Lindsay R. Freud1 and Lisa K. Hornberger2 1 University of Toronto; The Hospital for Sick Children, Toronto, ON, Canada 2 Stollery Children’s Hospital; Mazankowski Alberta Heart Institute; University of Alberta, Edmonton, AB, Canada Fetal cardiology has evolved over the past three decades into a highly specialized clinical field that merges fetal–perinatal medicine and pediatric cardiology. At the center of this field is the fetal echocardiogram, which permits the evaluation of fetal cardiovascular anatomy, function, and rhythm through a combination of 2D, M‐mode, and Doppler modalities. Improvements in ultrasound technology have led to an ability not only to detect most forms of congenital heart disease (CHD) in utero but also to evaluate cardiac pathology and physiology in detail. Moreover, it is now possible to diagnose some structural heart lesions as early as the first trimester. Fetal echocardiography has contributed significantly to our understanding of the pathophysiology of structural and functional cardiovascular lesions in utero and of the impact of noncardiac conditions on the fetal circulation. Serial observations have provided insight into the evolution and etiologies of fetal cardiac disease, improved prenatal counseling regarding prognosis, and optimized delivery planning for families and providers alike. In addition, the development of fetal interventions to alter the course of specific diseases has rested on fetal echocardiography. For example, successful pharmacologic strategies to prevent or reverse arrhythmia‐associated fetal heart failure have been developed and are widely used in clinical practice. Minimally invasive fetal interventions for specific forms of CHD and twin–twin transfusion syndrome are increasingly offered, and the fetal echocardiogram remains one of the main tools for assessing candidacy and procedural success. Fetal echocardiography will undoubtedly continue to play a critical role in the evolving management of a large spectrum of primary cardiac and noncardiac fetal pathologies. With the increasing availability of fetal echocardiography and the evolution of fetal therapy, the indications for a fetal echocardiogram have grown (Table 44.1). These indications can be divided into maternal, fetal, and familial categories. Knowledge of the types and incidence of lesions associated with a given risk factor, and the likelihood of diagnosis at a given gestational age are important in the approach to the exam, counseling, and management of the pregnancy. In particular, understanding of associated chromosomal anomalies and extracardiac structural defects is essential as they significantly affect the overall outcome for the fetus [1–6]. The most common reasons for referral for fetal echocardiography include in vitro fertilization, maternal disease, maternal teratogen exposure, and family history of CHD or syndromes associated with CHD [7–10]. The indication for referral most associated with fetal heart pathology, however, is an obstetric ultrasound suggesting an abnormality of the fetal heart [11]. As such, routine obstetric ultrasound screening in all pregnancies plays a critical role in the detection of fetal CHD. In 2013, the obstetric guidelines were expanded to include not only a four‐chamber view of the fetal heart but also visualization of the outflow tracts [12]. This has already led to enhanced prenatal detection of CHD, particularly of outflow and arch pathology, as well as improved delivery planning and management [13]. The timing of the fetal echocardiogram, in part, depends upon the reason for referral. Most fetal echocardiograms are performed in the mid second trimester between 18 and 22 weeks. This is typically the gestational age at which routine obstetric screening ultrasounds are performed and is also prior to the gestational age limit for pregnancy termination (24 weeks) in most regions. Occasionally, referral for fetal echocardiography occurs in the late second or third trimesters when a repeat ultrasound suggests a change in fetal cardiac function, rhythm, or the evolution of structural heart disease. Meanwhile, screening for fetal heart block associated with maternal Sjögren syndrome or systemic lupus erythematosus (SSA‐anti Ro/SSB‐ant‐La antibodies) should begin as early as 16 weeks, which is approximately the time of transplacental passage of disease‐related IgG antibodies. With the advent of endovaginal transducers and high‐resolution curvilinear probes over the past decade, there has been greater interest in first‐trimester fetal echocardiography. This may begin as early as 10–11 weeks [14], prompted in part because of the widespread use of nuchal translucency screening. At 10–14 weeks, increased nuchal translucency identifies the fetus at risk of aneuploidy [15,16] and CHD in the absence of aneuploidy [17–19]. Additional indications for first‐trimester fetal echocardiography include a suspected or known genetic syndrome (beyond aneuploidy), an extracardiac defect associated with CHD detected on first‐trimester obstetric ultrasound, hydrops, and a previously affected child with CHD. The latter indication may provide reassurance to families earlier in the pregnancy. Table 44.1 Indications for fetal echocardiography CHD, congenital heart disease; SSRI, selective serotonin reuptake inhibitor. Diagnostic imaging of the fetal heart requires a technical approach that combines obstetric ultrasound with postnatal echocardiography. The dynamic nature and complexity of the heart requires high frame rates to optimize temporal resolution. To obtain details of the fetal cardiovascular structures, a high‐frequency transducer in the 6–10 MHz range with dynamic focusing is preferred. The highest‐frequency transducer that provides the best penetration is chosen. Frame averaging options or persistence should be off. A compress setting allowing for a narrow dynamic range (grayscale) provides better sensitivity and definition of the blood–tissue interface. While the fetal lungs do not interfere, the maternal abdominal wall may be thick and/or echogenic from adipose or scar tissue. Amniotic fluid provides a very useful interface between the maternal abdomen and the fetal chest; however, fetuses with oligohydramnios may be difficult to image. As the fetal bones calcify throughout gestation, this contributes to limited planes of imaging in the third trimester. Changing to a lower‐frequency transducer for greater penetration (3–5 MHz range) and the use of harmonic imaging can facilitate evaluation in such cases. Color flow Doppler, which provides both the direction of blood flow and averaged flow velocities, is essential to the performance of a fetal echocardiogram. Color settings should be optimized so that the color fills the desired vessel or chamber without causing significant “bleeding.” The Nyquist limit should be set just above the level at which aliasing is seen. The Nyquist limit, color gain, and color persistence should be modified regularly throughout the examination, as during postnatal echocardiography. For example, lowering the Nyquist limit and increasing the gain may be necessary to demonstrate filling of the systemic and pulmonary veins. Power Doppler may also be used to image small vessels or vessels with low‐velocity flow. Power Doppler with high definition (HD) flow, which is now available on most machines, allows bidirectional power Doppler imaging. Spectral Doppler is also routinely used throughout the fetal echocardiogram and requires small sample volumes. In most cases, pulsed‐wave Doppler (PWD) is sufficient for interrogation given the lower velocities present in the fetal circulation. However, if aliasing is present due to higher velocities (i.e., semilunar valve stenosis or ductal restriction), then continuous‐wave Doppler (CWD) should be performed. CWD may not be available on the obstetric curvilinear probes and therefore may require transitioning to a cardiac phased array probe. A complete fetal echocardiogram should include 2D imaging of the fetal cardiac anatomy; color and spectral Doppler interrogation of the intracardiac structures, great arteries and arches, and systemic and pulmonary veins; and assessment of fetal heart rhythm and function (Table 44.2). Table 44.2 Components of a complete fetal echocardiogram IVC, inferior vena cava; LV, left ventricle; RV, right ventricle; SVC, superior vena cava. Assessment of extracardiac Doppler patterns, such as those in the ductus venosus, umbilical artery and vein, and middle cerebral artery, can provide useful information regarding overall fetal well‐being, the effect of cardiac disease on other organs, and placental function. Finally, fetal biometry, including measurements of the biparietal diameter, head circumference, abdominal circumference, and femur length, can be used to calculate the estimated fetal weight and assist if certain features are not consistent with the gestational age. A fetal echocardiogram differs from a postnatal echocardiogram in several important respects. First, one must begin with determination of the fetal position within the mother. This is critical for deciphering right and left positions and the relationship of cardiovascular structures within the fetal abdomen and chest, i.e., visceral and atrial situs. If the fetus is active, the position may shift during the exam and require reorientation to fetal position. Classical imaging planes used through the heart after birth are attempted in the fetus, but are frequently not available; as such, one must be both patient and creative in detailing complex fetal heart disease. The fetal circulation is also different from the postnatal circulation. In the fetus, the foramen ovale and ductus arteriosus provide for redistribution of flow and the equalization of pressure. Although the right ventricle (RV) is the dominant pumping chamber, the downstream resistance of both ventricles is the placenta. As such, indirect observations such as ventricular or great artery size discrepancy may be necessary to determine the severity of a condition. Finally, the anatomy and physiology must be immediately processed and synthesized in order to provide accurate counseling to a usually stressed patient and her partner. The performance of a fetal echocardiogram requires a comprehensive, systematic approach, including the determination of visceral and atrial situs, segmental anatomy, atrioventricular (AV) and ventriculoarterial (VA) concordance, and aortic and ductal arch morphology and position. Cross‐sectional and sagittal images through the fetal chest and short‐ and long‐axis imaging of the fetal heart are valuable in the evaluation of both normal and abnormal fetal cardiac anatomy. The vascular structures are positioned within the fluid‐filled lungs, providing a unique opportunity to appreciate the relationship of these structures to each other and their position within the thorax itself, akin to magnetic resonance imaging after birth. Delineation of visceral and atrial situs requires an orientation to fetal position. This is one of the most challenging tasks for the fetal echocardiographer as there are innumerable fetal positions that may change throughout the course of the study. Fetal position should be determined by scanning from inferior to superior along the maternal abdomen. Examples of fetal position include vertex or cephalic, breech, and transverse. The fetal spine helps distinguish the anterior and posterior aspects of the body. Once the fetal position has been established, use of a doll or model can facilitate recognition of the left and right sides of the fetal body. In another technique described by Cordes et al. [20], the groove of the transducer is oriented to align with the long axis of the fetus with the head of the fetus on the right side of the screen. The transducer is subsequently rotated 90° clockwise to produce a cross‐sectional or transverse image of the fetal chest. In this manner, the scanner has oriented the fetus as breech, i.e., head into the screen. After determining anterior and posterior from the position of the spine, the left and right sides of the fetal body may be determined (Video 44.1). Whatever the technique, labeling may be appropriate. It is also important to note that any of the techniques may be prone to error and often necessitate a hands‐on approach by the reading physician, particularly in the context of more complex disease. Once the left and right sides of the fetus have been determined, cross‐sectional sweeps from the abdomen to the chest provide information regarding visceral and atrial situs. A right‐dominant liver, left stomach and spleen, and right inferior vena cava (IVC) suggest visceral situs solitus (Figure 44.1). Sweeping to the fetal chest, the superior vena cava (SVC) moves anteriorly to connect to the floor of the right atrium (RA), just beneath the septum primum. A cross‐sectional image through the fetal chest provides images of the four chambers of the heart (Figure 44.2, Video 44.2). The heart should be roughly one‐third the size of the fetal chest. The cardiac axis, which is the axis of the ventricular septum relative to the midline of the fetal chest, is 43 ± 7° throughout the second and third trimesters in normal fetuses [21]. Interestingly, the heart is more mesocardic in the first trimester, rotating to the left by the twelfth week [22]. Figure 44.1 Cross‐sectional image through the normal fetal abdomen. Ao, descending aorta; IVC, inferior vena cava; sp, spleen; st, stomach. In the normal fetus, the RA and a portion of the RV are to the right of the midline. The left atrium (LA), left ventricle (LV), and a portion of the RV are to the left of the midline. Any change in cardiac axis or position of the four chambers relative to the midline should prompt a search for either intracardiac or intrathoracic (extracardiac) pathology, such as unilateral lung hypoplasia, congenital pulmonary airway malformation, pulmonary sequestration, or diaphragmatic hernia [23–25]. The LA is the most posterior four‐chamber structure, positioned just anterior to the descending aorta in the normal heart. At least one left and one right pulmonary vein on either side of the descending aorta should be demonstrated connecting to the LA at this level. Color Doppler settings need to be optimized, with an appropriately reduced Nyquist limit and increased color gain, to show the low‐velocity venous flow (Figure 44.3). Figure 44.2 Cross‐sectional image through the fetal thorax demonstrating (a) cardiac axis (angle of the septum (dashed line) from the midline (solid line)) and (b) the four‐chamber view. The arrow demonstrates the offset of the atrioventricular valves. RA, right atrium; RV, right ventricle. Figure 44.3 (a) Four‐chamber view demonstrating a left and right pulmonary vein (arrows). (b) Color Doppler confirms their connection with the left atrium. (c) Normal pulmonary vein flow is low velocity with peaks in ventricular systole (s) and early diastole (d) and decreased flow during atrial systole (arrow). Evaluation of the atrial and ventricular septa is best accomplished from planes of imaging perpendicular to the septa, thus preventing dropout of thinner structures such as septum primum and the membranous ventricular septum. Assessment of the atrial septum should include imaging of septum secundum and primum and the foramen ovale. The “flap” of septum primum should be visualized in the LA consistent with right‐to‐left shunting at the foramen ovale in the normal fetus. Color flow Doppler should confirm low‐velocity right‐to‐left shunting at the atrial level (Figure 44.4). Sweeping posteriorly and inferiorly from the four‐chamber view, the coronary sinus is visualized as it courses within the left posterior atrioventricular groove. Dilation of the coronary sinus, which may be misinterpreted as an atrial septal defect at its mouth into the RA, suggests the presence of a persistent left superior vena cava (LSVC). The mitral and tricuspid valves should be evaluated from both four‐chamber and short‐axis views. In the normal heart, there is a subtle offset of the valves with a more apically displaced septal leaflet of the tricuspid relative to the anterior leaflet of the mitral valve (Figure 44.2, Video 44.2). Sagittal views demonstrate the right SVC and IVC as they enter the RA (“bicaval view”), provide another view of the foramen ovale, and allow for visualization of the atrioventricular (AV) valve morphology en face (Figure 44.5). The anterior leaflet of the mitral valve may be inspected for a cleft and the papillary muscle architecture may be evaluated from this view. The assessment of ventricular morphology may be more challenging in the fetus than the neonate owing to less prominent trabecular patterns. The RV has a more pyramidal shape with a trabeculated sinus and outflow or infundibulum from which one or both great arteries arise. Identification of the moderator band, best recognized in a four‐chamber image, is useful. In contrast, the LV is more bullet‐shaped and has a smooth septal surface. The AV valves, when of normal morphology, can further assist in defining the ventricles. The tricuspid valve is slightly apically displaced and consists of attachments to the ventricular septum. There are three leaflets with a variable number of papillary muscles. On the other hand, the mitral valve is comprised of two leaflets, both removed from the ventricular septum, and two papillary muscles (Figure 44.5d). In normal fetal anatomy, there is continuity between the anterior mitral valve leaflet and aortic valve (Figure 44.6a). The ventricular septum, including the inlet, membranous, muscular, and outlet (conal septum) portions, is evaluated in multiple planes. At a minimum, 2D and color sweeps in the four‐chamber view (inferior to superior) and in the short‐axis view (right to left) should be performed. Additional oblique sweeps, akin to a postnatal parasternal long‐axis view, may be performed to assess the septum further. Slow sweeps with color flow imaging at lower‐velocity settings may permit detection of smaller ventricular septal defects. Sweeping to the fetal head from a four‐chamber view, the ventricular outflow tracts and great arteries can be demonstrated (Figure 44.6, Video 44.3). The LV outflow tract (LVOT) arises more posterior and leftward relative to the right ventricular outflow tract (RVOT), and courses towards the right. The RVOT begins more anterior and courses leftward, wrapping around the LVOT to ultimately meet the pulmonary valve and main pulmonary artery. The main pulmonary artery is positioned to the left of the midline in the anterior–superior aspect of the fetal chest. The three‐vessel view (3VV) facilitates the recognition of normal and abnormal great artery and arch anatomy relative to the trachea [26]. It is a cross‐sectional image obtained through the superior mediastinum. In normal fetuses, the 3VV demonstrates the relationship of the right SVC, ascending aorta, and main pulmonary artery to each other and to other structures within the fetal chest (Figure 44.7a, Video 44.4). The main pulmonary artery is the largest, most anterior and leftward vessel; the ascending aorta is the next biggest, rightward and slightly posterior to the main pulmonary artery; and the right SVC is the smallest, most rightward and posterior vessel. The right pulmonary artery may be seen coursing posterior to the ascending aorta and SVC, whereas visualization of the left pulmonary artery often requires a slight rotation of the transducer from a straight cross‐sectional plane as it courses slightly more inferiorly relative to the right pulmonary artery. The presence of an additional structure to the left of the main pulmonary artery and anterior to the left pulmonary artery often indicates the presence of a persistent LSVC. Figure 44.4 Atrial septal anatomy from the (a) four‐chamber and (b) sagittal views. (c) Color Doppler demonstrates the path of the foramen ovale from right to left atrium and (d) pulsed Doppler demonstrates the pulsatile flow. IVC, inferior vena cava; RA, right atrium; RV, right ventricle. The main pulmonary artery can be visualized joining the ductus arteriosus in the 3VV (Figure 44.7a, Video 44.4). The ductus arteriosus dives straight back just to the left of the midline to meet the left‐sided descending aorta in a normal fetus. Sweeping more superiorly towards the fetal head, the aortic arch can be seen coursing from right anterior to left posterior, crossing the midline towards the left of the trachea to ultimately join the descending aorta as well. These findings confirm the presence of left‐sided ductal and aortic ductal arches. If either arch reaches the descending aorta by coursing rightward of the trachea, then a vascular ring should be suspected. Figure 44.5 Sagittal sweeps through the fetal thorax from right to left in the fetus. (a) Beginning towards the right side of the fetus is the bicaval view. (b) Sweeping leftward, both atria are visualized (solid arrow denotes the right coronary artery), followed by (c) the mitral and tricuspid valves en face. (d) The most leftward image demonstrates the two papillary muscles of the mitral valve in the left ventricle (arrows). AMVL, anterior mitral valve leaflet; Ao, aortic valve; IVC, inferior vena cava; LA, left atrium; PMVL, posterior mitral valve leaflet; RA, right atrium; RV, right ventricle; SVC, superior vena cava. The 3VV is important not only for evaluating the relationship of the great arteries and arches but also for assessing their relative sizes. The size relationship can assist in identifying subtle lesions or outflow tract obstruction. For example, a dilated right SVC may suggest an interrupted SVC with azygos continuation or a vein of Galen aneurysm, whereas aortic hypoplasia may indicate coarctation [27]. For the latter, color flow evaluation is particularly helpful. Flow persistence at the isthmus or even reversal in the transverse arch may be a potent marker of arch obstruction [28]. Figure 44.6 Long‐axis images through the fetal heart (a, b) that demonstrate crossing of the ventricular outlets and great arteries, sweeping up to the normal three‐vessel view (c). (d) A slightly rotated three‐vessel view to demonstrate both branch pulmonary arteries. Ao/AAo, ascending aorta; DA, ductus arteriosus; DAo, descending aorta; LA, left atrium; LPA, left pulmonary artery; LV, left ventricle; PA, main pulmonary artery; RPA, right pulmonary artery; RV, right ventricle; SVC, superior vena cava. From the 3VV, one can rotate the transducer 90° to obtain sagittal or long‐axis images of the arches. By convention, the fetal head should be on the right of the screen. The ascending aorta begins centrally and gives rise to the three brachiocephalic arteries before meeting the descending aorta posteriorly. In normally related great arteries, it has a “candy cane” shape (Figure 44.7b). Regardless of the orientation of the great arteries, it is the more superior arch. The ductus arteriosus arises anteriorly (in the context of normally related great arteries) and inferiorly and should not give rise to other vessels along its course. It has a more flat, “hockey‐stick” shape, joining the descending aorta at a nearly 90° angle (Figure 44.7c). Both arches may be visualized in a sweep with slight counterclockwise rotations. Early in gestation, the left and right sides of the heart are usually symmetric or nearly symmetric in the normal fetus, particularly prior to 16 weeks. Later in gestation, however, the RA, RV, main pulmonary artery, and ductus arteriosus are mildly larger than the left‐sided structures [29,30]. Recognition of the normal degree of asymmetry is important in the detection of more subtle obstructive lesions, including coarctation of the aorta [31]. Use of nomograms and z‐scores for fetal cardiac dimensions, including valve size, ventricular dimensions, and great artery size, and the use of gestational age‐based z‐scores can help differentiate normal anatomy from abnormal pathology [32–34]. Figure 44.7 (a) A slightly rotated (clockwise) three‐vessel tracheal view demonstrates the aortic and ductal arches. (b) Drawing a line from the ascending aorta (fetal right and anterior) to the descending aorta (Ao) (fetal left and posterior) or from the pulmonary artery through the ductus arteriosus (DA) to the descending aorta (left and anterior to left posterior), the three‐vessel view can help one define the course of the aortic (c) and ductal (d) arches. By aligning the transducer with each of these lines and rotating 90°, the long axis of each arch can be acquired. Spectral Doppler interrogation is important for the evaluation of fetal function and rhythm and for the assessment of specific pathologic conditions, such as valve regurgitation or stenosis or ductal restriction. Routine interrogation of the ventricular inflows, outflows, arches, and pulmonary and systemic veins should be performed (Figure 44.8). Moreover, Doppler evaluation of the ductus venosus, umbilical artery and vein, and middle cerebral artery provides important information regarding overall fetal well‐being and peripheral flow (Figure 44.9). Evaluation of fetal heart function requires an understanding of normal fetal cardiovascular physiology as well as developmental changes that occur over the course of gestation. At a minimum, function should be assessed qualitatively on all studies. Certain pathologic lesions require more extensive quantitative assessment, especially if fetal therapy is being considered. Figure 44.8 Pulsed Doppler evaluation of the left ventricular inflow (a), outflow (b), and inferior vena cava (c). Inflow Dopplers are characterized from 12 weeks as biphasic and a‐wave (atrial systole (A)) dominant with increasing e‐wave (early ventricular diastole) velocities through gestation. Outflow velocities progressively increase with gestation with increasing cardiac output. The inferior vena caval flow is characterized by the presence of three phases: forward flow during ventricular systole (s) and early diastole (e), and reversed flow during atrial systole (a). Figure 44.9 Doppler evaluation of the fetal circulation including the ductus venosus (a), umbilical vein (b) and the normal umbilical artery (c). Ductus venosus flow is phasic with forward flow in ventricular systole (s) and early diastole, and forward flow but with reduced velocities (a) in atrial systole. Umbilical vein flow is forward towards the fetus, of low velocity with undulations during fetal breathing. (d) Doppler evaluation of the umbilical artery pulsatility index (mean velocity/peak systolic ‐ minimum diastolic velocity). (e) Umbilical arterial flow of a fetus with severe placental insufficiency with diastolic flow reversal. Cardiomegaly and the presence or absence of hydrops may provide initial clues regarding fetal heart function. The fetal heart size may be measured by the cardiothoracic area ratio, where normal is considered <0.35. Systolic function is often assessed qualitatively, but it may be assessed quantitatively by measurement of the shortening fraction from either M‐mode or 2D images. In the normal fetus, the left and right ventricular shortening fraction is 34 ± 3% [35]. Normal is considered >28%. Although the use of ejection fraction has been documented using Simpson’s technique [36], the reproducibility is poor [37] and, therefore, this approach is not recommended. 3D and 4D echocardiography may ultimately provide the most accurate means of volumetric and mass assessment of the fetal heart (see section “Evolving and future directions”) [38–42]. Stroke volume and cardiac output can be readily assessed by fetal echocardiogram. However, the accuracy and reproducibility of these measurements have not been systematically evaluated. In the normal fetus, the RV contributes 60–65% of the cardiac output, while the LV contributes 35–40% [43–45]. Determination of cardiac output requires accurate measurements of semilunar valve diameters and Doppler interrogation of the outflow tracts with an angle of insonation of <20° to reduce errors. Using the following equation, the stroke volume is calculated: π(diameter/2)2 × velocity time integral). Multiplying by heart rate yields the cardiac output. Given the unique nature of the fetal circulation, the combined cardiac output is often more useful. Normal combined output is 450 ± 50 mL/kg/min. Increased cardiac output may lead to high‐output cardiac failure and/or hydrops in various volume‐loading conditions, such as anemia, sacrococcygeal teratoma, agenesis of the ductus venosus, arteriovenous malformations, and acardiac twin gestations [46–49]. Since measurement of fetal combined cardiac output by echocardiography is subject to inherent variability of multiple measurements and a “gold standard” is not readily available, serial evaluation may be more important than individual measurements to help assess the clinical course and guide management and indication for fetal therapy. Fetal diastolic function may be evaluated by utilizing tools similar to those used in postnatal echocardiography. Spectral Doppler assessment of the ventricular inflows, SVC, ductus venosus, and umbilical vein is critical to recognize alterations in diastolic function and should be performed routinely on fetal echocardiogram. Changes in diastolic function more than systolic function place the fetus at risk of heart failure and hydrops from elevated central venous pressure [50,51]. As gestation progresses, there is an increase in the E‐wave velocity of both ventricular inflows, which reflects developmental changes in the fetal myocardium that permits more efficient relaxation and greater compliance [52,53]. Simultaneous assessment of LV inflow and outflow by spectral Doppler permits measurement of the LV isovolumic relaxation time, which is 43 ± 8 ms (heart rate‐corrected) in the normal fetus from 20 weeks’ gestation to term [54]. Using tissue Doppler imaging (TDI), the same method may be applied to measure the RV isovolumic relaxation time. The Tei or myocardial performance index may be used to assess global fetal myocardial function [55]. The Tei index equals the sum of the isovolumic relaxation and contraction times divided by the ejection time. It can be calculated for either the LV or RV using either PWD or TDI. In the normal second‐ or third‐trimester fetus, the Tei index decreases with gestational age, with the LV index showing a greater decline than the RV index [56]. The Tei index does not, however, discriminate between changes in systolic, diastolic, or combined cardiac dysfunction and may be difficult to measure and reproduce. As such, the Tei index is most often used to evaluate cardiac dysfunction in specific circumstances, such as cardiomyopathy or twin–twin transfusion syndrome [57]. TDI has been described in the assessment of fetal heart function for nearly two decades [58,59]. As is true for the postnatal patient, maximizing the frame rate, minimizing the angle of interrogation, and using a small Doppler gate are essential. In clinical practice, fetal TDI can be obtained in the majority of cases. Normal values for TDI, from 18 weeks’ gestational age to term, have been established for the LV wall, RV wall, and interventricular septum. LV and RV E/e′ normal values have also been published [60]. Generally, pulse‐wave TDI values increase as gestational age increases, with E/e′ values steadily decreasing. Color TDI has also been used to assess tissue strain and strain rate in the fetus [61]. The benefits of color TDI include being able to choose any region of interest offline for assessment [61,62]. However, like pulse‐wave TDI, color TDI remains angle dependent and requires special software that is only available for some systems. More recently, 2D speckle‐tracking echocardiography (STE) has been applied to the fetal heart [63–65]. One of the main benefits of STE is that, unlike TDI, it is angle independent. This can help overcome some of the specific technical challenges that variable fetal position poses. STE allows for assessment of tissue motion at multiple sites and in multiple different planes (longitudinal, radial, and circumferential). Although STE may provide useful insights into fetal myocardial function and adaptation to pathologic conditions, there are technical challenges. High frame rates are essential, measurements may be time‐consuming, and significant differences in vendor platforms limit comparability across cohorts. As a result, STE currently remains largely a research tool. A complete fetal echocardiogram includes assessment of fetal heart rate and rhythm. This is accomplished through the use of either M‐mode or PWD techniques that demonstrate the mechanical events of atrial and ventricular contraction by wall motion or flow, respectively (Figures 44.10 and 44.11) [66,67]. Several PWD techniques have been described to assess flow during atrial and ventricular contraction: simultaneous LV inflow and outflow (Figure 44.11a and b); pulmonary artery and pulmonary vein flow [68]; and SVC and ascending aortic flow [69] (Figure 44.11c and d). The mechanical PR interval may be measured readily from the simultaneous LV inflow and outflow tracing. These techniques are inherently limited by image resolution and fetal position and may require experience in interpreting the tracings. TDI with simultaneous evaluation of atrial and ventricular wall movement at multiple sites may prove to be the most effective echocardiographic technique for assessment of arrhythmias as it is less limited by image resolution and fetal position and may be easier to interpret [70]. Figure 44.10 Rhythm assessment using simultaneous (a) left ventricular inflow and outflow and (b) superior vena cava and ascending aortic pulsed Doppler interrogation. Doppler a–v intervals reflect a rough estimate of the EKG P–R interval. a, atrial systole; Ao, aorta; LV, left ventricle; SVC, superior vena cava; v, ventricular systole. Figure 44.11 Fetal rhythm assessment using M‐mode echocardiography. The M‐mode cursor is directed through the right atrium (RA) and one of the ventricles to obtain the tracing. a, atrial systole; LV, left ventricle; v, ventricular systole. A systematic, comprehensive approach to visceral and atrial situs, segmental anatomy, AV and VA alignments and connections, and aortic and ductal arch positions, morphology, and flow becomes critically important when there is fetal cardiovascular pathology, particularly of a complex nature. When detailing the cardiac pathology, it is important to consider what can and cannot be confirmed prenatally. In addition, it is critical to understand the potential for progression of certain types of fetal cardiac disease throughout gestation. Such information should be conveyed during counseling, and fetal therapy should be considered where appropriate. Finally, as the third trimester approaches, a nuanced understanding of ductal dependency and/or the need for urgent neonatal intervention is essential for proper delivery management at a tertiary care center. The cardiovascular pathology encountered prenatally tends to represent a more severe spectrum of pathology than is encountered after birth. This is due, at least in part, to referral bias: pathology most recognizable in the four‐chamber view (e.g., single‐ventricle heart disease) is more likely to be detected at the time of routine obstetric ultrasound screening [71,72]. In addition, cardiac disease associated with an underlying genetic diagnosis or syndrome, such as aneuploidy, is more likely to be detected [9,71]. A review of the prenatal features of specific forms of CHD is provided in the individual chapters devoted to these lesions. Examples of selected structural CHD in utero are provided in Figures 44.12–44.18 and Videos 44.5–44.11. Fetal echocardiography permits the diagnosis of nearly all forms of CHD before birth [73,74]. The accuracy of fetal diagnosis in the context of more complex CHD has been best demonstrated in heterotaxy syndrome (Figure 44.12, Videos 44.5 and 44.6) where pathology often includes abnormalities of visceral and atrial situs, systemic and pulmonary venous return, AV and VA concordance, outflow tract abnormalities, and arrhythmias. In a large, single‐center series of fetal heterotaxy, Taketazu et al. [75] demonstrated that the majority of the cardiac pathology was accurately defined when compared to observations at autopsy or postnatal echocardiography. There are some important limitations, however, in fetal echocardiography, and the following features may still be missed or under‐appreciated: small to moderate sized atrial and ventricular septal defects, minor valve abnormalities, partially anomalous pulmonary venous return, coronary anomalies, and cardiac lesions that evolve after birth. Coarctation in particular may be difficult to determine until after birth when there is ductal constriction [76]. Although most CHD is present by 5–7 weeks of gestation, there are several ways in which fetal heart disease can evolve in utero [77] as listed in Box 44.1. Some of the progressive forms of disease are uniquely permitted in the fetal circulation where there is redistribution of blood flow. The potential for progression should prompt serial evaluation throughout the pregnancy. Left and right heart obstructive lesions are among those of greatest interest, since these lesions may lead to candidacy for fetal or neonatal intervention. Subtle abnormalities of the aortic or pulmonary valve in the second trimester may be missed, but, owing to progression in the third trimester, may still require neonatal intervention [113]. Mid‐gestation aortic stenosis may initially be associated with subtle ventricular or great artery size discrepancy, with redistribution of flow at the foramen ovale. This lesion often progresses in utero with the development of LV hypoplasia, endocardial fibroelastosis, and retrograde flow in the aortic arch (Figure 44.13, Videos 44.7–44.9). Such progression connotes not only ductal dependency after birth but also inadequacy of the left heart to support the systemic circulation. Such patients are often managed as hypoplastic left heart syndrome (HLHS) with single‐ventricle palliation. A similar process may evolve on the right side of the heart with severe pulmonary stenosis or pulmonary atresia with intact ventricular septum and evolving hypoplastic right heart syndrome (Figure 44.14). This natural history and evolution of heart disease serves as the basis for performing fetal aortic or pulmonary valvuloplasty (see section “Fetal cardiac intervention”). Some cardiovascular lesions are identified more frequently before birth. For example, ventricular aneurysms or diverticula may be more common in prenatal series because this pathology or its associated lesions are not well tolerated [114–116] or are associated with no symptoms after birth (Figure 44.15). Vascular rings (Figure 44.16, Video 44.10) and rhabdomyomas (Figure 44.17, Video 44.11) may also be more frequently diagnosed before birth as they are identified through obstetric ultrasound screening and yet do not necessarily manifest early after birth. Finally, there are unique abnormalities of the fetal circulation. Restriction of the foramen ovale [99] may give rise to the appearance of LV hypoplasia due to decreased left heart preload [117], but this typically resolves after birth. Ductus arteriosus constriction [103,118] may produce RV dilation, hypertrophy, and dysfunction with tricuspid regurgitation. After birth, these neonates may have pulmonary hypertension, in addition to RV dysfunction, which typically resolves over time. Finally, agenesis of the ductus venosus [47] may cause high‐output failure, particularly when associated with direct drainage of the umbilical vein to the RA. Figure 44.12 Fetal echocardiogram in a 27‐week fetus with heterotaxy/asplenia syndrome (segmental anatomy {A,D,D}). (a) Four‐chamber image demonstrating levocardia and an unbalanced atrioventricular canal defect and a dominant D‐looped right ventricle (RV). (b) The pulmonary vein confluence is behind the posterior aspect of the atrium and (c) color Doppler confirms pulmonary venous flow (arrows). (d, e) The pulmonary vein confluence joins a vertical vein (also seen in (a) behind the atrium) that descends through the diaphragm to join the portal venous system (arrow). (f) The “three‐vessel view” demonstrating bilateral superior venae cavae (SVC), a large anterior aorta (Ao) and pulmonary atresia (branch pulmonary arteries indicated by arrow), the latter confirmed by retrograde ductus arteriosus flow. atr, atrium; IVC, inferior vena cava.
CHAPTER 44
Fetal Echocardiography
Introduction
Indications and timing of fetal echocardiography
Maternal
Fetal
Familial
Technical considerations
The screening fetal echocardiogram
Two‐dimensional imaging
Color flow mapping
Pulsed Doppler assessment
Rhythm assessment
Two‐dimensional and color Doppler imaging
Spectral Doppler interrogation
Fetal heart function
Fetal rhythm assessment
Fetal cardiovascular pathology
Fetal structural heart disease
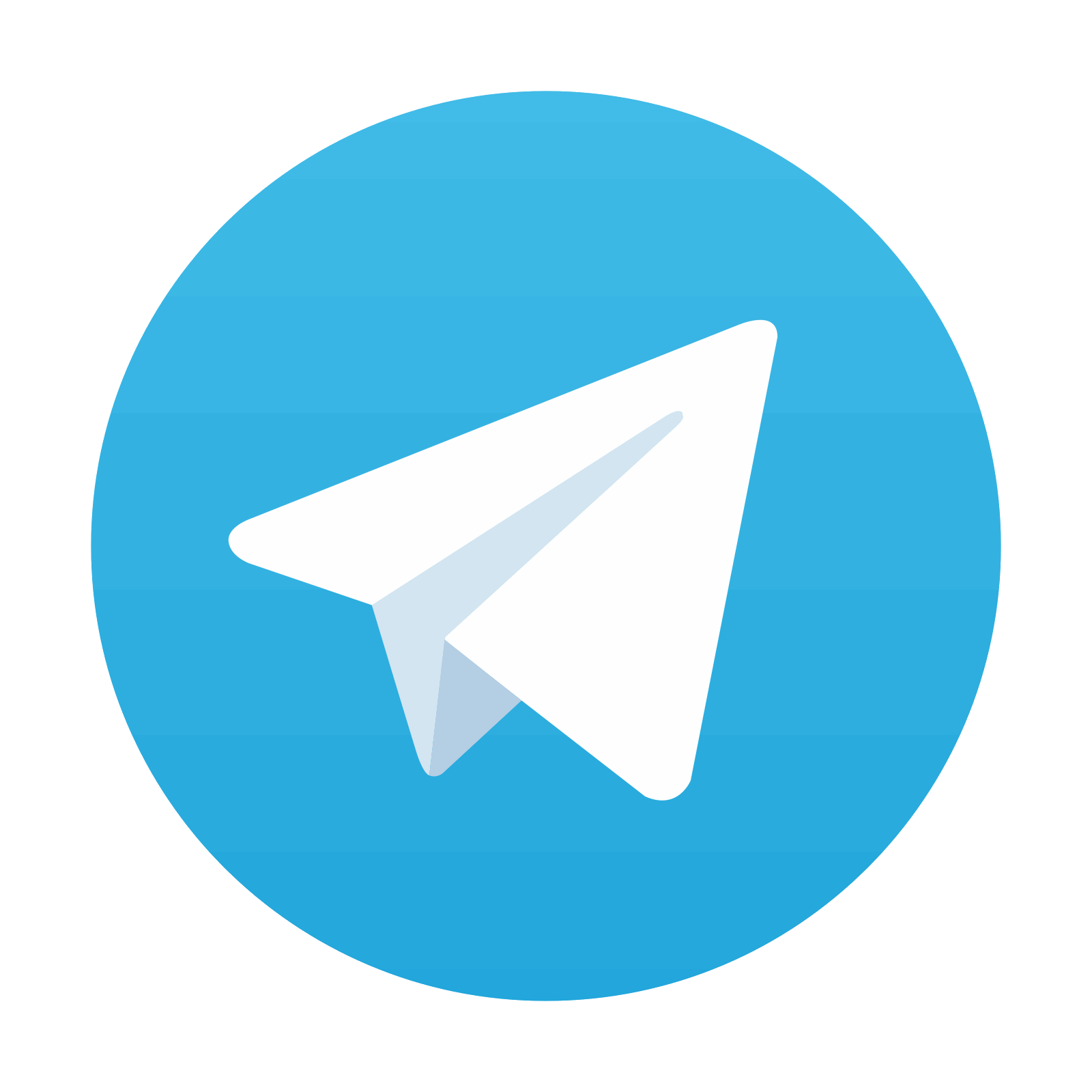
Stay updated, free articles. Join our Telegram channel

Full access? Get Clinical Tree
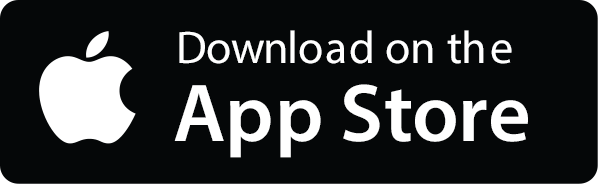
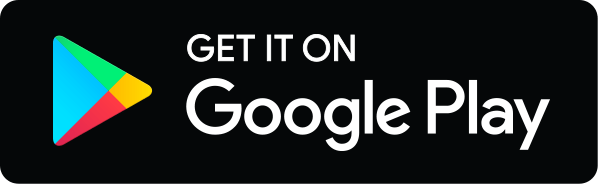