Anatomy and physiology
The liver has four primary functions: (1) synthesis of proteins, (2) detoxification of waste products, (3) biotransformation of drugs, and (4) storage of glycogen. The liver has a dual blood supply with approximately 70% derived from the hepatic portal vein and the remaining 30% coming from the hepatic artery. It is important to realize that only the hepatic arterial blood is well oxygenated and it is responsible for about 50% of the oxygen delivered to the liver.
Any factor that decreases systemic arterial blood pressure (ABP) and/or cardiac output will result in decreased portal vein flow. In normal, conscious patients this is partially compensated for by increased hepatic arterial flow. In anesthetized animals or animals with liver disease, though, this autoregulatory mechanism is lost and the risk of ischemia is increased.
Total hepatic blood flow is determined by hepatic perfusion pressure (mean arterial or portal venous pressure minus hepatic venous pressure) and splanchnic vascular resistance. Decreases in the former or increases in the latter will reduce hepatic blood flow. Positive pressure ventilation of the lungs, congestive heart failure, and fluid overload can all decrease hepatic perfusion pressure by increasing central venous and hepatic venous pressures. Activation of the sympathetic nervous system due to pain, hypoxemia, or surgical stress will increase splanchnic vascular resistance.
The anesthetist must also be aware that some hepatobiliary diseases have breed predispositions. Portosystemic vascular abnormalities, for example, are common in certain small purebreds, especially Maltese terriers, miniature Schnauzers, and Yorkshire terriers.
Animals with hepatic insufficiency may present with a variety of clinical signs including depression, anorexia, weight loss, icterus, ascites, hepatic encephalopathy (HE), and seizures. However, the liver has a great reserve capacity and these signs may be absent even in the face of significant hepatic disease. Only with exhaustion of hepatic functional reserve do clinical signs become evident. Consequently, a lack of clinical signs cannot be interpreted as absence of hepatic disease.
Serum hepatocellular enzyme concentrations, such as alanine aminotransferase (ALT), aspartate aminotransferase (AST), alkaline phosphatase (ALP), and γ-glutamyl transpeptidase (GGT) are commonly elevated with hepatobiliary disease. Elevation of these enzymes, although highly sensitive to hepatic damage, is not specific to liver insufficiency or even primary liver disease. Also, in chronic liver disease where hepatic tissue can be largely replaced by fibrosis, liver enzymes might be normal or only slightly elevated. Substrate metabolism tests such as pre- and postprandial bile acids or the elimination of indocyanine green are better indicators of hepatic function. Low serum values of albumin, glucose, and urea nitrogen are usually present in liver dysfunction but are not pathognomonic for liver disease.
A large array of coagulation proteins are produced by the liver and can also be diminished. A coagulation profile should be performed to aid in prediction of possible surgical complications in all patients that have suspected coagulopathy or hepatopathy. Blood typing and plasma and blood crossmatching are recommended before anesthesia if the coagulation profile is abnormal or if liver surgery is planned. Prolongation of prothrombin time (PT) and activated partial thromboplastin time (aPTT) do not necessarily give a diagnosis of active hemorrhage unless clinical signs such as anemia, petechiation, and prolonged bleeding from venous puncture are also present. Fresh frozen plasma can be used to supplement coagulation factors in these patients. Whole blood can be used; however, it should be reserved for anemic patients.
The liver synthesizes several plasma proteins and detoxifies ammonia, the major byproduct of protein metabolism. Albumin is exclusively produced in the liver. Because the liver normally produces albumin at only a third of its capacity, hypoalbuminemia is seen only in severe cases of hepatic disease and portosystemic shunting. Glomerular disease, protein-losing enteropathies, and hemorrhage are other nonhepatic causes of hypoalbuminemia to consider. Albumin is of great importance in the equilibrium of Starling forces due to exertion of colloidal osmotic pressure in the vascular bed. Also, albumin is a common binding site for anesthetic drugs, and therefore alterations in its plasma concentration can, in theory, affect the volume of distribution and half-life elimination of these drugs.
The liver plays an important role in glucose homeostasis. Patients with portosystemic shunts or acute hepatic failure are often hypoglycemic. Mechanisms of hypoglycemia in hepatic dysfunction include one or a combination of the following: decreased gluconeogenesis, decreased glycogen stores, and diminished response to glucagon. Monitoring and maintenance of normoglycemia should be a priority during anesthesia. Dextrose solutions (1–5%) can be given in combination with other isotonic crystalloids during surgery as needed to maintain normoglycemia. During anesthesia, fluid therapy is indicated to counteract relative hypovolemia secondary to anesthetic-induced vasodilation and to replenish fluid losses associated with respiration and surgical exposure of tissues. The use of hypotonic fluids (such as 5% dextrose) alone is not appropriate for this purpose, as they will precipitate a shift in free water from the plasma to the interstitium after rapid breakdown of the dextrose molecule. Consequently, an isotonic crystalloid with or without a colloid is recommended for intraoperative fluid therapy.
Sedatives
Animals with primary liver disease that require anesthesia are often obtunded to some degree. Therefore, most patients require minimal sedation depending on the procedure to be performed. Frequently, the administration of an opioid is enough to provide adequate sedation. Hydromorphone, oxymorphone, and methadone are full agonist opioids that provide good sedation and analgesia for diagnostic or surgical treatments in patients with hepatic disease. Compared to morphine, they present some potential advantages as they are not associated with elevations in plasma histamine concentrations and decreased blood pressure after intravenous administration.1–3 A decrease in systemic vascular resistance could possibly translate into a decrease in hepatic blood flow and subsequently decreased drug elimination capacity.4 Opioids with short elimination half-lives such as fentanyl and remifentanil can also be used as constant rate infusions in these patients. Care should be taken, however, because the context-sensitive half-life of most opioids used in constant rate infusions will be longer compared to normal patients.5 Remifentanil is unique in that it is metabolized by plasma esterases and shows no accumulation after constant rate infusion in humans.6
Most of the drugs used for their sedative properties in veterinary medicine can be categorized into one of three groups: phenothiazines, benzodiazepines, and alpha2 adrenergic agonists. Acepromazine is the most commonly used phenothiazine and has sedative and anxiolytic effects in dogs and cats. Acepromazine is not always contraindicated in patients with liver disease but judicious assessment of the patient is necessary. Acepromazine causes vasodilation due to its antagonistic effect at alpha adrenergic receptors.7,8 Although acepromazine vasodilation may be beneficial to increase renal blood flow in anesthetized dogs, data are not available regarding its effects on hepatic blood flow.9 When a patient is hypoalbuminemic, intravascular colloidal osmotic pressure is diminished and hypovolemia and hypotension can be present even before sedation. In these cases, a combination of colloid (e.g., Hetastarch) and crystalloid fluid therapy may be necessary if acepromazine is to be used. Also, acepromazine in combination with atropine is known to affect platelet aggregation in dogs for a couple of hours after administration and should be avoided if coagulation abnormalities are present.10
Advanced liver disease may result in HE due to accumulation of ammonium, mercaptans, and endogenous benzodiazepine compounds.11,12 In humans, the benzodiazepine antagonist flumazenil is often used to minimize clinical signs associated with HE.13 The use of benzodiazepines in veterinary patients presenting with HE is controversial, as these patients may exhibit increased sensitivity to these drugs, resulting in aggravation of HE.14 However, in the absence of HE, benzodiazepines can be used safely in patients with liver disease. Sedation is generally obtained with a combination of diazepam or midazolam and an opioid. Unwanted effects can be reversed by flumazenil. In human patients, increased sensitivity to benzodiazepines and prolongation of effect is possible,15,16 so conservative dosing may be prudent in dogs and cats with liver disease.
Dexmedetomidine provides excellent sedative and analgesic effects yet may be associated with significant cardiovascular depression characterized by decreases in cardiac output of up to 50%.17,18 Despite this, hepatic blood flow is unchanged in normal dogs after intravenous administration of up to 10 mcg/kg based on the radioactive microsphere method.19 While it appears that hepatic blood flow is reasonably well preserved during sedation with medetomidine or dexmedetomidine, these drugs have also been shown to decrease hepatic enzyme activity in vitro. In this regard, though, it is likely that their sparing effects on doses of other anesthetic agents may be more clinically relevant than any direct effects on liver metabolic capacity.20 In some cases, alpha2 agonist administration may cause excessive sedation and central nervous system depression in an animal that is already obtunded as a result of hepatic disease; however, reversal with specific antagonists could be a major advantage. Sedative selection should be based on anticipated sedation requirements.
Analgesics
Opioids are the mainstay of analgesia during anesthesia and most induce few adverse cardiovascular effects. However, both morphine and meperidine are associated with significant release of histamine when given rapidly intravenously.1–3 Histamine release may cause hypotension and therefore reduce hepatic blood flow. In humans, morphine also causes spasm of the common bile duct by constriction of the sphincter of Oddi, which increases the size and pressure in the gallbladder. This effect has not been demonstrated or observed in veterinary medicine.21
Most opioids are biotransformed in the liver by oxidation or glucuronidation, and some are converted into active metabolites. Since patients with liver dysfunction may take longer to metabolize these drugs, their effects could last significantly longer than in a healthy patient.22,23 Frequent assessments of analgesia are required to determine appropriate administration intervals. Sufentanil, alfentanil, fentanyl, and remifentanil are short-acting opioids with pharmacokinetics that are minimally affected by liver disease.22,24 Remifentanil, in particular, is rapidly hydrolyzed by plasma esterases, resulting in a high clearance and rapid elimination. The context-sensitive half-life seems to be independent of the dose or duration of infusions in human subjects with varying degrees of liver dysfunction.22,25,26
Nonsteroidal anti-inflammatory drugs (NSAIDs) are commonly used for acute and chronic pain management in dogs and cats. All NSAIDs have the potential to cause hepatic injury, either intrinsically (as is usually the case with aspirin and acetaminophen) or idiosyncratically (as tends to be the case with other NSAIDs, including the COX-2 selective agents). Idiosyncratic toxic reactions are rare, unpredictable, and not related to dose. Though carprofen was initially thought to be unique in its propensity to cause acute, idiosyncratic hepatotoxicosis, any NSAID has this potential. Clinical signs include inappetence, vomiting, and icterus associated with elevations in serum hepatic enzymes and bilirubin. Any unexplained increase in these biochemical parameters after initiation of NSAID therapy should be investigated. Since there have been no large-scale prospective studies to evaluate the effects of NSAIDs on hepatic structure and function in animals with preexisting liver disease, it is unknown whether or not this practice is safe.
In addition, NSAIDs may also impair coagulation by affecting platelet aggregation and clot formation. In dogs with osteoarthritis, platelet aggregation decreased after aspirin or carprofen administration while it did not change after meloxicam or deracoxib. Thromboelastography testing in these dogs showed decreased clot strength after carprofen but increased strength after deracoxib.27 Due to the potential for adverse effects, the administration of NSAIDs to patients with hepatic dysfunction, especially those with coagulopathies, should be avoided or very closely monitored.
Injectable anesthetics
While there are numerous studies evaluating the effects of inhalant anesthetics on hepatic function and blood flow, there are relatively few regarding the impact of injectable anesthetics. Studies comparing the effects of ketamine, thiopental, and etomidate on canine hepatic blood flow suggest ketamine causes a more modest decrease in comparison to the others.28,29 Propofol has been shown to maintain hepatic blood flow via arterial vasodilation in dogs and thiopental appears to have similar effects.30,31
As a general rule, most agents have minimal effects on hepatic blood flow if systemic ABP is maintained within normal limits. Propofol is a phenolic compound in an aqueous emulsion that provides rapid onset of action and short emergence times due to its rapid redistribution and elimination. Propofol clearance exceeds hepatic blood flow, suggesting an extrahepatic site of metabolism and making it a good choice for patients with liver disease.32 Caution is warranted, however, due to its cardiovascular depressant and systemic vasodilatory effects.33
Thiopental is an ultrashort-acting barbiturate with a very quick onset of effect. It is heavily metabolized by the liver, has several active metabolites, and its administration results in increased liver enzyme activity.34,35 Although termination of its anesthetic effects results from redistribution to nontarget tissues, suggesting it may be acceptable to use as a single bolus for induction, it is probably best avoided to minimize the metabolic load placed on the liver.
Etomidate has been shown to cause minimal cardiovascular effects when compared to propofol in canine anesthetic inductions.33 Etomidate is an imidazole compound in a propylene glycol-based vehicle. Propylene glycol is a hyperosmolar molecule and may cause erythrocyte rupture.36 Because some patients presenting with hepatobiliary disease may have bilirubinemia and icterus due to biliary obstruction or decreased hepatic conjugation, the hemolysis caused by etomidate could potentially further overload the liver. While hemolysis does not appear to be significant in normal dogs after a single bolus,37 the clinical consequences in animals with liver disease have not been studied. Overall, etomidate seems to be a good induction agent for the liver patient due to its minimal cardiovascular effects, which preserve systemic and hepatic blood flow.
Dissociative anesthetics such as ketamine and tiletamine (Telazol®, Pfizer Animal Health, New York) are reasonable choices for induction of anesthesia in patients with hepatic disease. However, the coadministration of a benzodiazepine may result in prolonged effects in animals with impaired hepatic function. In dogs and cats, dissociative anesthetics are largely metabolized by the liver but, if used for induction only, the termination of effect will be fairly quick due to redistribution of the drug to muscle and fat.38 Dissociative anesthetics decrease the seizure threshold in dogs and cats and may promote seizure activity. They should be avoided in animals with a recent history of seizures or HE.
Inhalants
Hepatic blood flow is variably affected by inhalant anesthetics. In general, inhalants impair autoregulatory mechanisms (i.e., increased hepatic arterial flow in response to decreased portal venous flow) that would otherwise maintain total hepatic blood flow. Studies have shown that isoflurane, sevoflurane, and desflurane better maintain total hepatic blood flow, oxygen supply, and delivery/consumption ratios compared to halothane or enflurane.39 While sevoflurane and desflurane appear to have the least effect on hepatic blood flow,40 it is important to note that, among the commonly used agents (isoflurane, sevoflurane, and desflurane), the effect is dose dependent. Consequently, efforts to reduce the dose administered will have a significantly greater impact than choice of anesthetic per se.
Neuromuscular blockers
The disposition of neuromuscular blocking drugs (NMBs) may be affected by liver disease. Vecuronium, rocuronium, and mivacurium all have increased volumes of distribution, prolonged elimination half-lives, and prolonged durations of effect.41–43 Atracurium and cisatracurium undergo liver-independent elimination (by nonspecific ester hydrolysis or Hofmann elimination) and have similar pharmacokinetics and pharmacodynamics in both healthy and liver-diseased patients.44–46
Anesthesia for specific procedures
Liver disease can have a significant impact on drug pharmacokinetics and pharmacodynamics depending on which hepatic functions are altered and the degree of insufficiency. Alterations in protein binding due to decreased production of binding proteins (e.g., albumin and alpha1 acid glycoprotein) and/or third spacing (e.g., ascites) will alter the volume of distribution and half-life of drugs. Furthermore, the metabolism of these drugs may also be altered by decreased hepatic functional capacity and greater tissue deposition, potentially increasing their duration of action. It is important to realize that hepatic blood flow is a key limiting factor in metabolism, especially for drugs with large extraction ratios. Obviously, decreased functional hepatic mass also plays a role but a drug will only be metabolized if blood carries it to the liver. Consequently, maintenance of normal systemic ABP and vascular volume are important for these patients. The use of colloids in conjunction with crystalloids may be necessary to increase colloidal osmotic pressure in hypoproteinemic animals. Hypoglycemia is commonly seen in hepatic patients and blood glucose should be measured perioperatively and, when necessary, dextrose infused to maintain normoglycemia.
Liver biopsy or excision
Liver biopsies may be necessary to diagnose hepatic disease. Samples can be obtained by ultrasound-guided TruCut needles, laparoscopy, and laparotomy. Also, laparoscopy and laparotomy can be used for the excision of abscesses and neoplasias. Knowledge of the specific implications of each technique can help the anesthetist prevent, anticipate, and resolve possible problems that may emerge during the procedure.
Ultrasound-guided biopsy
TruCut biopsies can sometimes be accomplished with sedation alone. For this technique the patient needs to lie still and not react to puncture. There is a chance that bleeding will occur so the technique is not advisable in animals with coagulopathy. The patient’s clinical signs and laboratory results, in addition to its demeanor and mentation, will determine drug and dose selection. Benzodiazepines are often used in critically ill patients due to their minimal cardiovascular effects, rapid elimination, and potential reversibility. Administration of midazolam (0.2–0.5mg/kg, intramuscularly [IM]) in combination with an opioid is frequently adequate to facilitate ultrasound-guided biopsies in mildly to severely obtunded patients. The opioids most commonly used in these combinations are butorphanol (0.2–0.4mg/kg), methadone (0.3–0.5mg/ kg), hydromorphone (0.05–0.2mg/kg), and oxymorphone (0.05–0.2mg/kg). Although usually unnecessary, flumazenil can be administered to reverse the sedative effects of benzodiazepines. In hepatic insufficiency, opioids often cause more pronounced sedation and side effects than in the healthy patient. For this reason, in the absence of pain, naloxone is administered to improve mentation and ventilation when sedation is no longer required or desirable. Even when only sedated, monitoring of the compromised patient is extremely important. Because of the variable degree of hepatic dysfunction and accompanying changes in pharmacokinetics, drug effects can be unpredictable. Pulse oximetry combined with evaluation of respiratory rate, pulse quality, capillary refill time, and mucous membrane color should be considered minimal monitoring standards during these procedures. ABP monitoring can be helpful to guide the initial choices of crystalloid and/or colloid fluid therapy. Oxygen supplementation may be advisable.
Laparoscopy
Laparoscopy is a less invasive alternative to laparotomy and is commonly used for liver biopsies and staging of liver neoplasia. Besides the obvious possibility of bleeding and difficulty in achieving hemostasis, especially in the presence of coagulopathies, this procedure introduces a new set of problems related to insufflation of gases into the abdomen that must be addressed. The infusion of carbon dioxide to improve visualization will decrease venous return and ventilation. The former is caused by the increase in intra-abdominal pressure by the pneumoperitoneum. The latter is caused by absorption of infused CO2 in combination with cranial displacement of the diaphragm due to increased abdominal pressure. The compression on the diaphragm will make it more difficult for the spontaneously breathing animal to ventilate and will decrease pulmonary functional residual capacity. The sum of these cardiopulmonary effects will also alter ventilation and perfusion relationships. Therefore, intermittent positive pressure ventilation (IPPV) is required to maintain adequate oxygenation and ventilation. IPPV can be achieved manually or by using a mechanical ventilator. The anesthetist should also monitor the intra-abdominal pressure, which should range from 10 to 16 mm Hg, as this can also significantly affect cardiac output.47 Monitoring of ABP is important as an indirect predictor of tissue perfusion and assessment of the effect of intra-abdominal pressure on venous return. A reversed Trendelenburg position (i.e., with the head higher than the abdomen) can help to minimize the effect of CO2 infusion on ventilation. Arterial blood gases are useful to evaluate patient ventilation and oxygenation status. Capnography, although helpful, can be misleading because the difference between end-tidal carbon dioxide (ETCO2) and PaCO2 can be significantly increased by ventilation/perfusion mismatching in some of these patients.
Laparotomy
Laparotomy is the most traditional approach for liver biopsies, abscesses, and neoplasia excisions. Besides concerns already mentioned for hepatic patients in particular, including hypoglycemia, hypoproteinemia, coagulopathies, and decreased drug metabolism, universal complications associated with any general anesthetic episode must also be taken in consideration. Hypothermia and hypoventilation are common in these cases and should be monitored and addressed by appropriate use of active warming devices and IPPV, respectively.
Portosystemic shunts
Portosystemic shunts are defined as anomalous vessels that allow blood flowing from the stomach, intestines, pancreas, and spleen to gain access to the central circulation without first passing through the liver. The blood bypassing the liver allows substances normally metabolized there (e.g., ammonia, mercaptans, short-chain fatty acids, etc.) to accumulate in the systemic circulation. Because blood flow to the liver is decreased, hepatotropic substances are not delivered and the liver fails to develop normally. Therefore, any and all of the key hepatic functions may be decreased as with any other form of liver disease. Portosystemic shunt patients may present with hypoglycemia, hypoal-buminemia, coagulopathies, and altered pharmacokinetics and pharmacodynamics of substances metabolized and excreted by the liver. HE and seizures may also be seen in these cases. Flumazenil has been used to improve mental status in humans with HE due to the possible involvement of intrinsic benzodiazepine-like compounds in this syndrome. Whether similar improvements occur in veterinary HE patients with flumazenil administration has not been verified.
Interventional radiology
This technique uses fluoroscopy to guide an intravenous catheter to the site where an anomalous vessel joins the systemic circulation. An auto-expandable stent is placed in the caudal vena cava next to the anomaly insertion. This stent will prevent the thrombotic coils deployed into the shunt vessel from becoming lost in the systemic circulation if they dislodge.48 The challenge for the anesthetist is to ensure complete immobilization of the patient at the critical stage of stent placement. During general anesthesia, an NMB can be administered to facilitate this if mechanical ventilation is available. Atracurium and cisatracurium can be used in boluses or constant rate infusions to maintain paralysis. In some situations, the surgeon may require an inspiratory hold to decrease patient abdominal movement during critical periods (e.g., measuring the anomalous vessel and placement of the stent). Hypothermia is a common problem and the use of a radiolucent heating pad is essential. Monitoring of cardiovascular (ABP and central venous pressure [CVP]) and respiratory parameters (ETCO2 and SpO2) is essential. Invasive ABP monitoring is ideal, but not mandatory, and CVP can be measured by connecting a sterile electronic transducer to the jugular catheter used by the surgeon to place the stent. The CVP will help assess patient blood volume and guide fluid therapy. As in other hepatic patients, colloidal and inotropic support may be needed. The anesthetist should be sure to restore vascular volume with crystalloids and colloids before inotropic administration. Dopamine (3–12mcg/kg/min) and dobutamine (2–10mcg/kg/min) are the most commonly used inotropes in veterinary medicine and can be used in these patients. Caution should be used as these drugs can cause cardiac arrhythmias.
Neuromuscular blockade should be terminated as soon as it is no longer necessary. Patients should be monitored for re-curarization. Reversal of nondepolarizing NMBs with an acetylcholinesterase inhibitor (e.g., neostigmine) is indicated even though spontaneous ventilatory efforts may be observed.
Laparotomy
All of the same concerns described for liver biopsies apply here. In addition, hemorrhage is a potential risk especially during intrahepatic shunt repair. Decreases in venous return should be monitored by the anesthetist (using CVP or by evaluating the character of the ABP waveform), as inadvertent compression of the abdominal vena cava by the surgeon is possible. Effective communication between the anesthetist and surgeon is extremely important.
Revised from “Hepatic Disease” by Stephen A. Greene and Steven L. Marks in Lumb and Jones’ Veterinary Anesthesia and Analgesia, Fourth Edition.
1. Guedes A.G., Papich M.G., Rude E.P., et al. Comparison of plasma histamine levels after intravenous administration of hydromorphone and morphine in dogs. J Vet Pharmacol Ther 30(6): 516–522, 2007.
2. Robinson E.P., Faggella A.M., Henry D.P., et al. Comparison of histamine release induced by morphine and oxymorphone administration in dogs. Am J Vet Res 49(10): 1699–1701, 1988.
3. Thompson W.L., Walton R.P. Elevation of plasma histamine levels in the dog following administration of muscle relaxants, opiates and macromolecular polymers. J Pharmacol Exp Ther 143: 131–136, 1964.
4. Lagerkranser M., Andreen M., Irestedt L. Central and splanchnic haemodynamics in the dog during controlled hypotension with sodium nitroprusside. Acta Anaesthesiol Scand 28(1): 81–86, 1984.
5. Höhne C., Donaubauer B., Kaisers U. Opioids during anesthesia in liver and renal failure. Anaesthesist 53(3): 291–303, 2004.
6. Dershwitz M., Hoke J.F., Rosow C.E., et al. Pharmacokinetics and pharmacodynamics of remifentanil in volunteer subjects with severe liver disease. Anesthesiology 84(4): 812–820, 1996.
7. Ludders J.W., Reitan J.A., Martucci R., et al. Blood pressure response to phenylephrine infusion in halothane-anesthetized dogs given acetylpromazine maleate. Am J Vet Res 44(6): 996–999, 1983.
8. Hitt M.E., Hanna P., Singh A. Percutaneous transabdominal hepatic needle biopsies in dogs. Am J Vet Res 53(5): 785–787, 1992.
9. Boström I., Nyman G., Kampa N., et al. Effects of acepromazine on renal function in anesthetized dogs. Am J Vet Res 64(5): 590–598, 2003.
10. Barr S.C., Ludders J.W., Looney A.L., et al. Platelet aggregation in dogs after sedation with acepromazine and atropine and during subsequent general anesthesia and surgery. Am J Vet Res 53(11): 2067–2070, 1992.
11. Aronson L.R., Gacad R.C., Kaminsky-Russ K., et al. Endogenous benzodiazepine activity in the peripheral and portal blood of dogs with congenital portosystemic shunts. Vet Surg 26: 189, 1997.
12. Maddison J.E. Newest insights into hepatic encephalopathy. Eur J Compar Gastroenterol 5: 17, 2000.
13. Als-Nielsen B., Gluud L.L., Gluud C. Benzodiazepine receptor antagonists for hepatic encephalopathy. Cochrane Database Syst Rev (2): CD002798, 2004.
14. Jones E.A., Yurdaydin C., Basile A.S. The role of endogenous benzodiazepines in hepatic encephalopathy: animal studies. Alcohol Alcohol Suppl 2: 175–180, 1993.
15. Nishiyama T., Hirasaki A., Toda N., et al. Pharmacokinetics of midazolam in patients with liver damage for hepatectomy. Masui 42(6): 871–875, 1993.
16. Bozkurt P., Kaya G., Süzer O., et al. Diazepam serum concentration-sedative effect relationship in patients with liver disease. Middle East J Anesthesiol 13(4): 405–413, 1996.
17. Kuo W.C., Keegan R.D. Comparative cardiovascular, analgesic, and sedative effects of medetomidine, medetomidine-hydromorphone, and medetomidine-butorphanol in dogs. Am J Vet Res 65(7): 931–937, 2004.
18. Flacke W.E., Flacke J.W., Bloor B.C., et al. Effects of dexmedetomidine on systemic and coronary hemodynamics in the anesthetized dog. J Cardiothorac Vasc Anesth 7(1): 41–49, 1993.
19. Lawrence C.J., Prinzen F.W., de Lange S. The effect of dexmedetomidine on nutrient organ blood flow. Anesth Analg 83(6): 1160, 1996.
20. Baratta M.T., Zaya M.J., White J.A., et al. Canine CYP2B11 metabolizes and is inhibited by anesthetic agents often co-administered in dogs. J Vet Pharmacol Ther 33(1): 50–55, 2010.
21. Flancbaum L., Alden S.M., Trooskin S.Z. Use of cholescintigraphy with morphine in critically ill patients with suspected cholecystitis. Surgery 106(4): 668–673; discussion 673–4. 1989.
22. Tegeder I., Lotsch J., Geisslinger G. Pharmacokinetics of opioids in liver disease. Clin Pharmacokinet 37(1): 17–40, 1999.
23. Bower S., Sear J.W., Roy R.C. Effects of different hepatic pathologies on disposition of alfentanil in anesthetized patients. Br J Anaesth 68(5): 462–465, 1992.
24. Chauvin M., Ferrier C., Haberer J.P., et al. Sufentanil pharmacokinetics in patients with cirrhosis. Anesth Analg 68(1): 1–4, 1989.
25. Navapurkar V.U., Archer S., Gupta S.K., et al. Metabolism of remifentanil during liver transplantation. Br J Anaesth 81: 881–886, 1998.
26. Dershwitz M., Hoke J.F., Rosow C.E., et al. Pharmacokinetics and pharmacodynamics of remifentanil in volunteer subjects with severe liver disease. Anesthesiology 84: 812–820, 1996.
27. Brainard B.M., Meredith C.P., Callan M.B., et al. Changes in platelet function, hemostasis, and prostaglandin expression after treatment with nonsteroidal anti-inflammatory drugs with various cyclooxygenase selectivities in dogs. Am J Vet Res 68(3): 251–257, 2007.
28. Thomson I.A., Fitch W., Hughes R.L., et al. Effects of certain I.V. anaesthetics on liver blood flow and hepatic oxygen consumption in the greyhound. Br J Anaesth 58: 69–80, 1986.
29. Thomson I.A., Fitch W., Campbell D., et al. Effects of ketamine on liver blood flow and hepatic oxygen consumption: studies in the anaesthetized greyhound. Acta Anaesthesiol Scand 32: 10–14, 1988.
30. Wouters P.F., Van de Velde M.A., Marcus M.A.E., et al. Hemodynamic changes during induction of anesthesia with eltanolone and propofol in dogs. Anesth Analg 81: 125–131, 1995.
31. Haberer J.P., Audibert G., Saunier C.G., et al. Effect of propofol and thiopentone on regional blood flow in brain and peripheral tissues during normoxia and hypoxia in the dog. Clin Physiol 13(2): 197–207, 1993.
32. Zoran D.L., Riedesel D.H., Dyer D.C. Pharmacokinetics of propofol in mixed breed dogs and greyhounds. Am J Vet Res 54: 755, 1993.
33. Sams L., Braun C., Allman D., et al. A comparison of the effects of propofol and etomidate on the induction of anesthesia and on cardiopulmonary parameters in dogs. Vet Anaesth Analg 35(6): 488–494, 2008.
34. Taylor J.D., Richards R.K., Tabern D.L. Metabolism of S35 thiopental (pentothal): chemical and paper chromatographic studies of S35 excretion by the rat and monkey. J Pharmacol Exp Ther 104: 93, 1952.
35. Sams R.A., Muir W.W. Effects of phenobarbital on thiopental pharmacokinetics in greyhounds. Am J VetRes 49(2): 245–249, 1988.
36. Nebauer A.E., Doenicke A., Hoernecke R., et al. Does etomidate cause haemolysis? Br J Anaesth 69(1): 58–60, 1992.
37. Ko J.C.H., Thurmon J.C., Benson G.J., et al. Acute haemolysis associated with etomidate-propylene glycol infusion in dogs. J Vet Anaesth 20: 92, 1993.
38. Kaka J.S., Hayton W.L. Pharmacokinetics of ketamine and two metabolites in the dog. J Pharmacokinet Biopharm 8(2): 193–202, 1980.
39. Frink E.J., Morgan S.E., Coetzee A., et al. The effects of sevoflurane, halothane, enflurane, and isoflurane on hepatic blood flow and oxygenation in chronically instrumented greyhound dogs. Anesthesiology 76: 85–90, 1992.
40. Hartman J.C., Pagel P.S., Proctor L.T., et al. Influence of desflurane, isoflurane and halothane on regional tissue perfusion in dogs. Can J Anaesth 39: 877–887, 1992.
41. Van Miert M.M., Eastwood N.B., Boyd A.H., et al. The pharmacokinetics and pharmacodynamics of rocuronium in patients with hepatic cirrhosis. Br J Pharmacol 44(2): 139–144, 1997.
42. Lebrault C., Berger J.L., D’Hollander A.A., et al. Pharmacokinetics and pharmacodynamics of vecuronium (ORG NC 45)in patients with cirrhosis. Anesthesiology 62: 601–605, 1985.
43. Devlin J.C., Head-Rapson A.G., Parker C.J. Pharmacodynamics of mivacurium chloride in patients with hepatic cirrhosis. Br J Anaesth 71: 227–231, 1993.
44. De Wolf A.M., Freeman J.A., Scott V.L., et al. Pharmacokinetics and pharmacodynamics of cisatracurium in patients with end-stage liver disease undergoing liver transplantation. Br J Anaesth 76: 624–628, 1996.
45. Ward S., Neill E.A. Pharmacokinetics of atracurium in acute hepatic failure (with acute renal failure). BrJ Anaesth 55: 1169–1172, 1983.
46. Kisor D.F., Schmith V.D. Clinical pharmacokinetic of cisatracurium besylate. Clin Pharmacokinet 36: 27–40, 1999.
47. Mayhew P.D. Advanced Laparoscopic Procedures (Hepatobiliary, Endocrine) in Dogs and Cats. Vet Clin North Am Small Anim Pract 39(5): 925–939, 2009.
48. Bussadori R., Bussadori C., Millan L., et al. Transvenous coil embolisation for the treatment of single congenital portosystemic shunts in six dogs. Vet J 176(2): 221–226. Epub 2007 Apr 26, 2008.
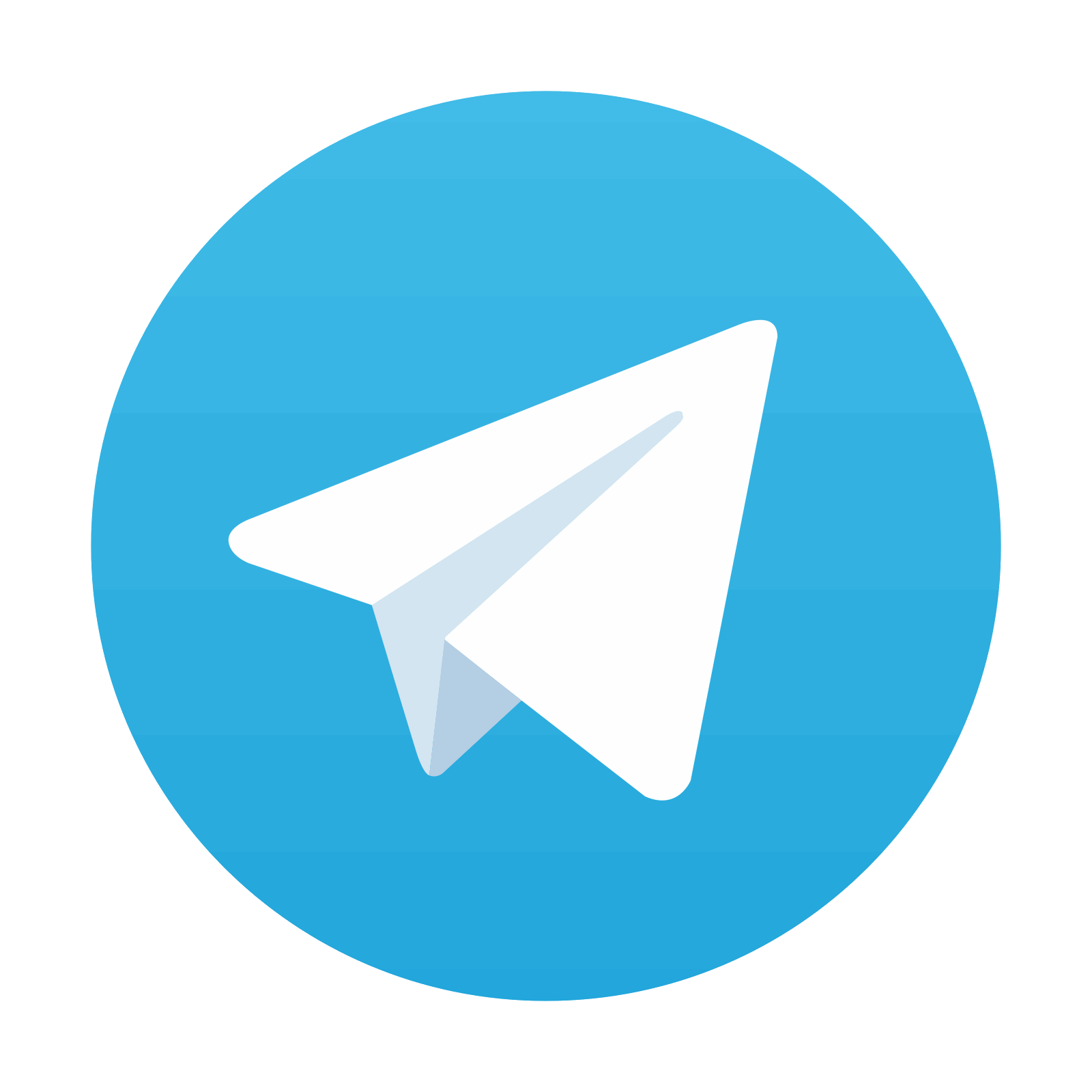
Stay updated, free articles. Join our Telegram channel

Full access? Get Clinical Tree
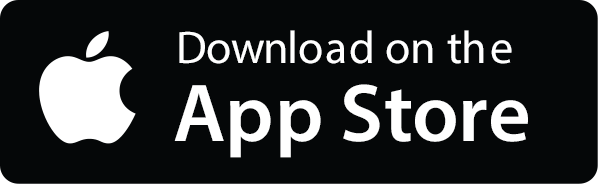
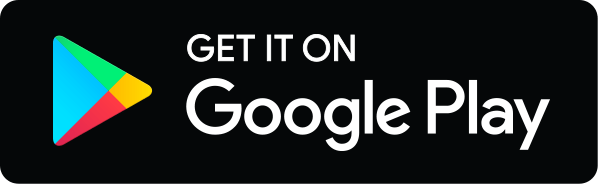