CHAPTER 8. Pregnancy Loss
OBJECTIVES
While studying the information covered in this chapter, the reader should attempt to:
■ Acquire an understanding of the factors that contribute to pregnancy loss in the equine embryonic, fetal, natal, and neonatal periods.
■ Acquire a working knowledge of the signs of placental and fetal infection.
■ Acquire a working knowledge of procedures to be followed to maximize the chance of diagnosing the cause of an abortion.
■ Acquire a working knowledge of the more common gestational abnormalities in the mare, including management of each condition.
STUDY QUESTIONS
1. Give the length of the embryonic, fetal, and neonatal periods of the horse.
2. List signs of embryonic loss in the mare, and discuss methods that can be used to confirm it.
3. Discuss management options for embryonic death in the mare.
4. List the causes of placental dysfunction in the mare.
5. List the more common infectious and noninfectious causes of abortion in the mare.
6. Explain why most aborted fetuses submitted for examination are 6 to 11 months of gestational age.
7. Explain how placentitis results in abortion.
8. Identify the routes of placental and fetal invasion by microorganisms.
9. Design a thorough diagnostic approach to an abortion problem on a broodmare farm.
10. Describe the more common gestational abnormalities in the mare, and discuss methods of diagnosis, treatment, and probable outcomes for each.
In a review of publications of pregnancy losses in horses, Ginther (1992) suggests an average expected loss rate of 18% when pregnancy diagnosis is done at 20 days after ovulation. He also notes that the loss rate per day should be expected to remain steady until approximately 60 days of gestation, after which loss rate per day should decline. Other reviewers have reported that equine pregnancy loss occurs with greater frequency early in pregnancy than later. Bain reported that, of pregnancies that were lost, 55% occurred by day 39 of gestation and 75% by day 49. Most authors agree that the rate of pregnancy loss apparently diminishes after day 60 to 75 of gestation. In a recent 5-year review of breeding records from a Quarter Horse–type herd, Texas workers reported an 11.0% pregnancy loss rate (79 of 715), with 42% of the losses occurring by 42 days of gestation. A similar proportion of losses occurred with the fall pregnancy examination (2 to 5 months of gestation), with 17% of losses occurring later (>5 months) in gestation (Figure 8-1). Within individual mares with early loss of pregnancy, subsequent pregnancy loss is reportedly not different from that in the rest of the mare population (i.e., pregnancy loss is no more likely to recur than in mares without previous pregnancy loss). In spite of this report, some mares seem to be predisposed to recurring pregnancy losses. In addition, pregnancy loss rates tend to gradually increase in broodmares older than 12 to 15 years of age. Thus, a widely held belief is that aged barren mares not only are less likely to become pregnant than maiden or foaling mares but are also more likely to lose the pregnancy.
Many genetic, maternal, and environmental factors can contribute to pregnancy loss during critical periods of development. These periods include the embryonic period (conception through organogenesis), the fetal period (completion of organogenesis through end of gestation), the natal period (birth), and the neonatal period (first 28 days of life). The susceptibility of the embryo to injurious agents varies with the stage of development. Cattle and sheep embryos less than 14 days of age (period of preattachment) are resistant to teratogens but susceptible to genetic mutations and chromosomal abnormalities. During the rest of the embryonic period, the embryo is highly susceptible to teratogens until this susceptibility begins to decrease as the various organ systems develop. The embryonic period in the equine includes the period from conception to 40 days of gestation (according to review by Ginther, 1992).
Limitations in accurate determination that conception has occurred prevent accurate assessment of the incidence of embryonic death in most animals. For the equine, the rate of pregnancy loss between days 15 and 50 after ovulation has been reported to be 10% to 15%. These estimates are based on losses that occur after early pregnancy diagnosis with transrectal ultrasonography. Estimates of earlier pregnancy loss (i.e., before ultrasonographic detection is possible at 10 to 14 days after ovulation) are more difficult to assess but have been obtained from flushing uteri on different days (7 to 10 days after ovulation) and comparing actual embryo recovery rates at those times with known fertilization rates obtained in other populations of mares (by determining whether ova are cleaved in the oviducts). Nonrecovered oocytes or embryos, a problem with any recovery methodology, add a measure of inaccuracy to such estimates.
Estimates for embryonic death rates in the equine vary from 5% to 24% and average 20% for conception to day 40 of gestation in some groups of fertile mares. Because fertilization rates are generally quite high in both fertile and subfertile mares (exceeding 80% to 90%), a significant cause of subfertility may be early embryonic death. New York researchers reported the incidence of embryonic death before day 14 of gestation to be 7 to 8 times as frequent for aged subfertile mares as for young fertile mares. Other investigators have found that older mares are more likely to produce defective oocytes that presumably, when fertilized, are more susceptible to embryonic death.
The nonviable embryo is resorbed or expelled from the uterus and is therefore seldom observed. If embryonic death occurs before maternal recognition of pregnancy, affected mares return to estrus at the usual time expected if the mares had failed to conceive (i.e., interestrus interval of 3 weeks). In such cases, early embryonic death goes unsuspected. When interestrus intervals are prolonged (e.g., beyond 24 to 25 days), embryonic death with delayed return to estrus can be suspected.
CAUSES OF EMBRYONIC DEATH
Possible causes of embryonic death include infectious agents, chromosomal abnormalities, teratogenic agents, immunologic reactions, genetic abnormalities, local oviductal or uterine disturbances, nutritional factors (particularly deficient dietary energy or protein intake), and temperature stress. Ball (1993) divides potential factors that contribute to embryonic mortality into three general areas: (1) maternal; (2) external; and (3) embryonic.
Maternal Factors
■ Endocrine: Low progesterone production from failure of maternal recognition of pregnancy, primary luteal deficiency, or uterine-induced luteolysis caused by endometrial irritation (e.g., endometritis).
■ Oviductal environment: Reduced levels of embryotrophic factors, elevated levels of embryotoxic factors, improper timing of oviductal transport; salpingitis.
■ Age: Increased embryonic death rates as mares age; attributed in part to age-related degeneration of the uterus and oocyte quality.
■ Lactation: If nutritional demands result in declining body condition; delayed uterine involution or persistent endometritis.
■ Age-related anatomic changes in the genital tract (such as those that lead to pneumovagina, urovagina, or a pendant uterus that drains poorly and accumulates fluid) can contribute to infection that compromises the fetal-placental unit, thereby leading to pregnancy loss.
External Factors
■ Stress: Hypothesized to decrease progesterone production, which may result in pregnancy loss.
■ Inadequate nutrition: Particularly when mares lose body condition in late gestation or early lactation.
■ Ingestion of toxins or infectious agents: Particularly those that have a predilection for the developing fetus. Consumption of Eastern tent caterpillars has been linked in mares in central Kentucky to a reproductive loss syndrome termed mare reproductive loss syndrome (MRLS).
Embryonic Factors
■ Small size, morphologic defects.
■ Embryos from aged subfertile mares have reduced survival rates when transferred to uteri of normal recipient mares.
■ Chromosomal abnormalities: From gamete aging or other causes.
DIAGNOSIS OF EMBRYONIC DEATH
In a thorough discussion of ultrasonographically detectable signs of impending embryonic loss, Newcombe (2000) suggests the following criteria for predicting its occurrence:
Small-for-Age Embryonic Vesicles
Embryonic vesicles are sometimes visible as early as days 10 to 11 after ovulation, at a diameter of 3 to 4 mm. Vesicle size (diameter, top to bottom) typically increases 3 to 4 mm in diameter each day until day 16, plateaus during days 17 to 25, and resumes increasing in diameter by 1.8 mm/day by day 28 (Ginther, 1986). Whenever the diameter of the vesicle (through 30 days) is retarded in development by more than 1 to 2 days, particularly when undersized on more than one examination, embryonic viability should be questioned (Figure 8-2). Colorado workers recently found that the percentage of examinations during which the vesicle was found to be undersized was significantly higher for abnormal conceptuses (44.4%) than for normal conceptuses (<1%). Some veterinary ultrasound machines are programmed with gestation tables that permit the practitioner to estimate gestational age based on ultrasonographic measurements, which is useful for determining whether conceptuses are small for gestational age.
Anembryonic Vesicles
On occasion, vesicles fail to develop an embryo (Figure 8-3). Such vesicles often are first noted to be small for gestational age when detected early in pregnancy before the embryo is visible with ultrasound (typically by days 20 to 21). If an embryo is not visible by days 24 to 26, embryonic viability should be questioned. Colorado workers have found the incidence of anembryonic vesicles to be 4.4%.
Retarded Development of the Embryo
Normal progression in embryo development, as outlined in Chapter 5, should be apparent during examination for pregnancy. With use of B-mode ultrasonography, the embryo proper is typically apparent by 21 days and the embryonic heartbeat by 24 to 25 days. The allantois should occupy approximately half of the vesicle by 28 to 30 days and most of the vesicle (with the embryo having migrated dorsally within the vesicle) by 33 to 36 days; it should migrate to the ventrum of the allantois by 48 to 50 days. The embryo should also increase in size and development during this time. When development is retarded, heart beat is lost, allantoic shrinkage occurs, or separation of membranes from the endometrium occurs (Figure 8-4), impending pregnancy loss should be suspected.
Abnormalities of Embryo Location and Orientation
Ginther (2007) postulates there are three potential contributors to final orientation of the equine vesicle with the embryo ending up located in the ventral (antimesometrial) position within the uterine horn. Those contributors are (1) differences in tensile strength between two-celled and three-celled layers of the vesicle (i.e., two layers without interposed mesoderm on one side, with three layers including mesoderm [from which embryo develops] on the other side); (2) differential dorsal thickening of the endometrium (encroachment) at the site of fixation; and (3) massaging action of uterine contractions. Ginther notes that the vesicle “rolls” during migration through the uterine lumen before fixation so that orientation of the vesicle before fixation is variable. Immediately after fixation, the previous factors are involved in orienting the embryonic vesicle so that the embryo finally appears in the 6 o’clock position (antimesometrial) within the uterine horn. As pregnancy progresses, the embryo moves dorsally to the 12 o’clock position (mesometrial) within the vesicle during allantoic development and then migrates ventrally as the umbilicus lengthens (back to the antimesometrial position).
Although many singleton pregnancies (beyond 21 days of gestation) have been noted to be in an altered orientation within the uterine horn, variations from the usual have not been proved to be prone to embryonic death. When unilateral twins are present, and one conceptus is spontaneously reduced, it is not uncommon for the eventual orientation of the embryo of the surviving conceptus to be altered. Altered orientation of the embryo also commonly results from development of a conceptus adjacent to an endometrial cyst (Figure 8-5). In these two conditions, the normal mechanism of dorsal endometrial thickening (encroachment) and the massaging action of uterine contractions may be interfered with in some manner by the adjacent twin or cyst.
The embryonic vesicle almost invariably becomes fixed and develops at the flexure (base) of one of the uterine horns. Rarely, the embryonic vesicle develops within the body of the uterus (body pregnancy) instead of at the base of one of the uterine horns. Body pregnancies are thought to be prone to failure even though early development appears normal.
Development Adjacent to Endometrial Cysts
The influence of endometrial cysts on fertility is discussed in Chapter 5. Although whether endometrial cysts affect fertility independent of mare age (from age-related changes in the endometrium that are often present in addition to cysts) remains controversial, when the embryonic vesicle develops adjacent to a cyst, an increased likelihood of pregnancy failure may be found. Certainly many such pregnancies continue to develop normally, with viable foals being delivered at term, so the size of the cyst and early orientation of the embryo in relation to the cyst may be important (Figure 8-6). Newcombe (2000) hypothesized that when the embryo begins development adjacent to the uterine wall, it is likely to develop normally, whereas if it begins development directly adjacent to a cyst, nutrient deprivation is more likely to occur and can result in pregnancy loss, similar to the deprivation hypothesis for spontaneous twin reduction proposed by Ginther (1992).
DEVELOPMENT OF UTERINE EDEMA DURING EARLY PREGNANCY
On occasion, a mare in early pregnancy (14 to 17 days after ovulation) has development of pronounced uterine edema, sometimes associated with behavioral estrus. Assay of progesterone concentration sometimes reveals a low concentration consistent with estrus. Some such pregnancies can be salvaged with immediate administration of progesterone or progestogen, which should be continued until either another ovulation occurs and forms a functional corpus luteum or progesterone supplementation is no longer deemed necessary to support the pregnancy (usually by 100 to 150 days of gestation). Newcombe (2000) suggests that conceptuses in such mares are more likely to be abnormal, particularly when embryonic vesicles are smaller than expected at 12 to 14 days after ovulation; thus, they are more likely to fail even though progesterone supplementation is implemented. An additional cause to be considered is developing endometritis (Figure 8-7), which can precipitate endometrial prostaglandin-F 2α (PGF 2α) release. Close monitoring of embryonic development is warranted in such progesterone-supplemented mares.
MANAGEMENT OF MARES WITH SIGNS OF ABNORMAL EMBRYONIC DEVELOPMENT
With the exception of progesterone and progestogen supplementation of mares developing uterine edema during early pregnancy, the most prudent management of mares with signs of abnormal embryonic development is serial reexaminations at intervals of 1 to 3 days. Colorado workers suggest that if an embryo and heartbeat cannot be identified with ultrasound by day 25 (Figure 8-8), monitoring through day 30 is indicated. If a viable embryo with an evident heartbeat does not become apparent by this time, PGF 2α should be administered to induce luteal regression and return to estrus for rebreeding. Newcombe (2000) also suggests that manual crushing of the conceptus at the time of induced luteolysis, immediately followed by uterine lavage to remove any remaining embryonic debris, might improve chances of conception when such mares are immediately rebred. We have noted that cloudy fluid accumulates in the uterus of some mares after the conceptus is manually crushed, so performing uterine lavage after the crush procedure is recommended. Treatment for more than 1 day may be necessary.
PREGNANCY LOSS BEYOND THE EMBRYONIC PERIOD
Early fetal death (i.e., abortion early in gestation), or pregnancy loss beyond 40 days gestation, usually occurs with no premonitory signs. The expelled fetus and membranes may be found in stall bedding or on pasture but frequently go unnoticed. Ultrasound examination during the period of early fetal death (before expulsion) may reveal intact membranes and fetal fluids of normal echogenicity but a dead fetus (no heartbeat, inactive) (Figure 8-9). In other cases, fetal fluids become more hyperechoic (flocculent or “fuzzy”) in appearance, suggesting the presence of debris or purulent material (Figure 8-10).
![]() |
Figure 8-10 (Courtesy Dr. James Morehead, Equine Medical Associates, PSC, Lexington, Ky.) |
Most commonly, early fetal death is idiopathic and of sporadic incidence. Although no specific pathogens that restrict their attack to the early fetus have been identified, the agents involved in mare reproductive loss syndrome (MRLS) were first thought to have a predilection for pregnancy during the early fetal period. Texas and Kentucky workers showed that the increased fetal losses occurred without a concurrent increase in embryonic losses, despite that many mares carrying embryos (<40 days of gestation) were exposed on the same farms during the outbreak. University of Kentucky workers identified Eastern tent caterpillar involvement in MRLS, which presented as a multifaceted clinical syndrome with pericarditis and unilateral uveitis in adult horses, birth of weak foals, and early (40 to 150 days gestation) fetal loss. However, most susceptible pregnancies were in this early stage of gestation when the outbreak occurred. Later, less severe outbreaks with signs consistent with MRLS (abortion with amnionitis or funiculitis) involved fetuses of later gestational age. Outbreaks of abortion (early or late fetal loss) of similar presentation have been described elsewhere in the United States and in other countries.
When embryonic death (<35 to 40 days) occurs during the breeding season, there is a good chance the affected mare will return to a fertile estrus and thus become pregnant again before the breeding season ends. Regardless of the cause of early fetal death, if endometrial cups are present, the affected mare can rarely be successfully rebred with establishment of another pregnancy during the same breeding season (Figure 8-11). For this reason, early fetal death usually results in failure to produce a foal for another year.
Fetal susceptibility to teratogens decreases with increasing age, except for those structures that differentiate later (e.g., cerebellum, palate, and urogenital system). Regarding infection, the extent of fetal damage that occurs likewise depends on fetal age at the time of infection and degree of fetal immunocompetence, virulence of the infectious agent, and extent of placental lesions and degree of placental dysfunction.
Although the exact level of function necessary to support fetal life and development is difficult to define, a critical level of placental function is deemed necessary to support the developing embryo and fetus to term. Therefore, placental dysfunction is a common cause of pregnancy loss. Possible causes of placental dysfunction include acute or chronic placentitis, hypoxia resulting from alterations in perfusion ratios between uterine and placental blood flow (as occurs with uterine torsion), a defective placenta (e.g., as occurs in hydrallantois), inadequate placental attachment, edema of the placenta, local immunologic reactions in the placenta, and maternal disease or malnutrition. Placental dysfunction may result in a malformed fetus, fetal death, mummification, abortion, fetal growth retardation (Figure 8-12), prematurity, full-term stillbirth, and neonatal weakness and death.
Low birth weights in neonates are often attributed to placental dysfunction caused by infection with microorganisms. Signs in the neonate (such as neonatal weakness and septicemia) that may occur as a result of fetal infection in utero are usually encountered in the first week of life, particularly within the first 24 hours.
Abortion is the termination of pregnancy before the conceptus is capable of extrauterine life. Causes of abortion can be infectious or noninfectious. The overall rate of abortion reported in the horse population varies from 5% to 15%, but “abortion storms” (outbreaks) have been reported in populations of susceptible pregnant broodmares exposed to reproductive pathogens (e.g., Equine herpesvirus [EHV-1], Equine viral arteritis [EVA], MRLS) (Figure 8-13). Observed abortions after 4 months of gestation usually account for a small fraction of equine pregnancy wastage; however, abortions before 4 months of gestation are rarely observed because fetal and placental tissues are relatively small and often overlooked in bedding or on pasture and genital discharges are usually scant after abortion at this early stage. Therefore, most aborted fetuses examined are 6 to 11 months of gestational age. Most equine abortions are the result of placental dysfunction.
< div class='tao-gold-member'>
Only gold members can continue reading. Log In or Register a > to continue
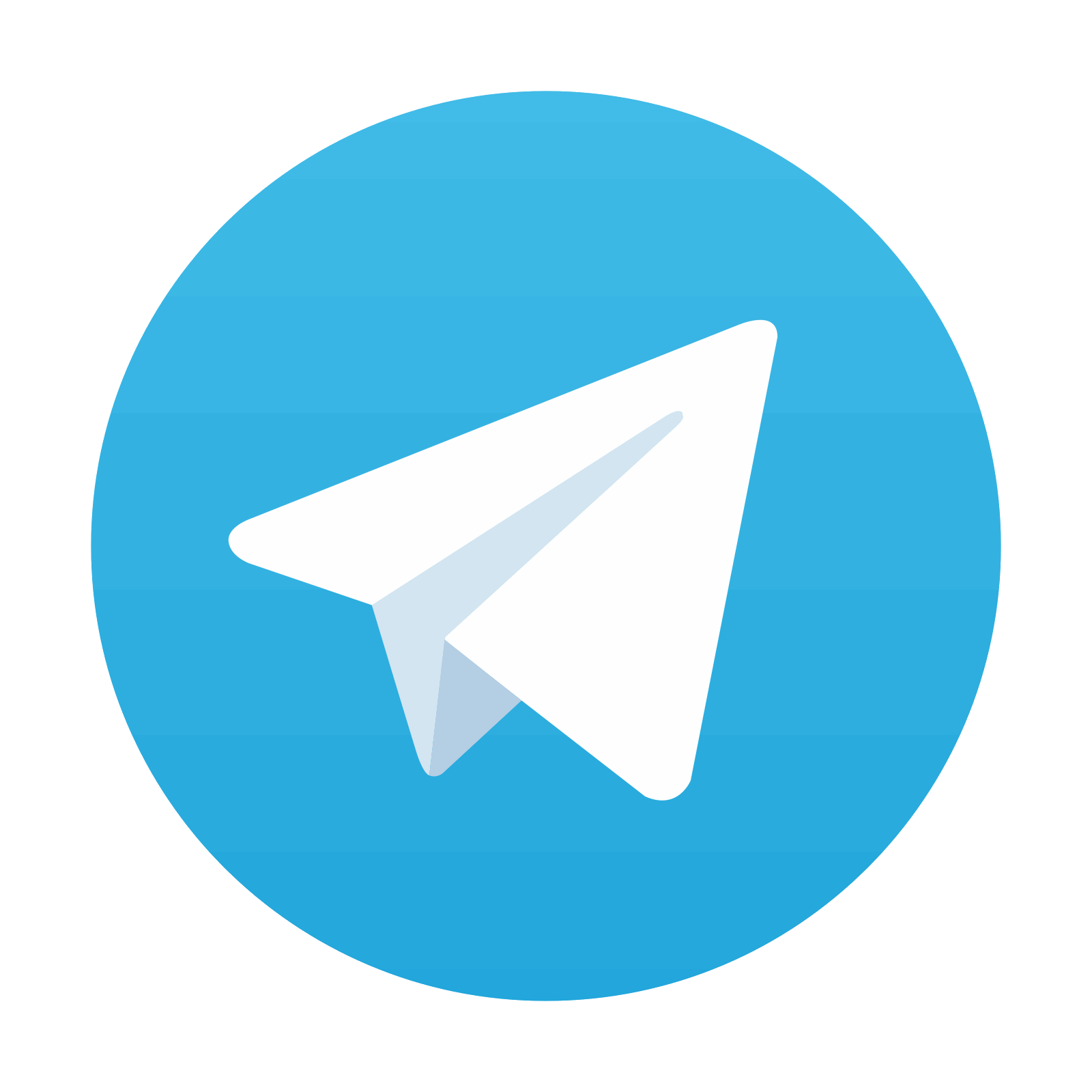
Stay updated, free articles. Join our Telegram channel

Full access? Get Clinical Tree
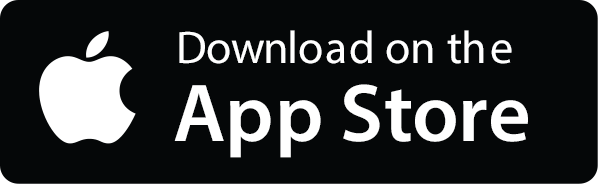
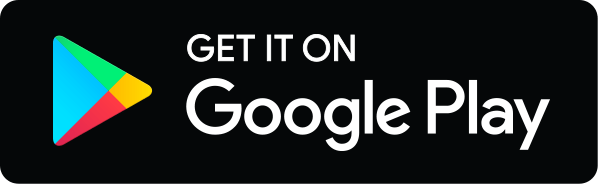
