7 Rose M. McMurphy, Elizabeth G. Davis, Amy J. Rankin, Daniel A. Frese, Barbara J. Lutjemeier, and Michael J. Kenney The sympathetic nervous system (SNS) influences the regulation of most organ systems and plays a critical role in regulating physiological homeostasis under basal conditions and in response to acute and sustained stressors. Changes in physiological function are initiated and sustained by altering the level of efferent sympathetic nerve outflow, which in turn affects the release of norepinephrine (NE) from postganglionic nerve terminals. Sympathetic nerve activity mediates NE release from nerve terminals, and the released NE binds to and activates adrenergic receptors located on postsynaptic effector tissues and at presynaptic sites. In a similar fashion, central activation of sympathetic nerve activity directed to the adrenal medulla initiates the release of epinephrine (EPI) and some NE from this gland, which circulates in the blood and binds to and activates adrenergic receptors in target tissues. Drugs that mimic the pharmacological and physiological actions of the endogenous catecholamines are classified as sympathomimetic drugs, that is their effects are mediated by activation of adrenergic receptors located on effector cells and tissues. One framework for classifying the functionality of adrenergic receptor agonists is centered on their general pharmacological mechanism of action (Figure 7.1). Three classifications are often considered. The first group, which includes the endogenous catecholamines (e.g., NE and EPI) and many sympathomimetic drugs (e.g., phenylephrine, dobutamine), are classified as direct agonists. These neurotransmitters and drugs bind directly to and activate adrenergic receptors (Figure 7.1). Many adrenergic agonists used in clinical medicine are direct adrenergic receptor agonists. A second group is classified as indirect-acting agonists because they mediate physiological responses via a pharmacological mechanism of action that involves increasing the synaptic levels of endogenous catecholamines, thereby enhancing the availability of endogenous catecholamines to bind to adrenergic receptors. This effect can be achieved by three different mechanisms of action: (i) reducing the metabolic breakdown of catecholamines by pharmacologically blocking or antagonizing endogenous enzymes involved in the metabolism of NE and EPI (e.g., monoamine oxidase inhibitors); (ii) inhibiting the physiological processes involved in the reuptake of NE from the synaptic space into postganglionic sympathetic nerve terminals (e.g., cocaine and tricyclic antidepressants); and (iii) enhancing the release of catecholamines from postganglionic sympathetic nerve terminals (e.g., tyramine). Some drugs (e.g., ephedrine) demonstrate the capability to directly activate adrenergic receptors as well as augment the release of NE from adrenergic nerve terminals, and these drugs are classified as mixed-acting adrenergic agonists (Figure 7.1). In a conceptual context, the physiological responses produced by direct, indirect, and mixed-acting agonists are similar to responses produced by activating central sympathetic neural circuits, increasing the level of efferent sympathetic nerve outflow, and inducing the release of NE from postganglionic sympathetic nerves. Figure 7.1 Framework for classifying the functionality of catecholamines and sympathomimetics based on pharmacological mode of action, that is direct agonists, indirect agonists, and mixed agonists. COMT, catechol-O-methyltransferase; MAO, monoamine oxidase. The capacity of sympathomimetic drugs to produce physiological responses consistent with functional patterns produced by SNS activation depends on the similarity of the chemical structure of sympathomimetic drugs to that of NE and EPI. The parent compound of sympathomimetic amines is β-phenylethylamine, which consists of a benzene ring and an ethylamine side chain. Chemical substitutions can be made on the aromatic ring, on the α and β carbons atoms, and on the terminal amino group to produce compounds with sympathomimetic activity. The endogenous adrenergic receptor agonists EPI, NE, and dopamine, as well as the synthetic sympathomimetic isoproterenol, contain a hydroxyl group on the 3 and 4 positions of the benzene ring. The 3,4-dihydroxybenzene structure is also known as catechol; therefore sympathomimetic amines that contain this nucleus are known as catecholamines. For the most part, the catechol nucleus is required for maximum α- and β-adrenergic receptor potencies, and modifications in phenylethylamine mediate changes in the affinity of drugs for specific adrenergic receptors, and affect the intrinsic ability to activate these receptors. Removal of one or both hydroxyl groups from the aromatic ring reduces β-adrenergic receptor activity. For example, phenylephrine, a specific α1-adrenergic receptor agonist, is identical in structure to EPI, a mixed α-β-adrenergic receptor agonist, except for the lack of one hydroxyl group on the ring (Table 7.1 ). Substitution on the β-carbon atom of the side chain produces a compound with reduced central nervous system (CNS) effects, whereas substitution on the α-carbon atom produces a drug that is generally more resistant to oxidation by monoamine oxidase (MAO). The α- and β-agonistic properties of various drugs are affected by alkyl substitutions on the amino group. Collectively, this information supports the idea that pharmacological profiles of specific agonists differ depending upon chemical structure. The chemical structures and related pharmacological characteristics of the endogenous catecholamines and several adrenergic drugs are summarized in Table 7.1 . Table 7.1 Chemical structures of catecholamines and selected sympathomimetic drugs Physiological responses produced by catecholamines and sympathomimetic drugs are initiated by the binding of agonists to adrenergic receptors located on the cell surface (Wilson-Pauwels et al., 1997; Yamada and Ito, 2001; Cazzola et al., 2012; Lei, 2014; Herring, 2015). Adrenergic receptors are G protein-coupled receptors (GPCRs) (Calebiro et al., 2010; Jalink and Moolenaar, 2010; Ambrosio et al., 2011; Vischer et al., 2011; Latek et al., 2012; Duc et al., 2015). G proteins are classified by their specific subunits, and select G proteins regulate specific effector proteins and interact with select molecular pathways. Membrane and intracellular processes associated with GPCRs have been previously introduced in Chapter 6. The β-adrenergic receptor family is composed of three different subtypes (β1, β2, and β3). Activation of β receptors, regardless of subtype, results in stimulation of adenylyl cyclase and increased cAMP, mediated via the stimulatory coupling protein Gs (Table 7.2 ). β-adrenergic receptors (β1, β2, and β3) are expressed in numerous tissues and organs (Table 7.3 ), although for a given tissue a specific receptor subtype may be more prominently expressed when compared with other receptor subtypes, thereby providing the tissue or organ with a relatively specific β-adrenergic subtype signature. For example, cardiac functions, such as increased heart rate and enhanced cardiac contractility, are medicated to a prominent extent by activation of cardiac β1-receptors, whereas relaxation of bronchial smooth muscle involves activation of bronchial smooth muscle β2-adrenergic receptors. Table 7.2 Adrenergic receptor types and subtypes, selected agonist and antagonist drugs, primary G proteins involved in mediating cellular responses to activation of specific adrenergic receptors, and typical intracellular effects secondary to adrenergic receptor ligand binding PLC, phospholipase C; PLA, phospholipase A; IP3, inositol-1,4,5-triphosphate; DAG, diacylglycerol; cAMP, cyclic AMP. Table 7.3 Anatomical distribution of adrenergic receptor subtypes and general physiological effectors produced in response to activation of specific adrenergic receptors β-adrenergic receptors are expressed in smooth muscle at multiple anatomical sites, including: pulmonary airways (β2 receptors), vascular smooth muscle (β2 receptors), and the detrusor muscle of the bladder (β2 and β3 receptors). Activation of β receptors in these tissues mediates smooth muscle relaxation contributing to bronchodilation, vasodilation of vascular smooth muscle with accompanying reduced vascular resistance, and relaxation of the bladder body. Smooth muscle relaxation secondary to activation of β-adrenergic receptors may be mediated via processes that include the phosphorylation of myosin light-chain kinase to an inactive form. In many species the innervation of vascular smooth muscle β2-adrenergic receptors by postganglionic sympathetic nerves is rather sparse, suggesting that the primary activation of these receptors is mediated by circulating EPI released from the adrenal medulla. β-adrenergic receptors (generally thought to be β2 receptors) are also located at presynaptic sites on postganglionic nerve terminals and, in general, activation of presynaptic β2-adrenergic receptors facilitates the release of NE. The α1-receptor family includes three subgroups: α1A, α1B, and α1D. Binding of an agonist to α1-adrenergic receptors, regardless of subtype, activates phospholipase C, mediated via activation of a Gq coupling protein, which leads in many cases to the formation of inositol 1,4,5-trisphosphate (IP3) and diacylglycerol, and multiple functional effects including the release of intracellular Ca2+ stores (Table 7.2 ). Although the distribution (Table 7.3 ) and activation of different α1-adrenergic receptor subtypes provides the substrate for a diverse profile of physiological responses, the functional signature of α1-adrenergic receptor activation is smooth muscle contraction, including contraction of vascular smooth muscle, the radial muscle of the iris (iris dilator muscle), and smooth muscle in the genitourinary system. Generally, α1-adrenergic receptors located in vascular smooth muscle are in close proximity to postganglionic sympathetic nerve endings, therefore they are considered innervated vascular receptors. The α2-receptor family includes three subgroups: α2A, α2B, and α2C. In many targets the physiological responses to activation of α2 receptors involves the coupling of these receptors to the inhibitory regulatory protein Gi (Table 7.2 ), resulting in inhibition of adenylyl cyclase activity and reduced intracellular cAMP levels. It is known that other signaling pathways, separate from the inhibition of adenylyl cyclase, are involved in mediating intracellular processes to α2-receptor activation. α2-adrenergic receptors are expressed in a variety of cells and tissues (Table 7.3 ), including vascular smooth muscle, thromobocytes, endothelial cells, and in the CNS. α2-adrenergic receptors are located on the terminals of postganglionic sympathetic nerve fibers, and activation of these presynaptic adrenergic receptors by endogenous catecholamines and other sympathomimetics reduces NE release from nerve terminals. By binding to and activating specific receptors at both central and peripheral sites, the endogenous catecholamine dopamine produces numerous physiological effects. Activation of the dopamine1 (D1) receptor is generally associated with increased cAMP, whereas activation of dopamine2 (D2) receptors can inhibit adenylyl cyclase activity. The breadth and diversity of (i) adrenergic receptor types and subtypes, (ii) G-proteins, and (iii) second messenger systems provides the cellular and molecular substrate for mediating the myriad number of physiological responses produced by activation of the SNS. In addition, many adrenergic receptor agonists demonstrate a level of selectivity for specific adrenergic receptors. An individual endogenous catecholamine or sympathomimetic drug may exhibit a higher affinity or selectivity for one or more subtypes of adrenergic receptors. Receptor selectivity provides an important framework for understanding target organ effects of endogenous catecholamines and sympathomimetic drugs. However, receptor selectivity for a given agonist is generally not absolute; that is, at higher concentrations a given drug or catecholamine may interact with and activate other subtypes or classes of adrenergic receptors, providing the functional basis for considering a spectrum of relative receptor affinities for most adrenergic agonists. Examples of relative receptor affinities for endogenous catecholamines of select adrenergic drugs are listed in Table 7.4. EPI is an agonist at α1-, α2-, β1-, and β2-adrenergic receptors, whereas NE is an agonist at α1-, α2-, and β1-adrenergic receptors, with less potent effects on β2-adrenergic receptors. Isoproterenol is a β1– and β2-adrenergic receptor agonist whereas phenylephrine is a selective α1-adrenergic receptor agonist (Table 7.4 ). Table 7.4 Classification of adrenergic receptor agonists and their relative selectivity for specific adrenergic receptors The anatomical location of specific adrenergic receptor types and subtypes plays a key role in mediating the functional/physiological responses produced by a given drug or catecholamine. Pharmacological interactions between specific agonists and adrenergic receptors, as well as the profile of physiological responses produced by agonist–receptor interactions, are diverse and dynamic and are influenced by a number of factors, including: the tissue distribution of adrenergic receptors; the number of adrenergic receptors expressed at specific sites; interactions between the sympathetic and parasympathetic nervous systems at target sites; specific pathophysiological and disease states; the background level of sympathetic nerve activity; and levels of endogenous catecholamines and/or sympathomimetic drugs. Regarding the latter, prolonged exposure of adrenergic receptors to specific agonists reduces the responsiveness of these receptors to agonist activation, producing a progressive attenuation in the tissue’s capacity to facilitate physiological responses. This physiological phenomenon is known as desensitization of adrenergic receptors. Multiple cellular and molecular mechanisms likely play a role in mediating adrenergic receptor desensitization, including changes in the number of adrenergic receptors and in intracellular signaling mechanisms. The pivotal contributions of adrenergic receptor selectivity and tissue distribution in mediating physiological responses to sympathomimetic drugs can be highlighted by comparing the effects of specific endogenous catecholamines and sympathomimetic drugs on various physiological regulatory systems, including cardiovascular regulation. In this section the cardiovascular effects (blood pressure, heart rate, myocardial contractility, cardiac output) produced by the administration of phenylephrine (selective α1-adrenergic receptor agonist), NE and EPI (endogenous catecholamines), and isoproterenol (β1– and β2-adrenergic receptor agonist) are compared, with an emphasis on integrating and explaining how differences in their affinity or selectivity for specific α- and β-adrenergic receptors, and the primary anatomical location of specific adrenergic receptors, contribute to the observed cardiovascular responses (Figure 7.2). Arterial blood pressure is determined by the flow of blood through a vessel, which is ultimately determined by cardiac output and by the diameter or tone of that vessel, represented as peripheral vascular resistance. Information regarding the specific adrenergic receptors that play a role in cardiovascular regulation, their primary anatomical distributions in the vasculature and heart, the specific cardiovascular responses produced by their activation, and the order of potency of EPI, NE, isoproterenol, and phenylephrine are summarized in Table 7.5. This information provides the background required for explaining differences in cardiovascular response profiles to specific adrenergic agonists (Figure 7.2). In the examples described in this section each of the adrenergic receptor agonists have been administered as bolus injections over a short period of time. Table 7.5 Selected adrenergic receptors that play a role in cardiovascular regulation, their primary distributions in the vasculature and heart, general cardiovascular responses produced by their activation, and the selectivity of agonists (EPI, NE, ISO, and PE) for the specific adrenergic receptors EPI, epinephrine; ISO, isoproterenol; NE, norepinephrine; PE, phenylephrine. Figure 7.2 Schematic showing cardiovascular effects in response to the intravenous administration of four adrenergic agonists (phenylephrine (PE), norepinephrine (NE), epinephrine (EPI), and isoproterenol (ISO)) characterized by different relative receptor affinity profiles. Schematic representations of approximate equivalent doses of these endogenous catecholamines and sympathomimetic amines on blood pressure (BP), femoral blood flow (FBF), renal blood flow (RBF), peripheral vascular resistance (PR), myocardial contractile force (MCF), heart rate (HR), and cardiac output (CO). See text for explanation of the cardiovascular responses. Phenylephrine is a potent and selective agonist at α1-adrenergic receptors. α1-receptors are expressed in numerous vascular beds (e.g., visceral organs, skin, and skeletal muscle) and their activation by phenylephrine administration produces vasoconstriction of arterial smooth muscle, which in turn increases peripheral vascular resistance and reduces femoral blood flow and renal blood flow (Figure 7.2). In addition, phenylephrine administration produces venoconstriction, which reduces venous capacitance and increases venous return. The increase in arterial blood pressure is primarily due to the marked α1-mediated increase in peripheral vascular resistance. The abrupt increase in arterial blood pressure secondary to phenylephrine administration activates the arterial baroreflex, which elicits a reflex-mediated increase in cardiac vagal nerve activity and a reduction in heart rate (bradycardia). α1-receptors are not prominently expressed on cardiac cells involved in the regulation of cardiac rate or myocardial contractility; therefore phenylephrine administration does not elicit substantial direct effects on heart rate or cardiac output. However, from an integrative perspective phenylephrine administration does affect several of the physiological indices involved in the regulation of cardiac output, including: increased peripheral vascular resistance and afterload; increased venous return to the heart; and reduced heart rate via activation of the baroreflex. Norepinephrine is an agonist at α-adrenergic (α1 and α2) and β1-adrenergic receptors (and to a much lesser extent to β2-adrenergic receptors). Activation of vascular α1-adrenergic receptors by NE increases peripheral vascular resistance, as evidenced by decreases in femoral and renal blood flow (Figure 7.2). In addition, NE administration produces venoconstriction, which reduces venous capacitance and increases venous return. The activation of α2-adrenergic receptors in vascular smooth muscle produces vasoconstriction and likely contributes to NE-induced increases in peripheral vascular resistance. β1-adrenergic receptors are expressed in the heart, and activation of cardiac β1-receptors by NE produces positive inotropic (increased cardiac contractility) and chronotropic (increased rate) effects. The increase in arterial blood pressure is mediated by combined vascular (α-adrenergic receptor-mediated vasoconstriction) and cardiac (increased cardiac output due to activation of β1-adrenergic receptor) effects. The marked NE-induced increase in arterial blood pressure activates the arterial baroreflex, which elicits a reflex-mediated increase in cardiac vagal nerve activity and a reduction in heart rate. The reflex bradycardia does not necessarily lead to a reduction in cardiac output because of the direct effect of NE to activate cardiac β1-receptors and increase myocardial contractility and stroke volume. Epinephrine is a potent agonist at α-adrenergic (α1 and α2) and β-adrenergic (β1 and β2) receptors, and activation of vascular β2-adrenergic receptors produces vasodilation. Therefore, EPI administration produces vasoconstriction and reduces blood flow in vascular beds that contain high concentrations of α-adrenergic receptors (e.g., visceral organs, cutaneous vessels), accounting for the EPI-induced reduction in renal blood flow (Figure 7.2). Some targets, such as the smooth muscle in the arterial vasculature supplying skeletal muscle, contain both β2– and α-adrenergic receptors, and EPI-induced activation of β2 receptors produces vascular vasodilation, whereas EPI-induced activation of α-adrenergic receptors produces vasoconstriction. In general, skeletal muscle vascular beds are often characterized by reduced peripheral vascular resistance in response to EPI administration (demonstrated as increased femoral blood flow in Figure 7.2), whereas EPI produces α-adrenergic receptor-mediated vasoconstriction in visceral vascular beds. Due to the competing vasoconstrictor and vasodilatory effects of EPI, the increase in peripheral vascular resistance to EPI administration is generally not as robust as that observed in response to NE administration (Figure 7.2). EPI is a prominent cardiac stimulant and activation of cardiac β1-adrenergic receptors increases cardiac contractility, heart rate, and cardiac output. The initial effect of EPI administration is often characterized by a rapid increase in cardiac output (cardiac β1-receptor activation), a marked increase in peripheral vascular resistance mediated by the activation of visceral α-adrenergic receptors, and an increase in arterial blood pressure, which in turn may elicit a baroreflex-mediated increase in cardiac vagal nerve activity and a transient reduction in heart rate. Subsequent activation of β2-adrenergic receptors as EPI is distributed to peripheral sites, such as skeletal muscle vascular beds, attenuates increases in peripheral vascular resistance and arterial blood pressure. Isoproterenol is a potent, nonselective β1– and β2-adrenergic receptor agonist that exerts little effect on α-adrenergic receptors; therefore blood pressure responses to this β-adrenoceptor agonist are a function of its effects on cardiac and peripheral vascular β-adrenergic receptors. The vascular response profile to isoproterenol administration is characterized by vascular vasodilation, an increase in blood flow to vascular beds that contain a high density of β2-adrenergic receptors (skeletal muscle, as supported by the increase in femoral blood flow), modest β2-adrenergic receptor-mediated vasodilation in visceral vascular beds (e.g., renal blood flow), and reduced peripheral vascular resistance (Figure 7.2). Because isoproterenol lacks affinity for α-adrenergic receptors, there is no pharmacological-induced vascular smooth muscle contraction; therefore isoproterenol produces a marked reduction in peripheral vascular resistance. Isoproterenol is a potent cardiac stimulant. Isoproterenol activation of cardiac β1-adrenergic receptors increases cardiac output by increasing heart rate, mediated by direct activation of the sinus node, and by increasing contractility of myocardial cells. It is generally considered that β1-adrenergic receptors are the predominate type of myocardial β-adrenergic receptor. Mean arterial blood pressure is typically reduced in response to isoproterenol secondary to the marked reduction in peripheral vascular resistance, despite the accompanying increase in cardiac output. Comparing cardiovascular responses to specific catecholamines and select sympathomimetic drugs supports the concept that relative receptor affinity, direct receptor effects, receptor distribution, and intrinsic reflex adjustments are key components for predicting drug-associated physiological response profiles for a variety of organs and regulatory systems. As stated previously, adrenergic receptors are distributed widely throughout many tissues and organ systems, and activation of these receptors facilitates a myriad of physiological responses. Selected clinical applications of sympathomimetics in specific conditions are described in this section as a framework for characterizing the pharmacodynamic effects of these drugs. A 15-year-old Quarter Horse mare presented with the clinical complaint of cough and increased abdominal effort during the expiratory phase of respiration. Physical examination revealed a heart rate of 44 beats per minute (normal; 36–44 beats per minute) and a respiratory rate of 20 breaths per minute (normal; 12–24 breaths per minute). Thoracic auscultation revealed increased bronchovesicular sounds and expiratory wheezes in all lung fields. In addition to an increased respiratory effort, the mare demonstrated prominent nostril flare (wide open nostrils) with expiration. Arterial blood gas analysis indicated hypoxemia as demonstrated by an arterial partial pressure of oxygen (Pa02) of 66 mmHg. Based on the clinical findings, an immediate treatment protocol included the administration of aerosolized albuterol (selective β2-adrenergic receptor agonist) (Rush et al., 1999). β2-adrenergic receptors are present in bronchial smooth muscle, and activation of these receptors by β2
Adrenergic Receptor Agonists and Antagonists
Classification of Adrenergic Agonists
Sympathomimetic Amines and Structure–Activity Relationships
Drug
β-phenylethylamine
…
H
H
H
β-phenylethanolamine
…
OH
H
H
Catecholamines
Dopamine
3-OH, 4-OH
H
H
H
Norepinephrine
3-OH, 4-OH
OH
H
H
Epinephrine
3-OH, 4-OH
OH
H
CH3
Isoproterenol
3-OH, 4-OH
OH
H
CH(CH3)2
Noncatecholamines
Phenylephrine
3-OH
OH
H
CH3
Tyramine
4-OH
H
H
H
Ephedrine
…
OH
CH3
CH3
Activation of Adrenergic Receptors and Cell Signaling
Receptor
Agonist
Antagonist
G Protein
Effects
α1-Adrenergic
Phenylephrine
Prazosin
Gq
Activation of PLA2, PLC, ↑ IP3 and DAG; common to all
α1A
Tamsulosin
α1B
α1D
α2-Adrenergic
Dexmedetomidine
Yohimbine Atipamezole
Gi
Inhibition of adenylyl cyclase, ↓ cAMP; common to all
α2A
α2B
α2C
β-Adrenergic
Isoproterenol
Propranolol
Gs
Stimulation of adenylyl cyclase, ↑ cAMP; common to all
β1
Dobutamine
Atenolol
β2
Terbutaline
Butoxamine
β3
Mirabegron
Dopaminergic
Dopamine
D1
Fenoldopam
Gs
Stimulation of adenylyl cyclase, ↑ cAMP
D2
Bromocriptine
Gi
↓ cAMP
Receptor
Tissue
Response
α1
Most vascular smooth muscle (innervated)
Contraction
Pupillary dilator muscle
Contraction (dilates pupil)
Splenic capsule
Contraction
Urethral smooth muscle
Contraction
α2
Postsynaptic CNS neurons
Numerous sites
Platelets
Aggregation
Adrenergic and cholinergic nerve terminals
Inhibits transmitter release
Selected vascular smooth muscle
Contraction
β1
Heart, juxtaglomerular cells
Increases force and rate of contraction; increases renin release
β2
Respiratory, uterine, and vascular smooth muscle
Smooth muscle relaxation
Liver
Gluconeogenesis
β3
Fat cells
Activates lipolysis
D1
Vascular smooth muscle
Dilates select blood vessels
D2
Autonomic nerve terminals
Modulates neurotransmitter release from nerve terminals
Adrenergic Receptor Selectivity and Tissue Distribution
Overview
Agonists
Receptor selectivity
Alpha agonists
Phenylephrine
α1 > α2 >>>>> β
Dexmedetomidine, Medetomidine, Xylazine
α2 > α1 >>>>> β
Mixed alpha and beta agonists
Norepinephrine
α1 = α2; β1 >> β2
Epinephrine
α1 = α2; β1 = β2
Beta agonists
Dobutamine
β1 > β2 >>>> α
Isoproterenol
β1 = β2 >>>> α
Albuterol, Terbutaline
β2 >> β1 >>>> α
Dopamine agonists
Dopamine
D1 = D2 >> β >> α
Cardiovascular Responses to Adrenergic Receptor Agonists: Effects of Adrenergic Receptor Selectivity and Distribution
Receptor
Tissue
Physiological response
Agonist selectivity
α
Blood vessels
Vasoconstriction
PE; EPI > NE >>> ISO
β1
Heart
Augmented inotropic and chronotropic effects
ISO > EPI ≥ NE
β2
Blood vessels
Vasodilation
ISO > EPI >>> NE
Clinical Applications of Adrenergic Receptor Agonists
Adrenoceptor Agonists and Respiratory Physiology
Stay updated, free articles. Join our Telegram channel

Full access? Get Clinical Tree
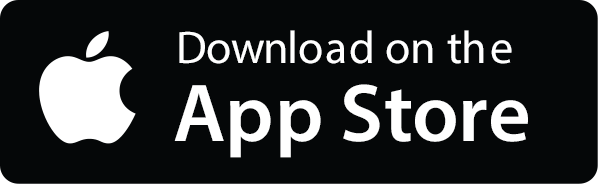
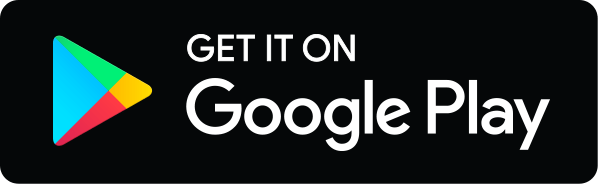