg PaO2 = 95 mm Hg PAO2 − PaO2 = 100 − 95 = 5 mm Hg Normal values = 0 – 10 mm Hg is considered normal; 10 – 20 is considered mild gas exchange impairment; 20 – 30 is moderate impairment; and > 30 is severe. | PaO2 = 95 mm Hg FiO2 = 0.21 PaO2/FiO2 = 452 Normal values = 400 – 500 (300 – 400 may indicate insignifi cant changes); < 250 = severe gas exchange impairment. |
Advantages | Includes values for CO2, which can contribute signifi cantly to alveolar oxygen concentrations (i.e., high PACO2 is likely to cause low PAO2). | Easy to calculate. Can be used to estimate dysfunction at any FiO2; thus, patients on supplemental oxygen (or under anesthesia) DO NOT need to be disconnected from oxygen to assess pulmonary function. |
Disadvantages | A bit cumbersome to calculate. Does not work for patients on high FiO2. | Not as accurate as (A − a)DO2 because the formula does not include values for CO2. |
a Because CO2 is highly diffusible, PaCO2 is used to estimate PACO2.
b 0.8 is the respiratory quotient, which is the ratio of carbon dioxide production (VCO2) to oxygen consumption (VO2).
If the patient is extremely fractious, a low dose of an alpha2 adrenergic agonist, like medetomidine or dexmedetomidine, can be used. However, because of the potential for profound sedation, vomiting, relaxation of the musculature of the upper airway, and decreased respiratory rate and central respiratory drive,5 the patient should not be left unobserved following the administration of an alpha2 adrenergic agonist and the anesthetist should be prepared for immediate intubation or reversal of the effects of drug (e.g., atipamezole) in the event of profound cardiovascular or respiratory decompensation. Alpha2 adrenergic agonists provide analgesia as well as sedation.
Opioid-mediated respiratory depression is a major concern in human patients but only a minor concern in most veterinary patients since dogs and cats do not seem to be as sensitive to the respiratory depressant effects of the opioids. However, opioids do cause some dose-dependent, centrally mediated respiratory depression, and this is exacerbated by the concurrent use of other drugs that depress ventilatory function, like the inhalant anesthetic drugs. Because opioids provide sedation and analgesia, they are often the best choice for patients that need both calming and pain relief. Also, the opioids are reversible (e.g., with naloxone) and their effects can be eliminated if the patient has an adverse, or excessively profound or prolonged, reaction. Reversal of side effects associated with opioid analgesics with naloxone will also antagonize analgesia.6 Rapid intravenous (IV) administration of naloxone has been associated with the development of cardiac dysrhythmias and even sudden death.7,8 Butorphanol is an agonistantagonist and can be used to antagonize respiratory depression mediated at the mu receptors while maintaining some analgesic action at the kappa receptors.9 Nalbuphine10 and buprenorphine can also be used to reduce, but not eliminate, the effects of mu opioid agonists.
Induction drugs
Propofol is a rapidly acting injectable anesthetic drug that causes respiratory depression through suppression of the central respiratory neuronal network that is marked by a decrease in both VT and respiratory rate.11 Propofol can also cause suppression of the ventilatory response to carbon dioxide and hypoxemia. 11 Propofol can also produce apnea after bolus administration and this effect is related to both dose and the speed of injection of the drug, with faster injection rates associated with a greater incidence of apnea.12
The dissociative anesthetics (ketamine and tiletamine) do not alter the response to CO2 and may actually cause an increase in respiratory rate for 2–3 minutes after IV injection. However, they can exacerbate respiratory dysfunction caused by other drugs.11 Rapid injection or excessive dosing of ketamine or tiletamine can cause apnea, but more commonly an apneustic breathing pattern (pause at the end of inspiration instead of at the end of expiration) is observed.
Etomidate can produce mild dose-dependent respiratory depression marked by decreased VT that is generally offset by increased respiratory rate.11 Although apnea following administration of etomidate can occur, the effects are short lived (3–5 minutes) and alleviated by limiting the rate of administration.
Maintenance drugs
The inhalant anesthetic gases isoflurane, sevoflurane, and desflurane are respiratory depressants that cause dose-dependent alteration of the (1) pattern of breathing, (2) depth of ventilation (VT), (3) ventilatory response to arterial hypoxemia, and (4) airway resistance.13 During inhalant anesthesia an increase in respiratory rate may occur but not to the degree adequate to compensate for the decrease in VT. Inhalant anesthetic drugs cause a dose-dependent increase in the set point at which arterial carbon dioxide drives spontaneous ventilation, resulting in a delayed response (altered apneic threshold) and a decreased response (altered carbon dioxide responsiveness) to increases in arterial carbon dioxide.13 Inhaled anesthetics also depress the ventilatory response to hypoxemia.
Analgesic drugs
Adequate pain management in patients with respiratory disease or airway compromise is essential in the prevention of further respiratory dysfunction. Intermittent boluses of opioids (e.g., fentanyl, hydromorphone, or morphine) are appropriate, but a constant rate infusion (CRI) can provide a more consistent level of analgesia. Fentanyl, administered at either 5 or 10 mcg/kg/h, has been shown to not significantly change the respiration rate or have a clinically relevant effect on SpO2 in dogs.14 However, careful monitoring of ventilation and availability of respiratory supportive measures (e.g., oxygen, endotracheal [ET] tube, etc.) should be considered. Morphine or hydromorphone would also be appropriate for an infusion, and combinations of opioids with drugs like lidocaine and/or ketamine provide multimodal analgesia and may be more effective than single-drug administration in many patients.
Local anesthetic drugs are an excellent addition to any analgesic protocol since they not only provide immediate pain relief but also may decrease the incidence of central sensitization (i.e., windup). Nonsteroidal anti-inflammatory drugs may be utilized when appropriate since the pain from surgery is primarily from inflammation.
Anesthesia for patients with specific respiratory disease or airway compromise
Patients with compromised airways may present for surgery of the respiratory system (e.g., arytenoid cartilage lateralization or removal of a consolidated lung lobe) or may present for surgery of other organ systems with airway compromise as a complication (e.g., dental prophylaxis in a patient with collapsing trachea or removal of an intestinal foreign body in a patient with aspiration pneumonia). Regardless of the reason for presentation, the patient should be carefully evaluated before induction of anesthesia. The location, extent, and severity of the problem should be assessed and the degree of respiratory dysfunction should be determined. Along with a routine physical exam, the evaluation should include any specific tests (e.g., thoracic radiographs, bronchoscopy, arterial blood gases, etc.) necessary to fully define the extent of the pathology.
During evaluation the patient should be handled quietly and carefully to avoid stressinduced tachypnea with subsequent increased work of breathing and possible further respiratory dysfunction. Many patients that breathe with difficulty can have elevated body temperatures that may result in further increases in respiratory effort. Tranquilization is often necessary to keep the patient calm and analgesia is required for any painful patients.
Oxygen should be administered to all patients with respiratory disease during handling and during preparation for anesthesia. Hypoxemia can occur rapidly regardless of the location of the airway dysfunction. However, preoxygenation may delay desaturation.15
Anesthesia for patients with upper airway dysfunction
Patients in this category include those with laryngeal paralysis, laryngeal/pharyngeal tumors, airway trauma, cervical tracheal collapse, and upper airway foreign bodies. Other potential complications include diseases that cause external compression of the airway like enlarged pharyngeal lymph nodes or grossly enlarged thyroid glands. Brachycephalic breeds, like bulldogs and pugs, often have a plethora of abnormalities that can lead to upper airway obstruction. Brachycephalic syndrome includes elongated soft palate, everted laryngeal saccules, hypoplastic trachea, and stenotic nares.
The induction and recovery phases of anesthesia can be associated with significant risk in patients with upper airway dysfunction but the maintenance phase is straightforward since the airway is controlled during maintenance. However, upper airway dysfunction can develop in the maintenance period if the ET tube becomes dislodged (e.g., during patient repositioning for surgery, radiology, or dentistry), plugged (i.e., by blood or mucous) or kinked, or if the ET tube cuff is grossly overinflated, leading to collapse of the lumen of the tube.16
Preanesthetic preparation
Patients presenting for surgery of the upper airway generally present with airway narrowing and/or airway dysfunction and are often at risk for total airway obstruction. In addition to the anatomic narrowing of the airway, a variety of factors can cause a physiological narrowing of the airway. Increased respiratory drive, which can be caused by a multitude of factors including pain, excitement, hyperthermia, exercise, hypoxia, and hypercarbia, causes an increase in respiratory effort, which, in turn, causes greater negative pressure in upper airway. This negative pressure pulls the structures of the pharynx into the airway and causes an upper airway collapse. Judicious dosages of sedatives and analgesic drugs may allow the patient to breathe slower and deeper, which alleviates or even eliminates excitement or anxiety-induced increased work of breathing and the resultant exacerbation of negative pressures. Opioids alone are often adequate for sedation in dogs, but panting may occur following some opioids (e.g., hydromorphone). Also in dogs, a combination of acepromazine and buprenorphine has been shown to cause minimal respiratory effects and better sedation than acepromazine used alone.17 Midazolam or diazepam can also be combined with buprenorphine or butorphanol for mild to moderate sedation that is reversible. Mu agonist opioids (e.g., morphine or hydromorphone) can be used in cases that are in moderate to severe pain and where vomiting is not problematic. Protocols for cats are similar to those used in dogs but opioids may cause excitement and are almost always used in conjunction with a sedative.
In some instances, laryngeal function needs to be evaluated prior to intubation. Small boluses of propofol (2–4 mg/kg) are generally used. The use of 0.2 mg/kg IV diazepam or midazolam just before the propofol boluses will decrease the amount of propofol needed to complete the exam but may interfere with laryngeal function. Thiopental is also useful and both propofol and thiopental are more effective than ketamine/diazepam for upper airway exams.18 The patient should be intubated immediately after the examination has concluded.
The use of anticholinergics in the preoperative period is controversial. Laryngeal manipulation can cause vagally mediated bradycardia and brachycephalic breeds tend to have high resting vagal tone. Thus, anticholinergics are often used in brachycephalic breeds and other patients with upper airway disease. The use of anticholinergics as antisialagogues and to decrease respiratory tract secretions is generally not recommended, although excessive secretion production may create an exception to this rule.
Induction
If tolerated, the patient should be preoxygenated while the sedatives are taking effect. During this time, an IV catheter should be placed. Following adequate sedation, the patient should be rapidly induced to anesthesia and immediately intubated. Any of the fast-acting injectable induction drugs are appropriate (e.g., propofol, thiopental, or ketamine/diazepam). Induction with inhalant anesthetic gases is too slow to ensure expedient control of the airway without undue stress and is not generally acceptable in patients with airway compromise.
Intubation
The rapid establishment of an airway in all patients, and especially in patients with upper airway disease, is critical. Because intubation needs to occur as rapidly and as smoothly as possible, preparation for intubation is imperative. ET tubes of several different sizes, a laryngoscope, stylet (especially when intubating cats), and tracheostomy kit should all be readily available. The ET tubes should be measured to ensure that they are long enough to bypass any upper airway obstruction but not so long that the tip of the tube could end up in a bronchus.
In all patients, the larynx should be visualized at intubation and the ET tube should be observed passing between the arytenoids. Laryngospasm, which will impede successful intubation, occurs most frequently in cats, swine, rabbits, and primates, but has also been observed in dogs and horses.1 Laryngospasm may be triggered by touching the larynx during a light plane of anesthesia. Topical application of a local anesthetic such as lidocaine is recommended prior to tracheal intubation in species prone to laryngospasm (e.g., cats) to minimize tactile stimulation-induced laryngospasm. Small boluses of additional propofol (1–2 mg/kg) may also be used to improve laryngeal relaxation and facilitate intubation. However, propofol is a potent respiratory depressant and this technique should be quickly followed by proper placement of the ET tube.
Once intubated, the ET tube cuff should be carefully inflated. To prevent damage to the trachea, patients should be disconnected from the breathing tubes if they are moved or repositioned. Damage from an overinflated cuff or from cuff rotation can cause postoperative tracheal constriction or laceration. This is one of the most common causes of iatrogenic postanesthetic upper airway dysfunction in cats.19
Maintenance
Once the patient with upper airway dysfunction is intubated, the patient can be allowed to breathe spontaneously as long as adequate gas exchange occurs. If the ET tube does not extend past the obstruction (e.g., intrathoracic tracheal collapse), ventilatory support will be necessary, and this is discussed in the next section. Appropriate monitoring and support of the patient are critical. If the patient must be extubated during the operative procedure (e.g., for evaluation of airway diameter following an arytenoid cartilage lateralization), dosages of injectable induction drugs, a laryngoscope, a variety of ET tubes (in case the first one can’t be reinserted), and a stylet must be readily available. If the patient cannot be intubated because of the location of the surgical repair, anesthesia can be maintained with injectable anesthesia. A CRI of propofol (0.1–0.4 mg/kg/min) with intermittent boluses (1–2 mcg/kg) of fentanyl or a concurrent infusion of fentanyl (0.02–0.1 mcg/kg/min) and supplemental oxygen is an effective means of providing total IV anesthesia.
Recovery
A low-stress recovery is essential in patients with upper airway dysfunction. Excitement and pain, with subsequent increased ventilation and increased peak inspiratory pressure, can cause airway edema and obstruction. Thus, patients should be adequately sedated (e.g., acepromazine) and should be administered analgesics (e.g., opioids) as needed. The patient should remain intubated for as long as it will tolerate the ET tube (if orotracheally intubated) or as long as the upper airway might reobstruct (if intubated through a tracheostomy site). The administration of a single dose of short-acting corticosteroids is often recommended to alleviate or attenuate obstruction of the airway from postoperative swelling. If upper airway edema is present, a diuretic (e.g., 0.5–1.0 mg/kg IV furosemide) can be administered in addition to the corticosteroids. Administration of phenylephrine nasal drops will counteract nasal passage hyperemia or edema. Because of the presence of redundant tissue in the pharynx, most brachycephalic breeds (e.g., bulldog, pug, and Shar Pei) benefit from having the anesthetist hold the dog’s tongue and/or extend its neck immediately after extubation of the trachea.16 In all instances, reobstruction should be anticipated and the anesthesiologist should be prepared to reanesthetize and reintubate the patient or perform a tracheostomy. Thus, injectable anesthetic drugs, a laryngoscope, a variety of ET tubes, and a tracheostomy kit should be readily available.
Dogs with upper airway obstruction can develop life-threatening pulmonary edema.20 Pulmonary edema formation after airway obstruction is probably multifactorial, so treatment is symptomatic. Oxygen supplementation to maintain SpO2 greater than 90%, diuretics, and corticosteroids can be used to treat pulmonary edema.
The effects of opioids can be reversed if the patient is having an exaggerated response to these drugs in recovery. However, painful or distressed animals may experience more respiratory difficulty following extubation, so the benefits of opioid reversal should be carefully weighed against the potential complications of the increased pain and stress that may accompany reversal (i.e., increased upper airway pressure secondary to paininduced increased respiratory drive). Monitoring and oxygen supplementation should continue until the patient is ventilating normally.
Anesthesia for patients with lower airway dysfunction and/or intrathoracic surgery
Patients with pulmonary dysfunction may lack the ability to expand their lungs properly (extrapulmonary disease) and/or may have impairment of oxygen and carbon dioxide transfer across the alveolar membranes (intrapulmonary disease). Examples of extrapulmonary disease include diaphragmatic hernia, pneumothorax, chylothorax, hemothorax, pyothorax, intrathoracic masses, flail chest, and any condition that restricts chest wall expansion (e.g., fractured ribs) or impairs diaphragmatic movement (e.g., obesity, pregnancy or gastrointestinal [GI] distention). Examples of intrapulmonary disease include asthma, chronic obstructive pulmonary disease (COPD), pneumonia, pulmonary edema, intrapulmonary hemorrhage (contusions), atelectasis, or consolidated lung lobes. Patients that have normal lower airway function that must undergo thoracic surgery are also included in this category and include patients with intrathoracic collapsing trachea/bronchi or patients needing intrathoracic cardiovascular surgery.
Patients with lower airway dysfunction may present with, or develop, metabolic derangements including acid-base imbalance (due to hypercarbia) and hypoxemia. These conditions are likely to develop even in patients with normal lung function if the surgical procedure requires an open thorax. Patients may be an emergency if they present with, or develop, a tension pneumothorax, which requires immediate thoracocentesis.
Premedicants
In all patients, fear, pain, and excitement can cause an increased oxygen demand that will result in an increased respiratory drive. If oxygen demand is not met by oxygen delivery, oxygen debt, or hypoxemia with resultant tissue hypoxia, occurs. Pulmonary disease may limit the patient’s ability to oxygenate so oxygen debt is likely if patients with lower airway disease experience fear, pain, or excitement. Sedation and analgesia allow slower, deeper breathing with no increase in oxygen demand. Acepromazine, the benzodiazepines, and most members of the opioid class (at low dosages) are generally good choices for sedation. If the patient is extremely obtunded, sedation may be precluded but opioids should be part of the anesthetic protocol as a means to provide analgesia and to lower the dosages of induction and maintenance drugs.
The use of anticholinergics is, again, controversial, although both atropine and glycopyrrolate do have a bronchodilating effect. Bronchodilation occurs due to antagonism of acetylcholine effects on airway smooth muscle and the effects are most prominent in large and medium-sized airways. This effect leads to lowered airway resistance, but also to increased anatomical dead space.21 The possible beneficial effects of bronchodilation should be weighed against the detrimental effects of tachycardia and inspissation of secretions. Anticholinergics change secretory composition from a watery fluid to a thick mucous. This mucous may not be adequately cleared by the mucociliary system and may remain in the respiratory tree, where it can promote bacterial growth.
Induction
Any of the rapidly acting injectable anesthetic agents are acceptable choices for induction of anesthesia. Both ketamine and propofol have mild bronchodilating effects and may reduce bronchospasm in asthmatic patients.22 Propofol may be the best choice since it produces anesthesia very quickly and can easily be titrated to effect. Although propofol produces apnea similar to that produced by barbiturates, the effects of propofol are quickly dissipated, allowing ventilation to rapidly return to normal. Etomidate and tiletamine/zolazepam are also appropriate induction drugs for patients with lower airway disease.
Maintenance
Inhalant anesthesia with controlled ventilation is generally the safest method for anesthetic maintenance of patients with lower airway disease. Inhalant gases have a nonspecific bronchodilating effect. This is especially beneficial in patients who have reactive and/or constricted airways (e.g., cats with asthma) and in those who may undergo surgery that requires direct manipulation of the airways (e.g., lung lobectomy). Inhalant anesthetic gases are potent respiratory depressants and will cause a dose-dependent decrease in VA and impaired ventilatory response to hypercarbia and hypoxemia. Thus, excessive anesthetic depth must be avoided. Appropriate analgesia will allow a decrease in inhalant gas requirements.
Mechanical ventilation
Intermittent positive pressure ventilation (IPPV) is generally recommended in patients with lower airway disease in order to maximize alveolar function. IPPV is mandatory in patients with an open thorax. IPPV should be instituted at 10–15 breaths per minute, a VT of 10–15 mL/kg, and a peak inspiratory pressure of 10–20 cm H2O. Appropriate ventilator settings can be determined by monitoring end-tidal carbon dioxide (ETCO2) (the goal is to maintain ETCO2 between 35 and 55mmHg). Positive end-expiratory pressure (PEEP) is occasionally necessary to maintain adequate gas exchange, especially in patients with an open thorax. A PEEP of 5–10cmH2O is often effective in maintaining adequate PaO2. However, inappropriate use of IPPV and PEEP (i.e., excessive airway pressures) may cause decreased venous return and decreased cardiac output. This is more of a concern in hypovolemic patients, and appropriate fluid loading can attenuate the negative cardiovascular effects of mechanical ventilation. If a positive-pressure ventilator is not available, a staff member should be dedicated to maintaining ventilation in the patient by manual intermittent squeezing of the breathing circuit reservoir bag.
Recovery
Patients recovering from thoracic surgery are at high risk for development of postoperative pulmonary complications, including atelectasis and/or pneumonia. The duration of surgery should be kept as short as possible since duration of surgery is highly correlated with postoperative dysfunction with surgeries of 1, 2, 4, and greater than 4 hours, causing postoperative complications in 4%, 23%, 38%, and 73% (respectively) of patients.23
Provision of adequate postoperative analgesia is beneficial for return of normal ventilatory function. Pain associated with breathing can cause splinting or tensing of the abdomen, active exhalation, and failure to cough. These actions intensify respiratory dysfunction by promoting retention of secretions, airway closure, and atelectasis. Following thoracotomy, intraoperative blockade of the intercostal muscles24 with local anesthetic drugs may provide analgesia for 60–360 (depending on the local anesthetic drug used) minutes postoperatively. Infusion of local anesthetics directly into a chest will also provide some degree of analgesia but fluid and blood in the chest will dilute the agents and decrease their efficacy; the patient may need to lie with the surgery site dependent (this may be uncomfortable for the patient) so that the local anesthetic comes in contact with the incision and large volumes of anesthetic agent may be necessary in order to cover adequate surface area. Constant IV infusions of opioids, ketamine, and/or lidocaine are easy to use and will also provide effective analgesia. The administration of opioids through epidural catheters that are advanced into the thoracic region is a pain relief technique commonly used in human beings but not commonly used in veterinary patients. Morphine epidurals are effective for the relief of thoracic pain in dogs and should be considered as an analgesic option.25
Monitoring and support of both respiratory and cardiovascular function should continue well into the recovery period. Support should include administration of oxygen, maintenance of circulating fluid volume through the administration of IV fluids, and normalization of electrolyte and acid–base status. Following thoracotomy, a chest drain should be maintained until negative pressure can be consistently achieved with minimal to no aspiration of air, blood, or fluid.
Revised from “Pulmonary Disease” by Robert R. Paddleford and Stephen A. Greene in Lumb and Jones’ Veterinary Anesthesia and Analgesia, Fourth Edition.
1. Paddleford R.R., Greene S.A. Pulmonary disease. In: Lumb & Jones Veterinary Anesthesia, 4th ed. W.J. Tranquilli, J.C. Thurmon, and K.A. Grimm, eds. Ames, IA: Wiley-Blackwell, 2007, pp 899–903.
2. Baldessarini R.J., Tarazi F.I. Drugs and the treatment of psychiatric disorders. In: Goodman & Gilman’s the Pharmacological Basis of Therapeutics, 10th ed. J.G. Hardman and L.E. Limbird, eds. New York: McGraw-Hill, 2001, pp 485–520.
3. Popovic N.A., Mullane J.F., Yhap E.O. Effects of acetylpromazine maleate on certain cardiorespiratory responses in dogs. Am J Vet Res 33: 1819–1824, 1972.
4. Haskins S.C., Farver T.B., Patz J.D. Cardiovascular changes in dogs given diazepam and diazepa-ketamine. Am J Vet Res 17: 795–798, 1986.
5. Lerche P., Muir W.W. Effect of medetomidine on breathing and inspiratory neuromuscular drive in conscious dogs. Am J Vet Res 65(6): 720–724, 2004.
6. Copland V.S., Haskins S.C., Patz J. Naloxone reversal of oxymorphone effects in dogs. Am J Vet Res 50: 1854–1858, 1989.
7. Michealis L.L., Hickey P.R., Clark T.A., et al. Ventricular irritability associated with the use of naloxone hydrochloride. Ann Thorac Surg 18: 608–614, 1974.
8. Andree R.A. Sudden death following naloxone administration. Anesth Analg 59: 782–784, 1980.
9. McCrackin M.A., Harvey R.C., Sackman J.E., et al. Butorphanol tartrate for partial reversal of oxymorphone-induced postoperative respiratory depression in the dog. Vet Surg 23: 67–74, 1994.
10. Jacobson J.D., McGrath C.J., Smith E.P. Cardiorespiratory effects of four opioid-tranquilizer combinations in dogs. Vet Surg 3(4): 299–306, 1994.
11. Stoelting R.K. Nonbarbiturate induction drugs. In: Pharmacology and Physiology in Anesthetic Practice, 3rd ed. R.K. Stoelting, ed. Philadelphia, PA: Lippincott, Williams & Wilkins, 1999, pp 140–157.
12. Musk G.C., Pang D.S., Beths T., et al. Target-controlled infusion of propofol in dogs-evaluation of four targets for induction of anaesthesia. Vet Rec 157(24): 766–770, 2005.
13. Stoelting R.K. Inhaled anesthetics. In: Pharmacology and Physiology in Anesthetic Practice, 3rd ed. R.K. Stoelting, ed. Philadelphia, PA: Lippincott, Williams & Wilkins, 1999, pp 36–76.
14. Lemmens S., Stienen P.J., Jaramillo L.G., et al. The cardiorespiratory effects of a fentanyl infusion following acepromazine and glycopyrrolate in dogs. Tijdschr Diergeneeskd 133(21): 888–895, 2008.
15. McNally E.M., Robertson S.A., Pablo L.S. Comparison of time to desaturation between preoxygenated and nonpreoxygenated dogs following sedation with acepromazine maleate and morphine and induction of anesthesia with propofol. Am J Vet Res 70(11): 1333–1338, 2009.
16. Greene S.A., Harvey R.C. Airway Disease. In: Lumb & Jones Veterinary Anesthesia, 4th ed. W.J. Tranquilli, J.C. Thurmon, and K.A. Grimm, eds. Ames, IA: Wiley-Blackwell, 2007, pp 937–943.
17. Stepien R.L., Bonagura J.D., Bednarski R.M., et al. Cardiorespiratory effects of acepromazine maleate and buprenorphine hydrochloride in clinically normal dogs. Am J Vet Res 56(1): 78–84, 1995.
18. Gross M.E., Dodam J.R., Pope E.R., et al.Acomparison of thiopental, propofol, and diazepamketamine anesthesia for evaluation of laryngeal function in dogs premedicated with butorphanol-glycopyrrolate. J Am Anim Hosp 38(6): 503–506, 2002.
19. Mitchell S.L., McCarthy R., Rudloff E., et al. Tracheal rupture associated with intubation in cats: 20 cases (1996–1998). J Am Vet Med Assoc 216(10): 1592–1595, 2000.
20. Kerr L.Y. Pulmonary edema secondary to upper airway obstruction in the dog: a review of nine cases. J Am Anim Hosp Assoc 25: 207–212, 1989.
21. Gotta A.W., Ray C., Sullivan C.A., et al. Anatomical dead space and airway resistance after glycopyrrolate or atropine premedication. Can J Anesth 28(1): 51–54, 1981.
22. Burburan S.M., Xisto D.G., Rocco P.R. Anaesthetic management in asthma. Minerva Anestesiol 73(6): 357–365, 2007.
23. Kroenke K., Lawrence V.A., Theroux J.F., et al. Operative risk in patients with severe obstructive disease. Arch Intern Med 152(5): 967–971, 1992.
24. Skarda R.T., Tranquilli W.J. Local and regional anesthetic and analgesic techniques: dogs. In: Lumb & Jones Veterinary Anesthesia, 4th ed. W.J. Tranquilli, J.C. Thurmon, and K.A. Grimm, eds. Ames, IA: Wiley-Blackwell, 2007, pp 561–595.
25. Pascoe P.J., Dyson D.H. Analgesia after lateral thoracotomy in dogs. Epidural morphine vs intercostal bupivacaine. Vet Surg 22(2): 141–147, 1993.
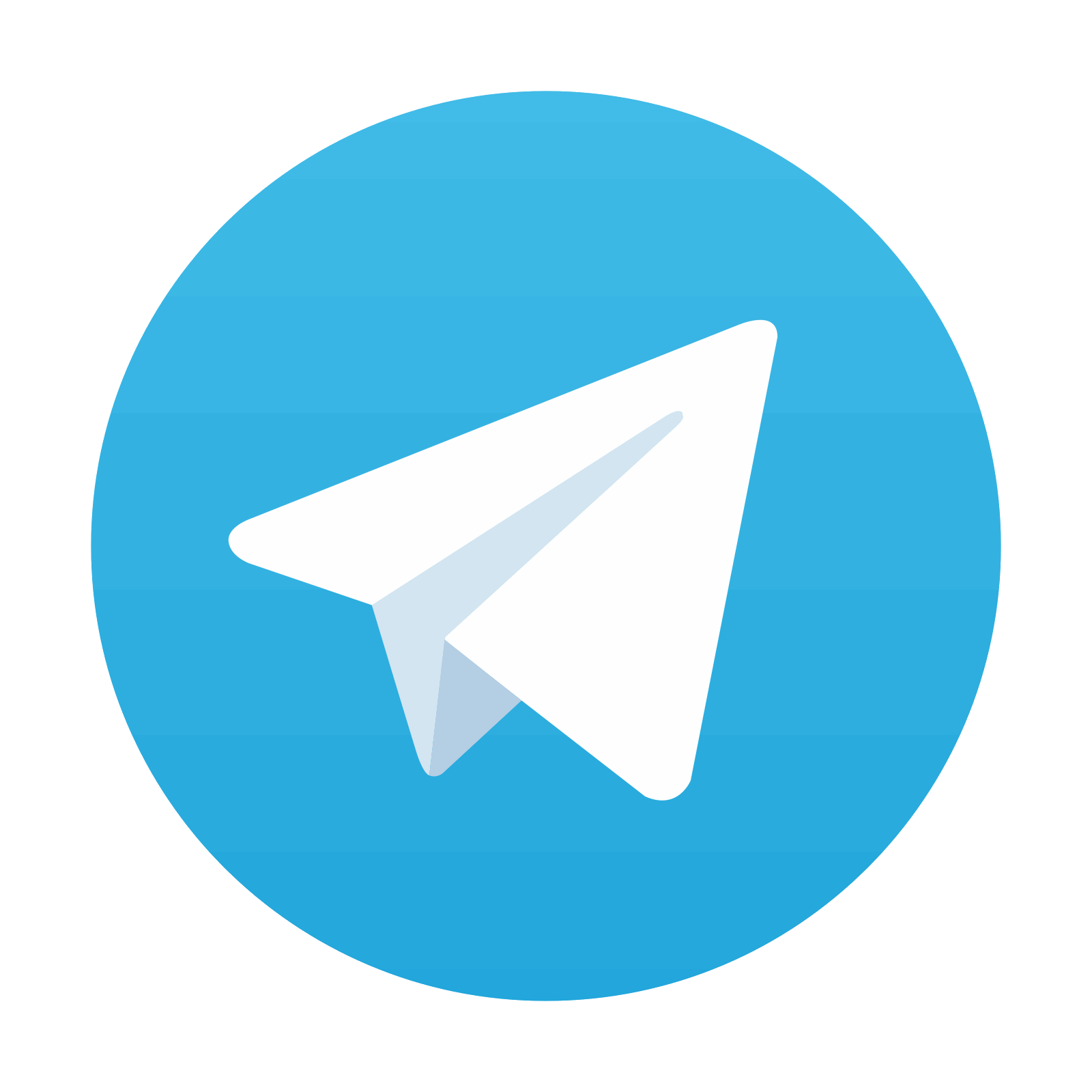
Stay updated, free articles. Join our Telegram channel

Full access? Get Clinical Tree
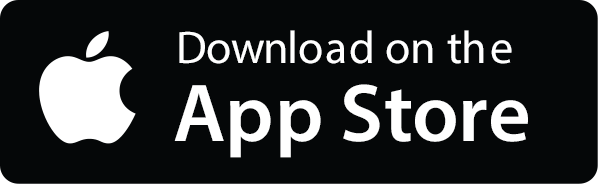
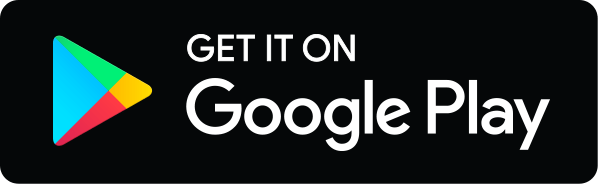