Owen I. Miller and John M. Simpson Evelina London Children’s Hospital, London, UK Transesophageal echocardiography (TEE) is an important imaging modality for the management of patients with congenital heart disease (CHD) [1–15]. Suitable TEE ultrasound probes have been developed that permit high‐resolution imaging of small infants right through to adult patients [16–20]. The major advantages of TEE are that it provides images of both high temporal and spatial resolution and avoids many of the issues of suitable acoustic windows that may limit a transthoracic approach. Furthermore, TEE lends itself to guidance of both surgical [21–29] and catheter procedures [30–36] because it can be performed without compromising access or the field of view for either the surgeon or the interventional cardiologist. The information sought by TEE, any patient‐specific factors, and the type of sedation or anesthesia that may be used during the procedure must all be carefully considered when planning the TEE. Comprehensive guidance for the performance of pediatric TEE has recently been published [37]. In selected intraoperative cases TEE may not be feasible due to patient size, lesion type, or extracardiac contraindications. In these cases, epicardial echocardiography can provide excellent real‐time 2D and 3D imaging of the surgical field [38,39]. In smaller patients, TEE is limited to 2D imaging but in larger children and adults 3D TEE adds further scope to morphologic and functional assessment. Current manufacturer guidance recommends the use of “adult” 3D TEE probes in patients weighing >30 kg but many operators will use such probes down to around 20–25 kg. Smaller 3D TEE probes are currently in development. Epicardial echocardiography is not limited by patient size, and both 2D and 3D modalities are available. In approaching the patient to undergo TEE, the size and age of the patient are fundamental considerations. For infants and children, the procedure is usually performed under general anesthesia [40]. For airway protection, endotracheal intubation is performed, which avoids the risk of TEE probe misplacement. Intubation is preferred over the use of laryngeal masks because of the potential for the probe to dislodge the mask. In selected cases, particularly where spontaneous ventilation is deemed to be a hemodynamic advantage, the patient may be anesthetized/sedated with or without endotracheal intubation and remain spontaneously ventilating throughout the examination [41,42]. The patient’s body size determines the type of TEE probe to be used. In patients >30 kg an “adult” size multiplane 2D or 3D TEE probe can be used for the examination. Multiplane 2D pediatric probes are used below a patient weight of 30 kg, down to a weight of around 3–4 kg. Recently, smaller “micro” TEE probes have been developed that can be inserted in infants below 3 kg [17–19,43], although technical challenges continue to limit the use of these probes. For patients who are “borderline” between the different probe sizes, both sizes should be prepared and be available at the start of the procedure in case the larger probe cannot be safely passed. Whatever probe type is employed, great care should be taken during probe insertion as vigorous or forcible probe insertion is strongly discouraged. Damage to teeth, mouth, hypopharynx, esophagus, and other structures have all been reported [44]. Appropriate positioning of the head and neck by the cardiologist and/or anesthesiologist is important [45] as well as slight anteflexion of the TEE probe to aid initial passage into the hypopharynx and proximal esophagus. During the insertion of the TEE probe, it is important to continue to monitor both the adequacy of ventilation, as the TEE probe can compress the trachea [46,47], and changes in heart rhythm/rate, particularly bradycardia, as the TEE probe may produce an intense vagal stimulus. Probe insertion can also compress posteriorly located cardiovascular structures such as a collecting chamber, descending aorta, or pulmonary vein, which can cause hemodynamic instability. TEE across the age range by definition encompasses children and young adults with varying body sizes. For this reason, the depth of probe insertion should be gauged by direct vision of the echocardiographic image during insertion and should not rely on published standard probe depths at the mouth, or other probe‐oriented marks. Before insertion, the probe must be connected to the echocardiogram machine, the TEE probe should be selected, and the multiplane angle should be set at 0°. Real‐time imaging should be undertaken during insertion to gauge an adequate depth of insertion. Both the cardiologist and the anesthesiologist need to understand the hemodynamics of the underlying cardiac lesion prior to the procedure. Anesthetic agents may cause systemic arterial vasodilation; for example, in patients with tetralogy of Fallot, anesthesia may accentuate right‐to‐left shunting across the ventricular septal defect (VSD) leading to profound cyanosis. Care is also necessary in patients with a total cavopulmonary connection in whom positive pressure ventilation may impede venous return to the heart [48]. Furthermore, in clinical settings where the venous return to the left ventricle (LV) is relatively fixed, such as patients with marked elevation of pulmonary vascular resistance or with severe mitral valve stenosis, hemodynamic instability is more likely. Usually, such patients can be managed safely with appropriate use of intravascular volume expanders, vasopressor agents, oxygen (to reduce pulmonary vascular resistance), or minimizing the period of positive pressure ventilation depending on the individual situation. Consideration of potential adverse physiologic changes prior to the procedure is central to pre‐empting any problems and should be part of any preoperative briefing or checklist routine. Some patients with specific lesions such as repaired esophageal atresia, portal hypertension, or liver disease would appear at greater risk of complications during TEE [49], and some authors have recommended an endoscopic examination prior to consideration of TEE. However, recent reviews have suggested that the risk of bleeding during TEE from known esophageal varices is not significantly increased compared to other subjects [50]. It would seem prudent to exercise extreme caution with such patients but not absolutely contraindicate TEE if this imaging approach is clinically important and other imaging modalities will not provide similar information. There have been major advances in the design of TEE probes over the past decade. Currently, all major manufacturers produce multiplane TEE probes suitable for both adults and children [51]. “Micro” TEE probes have been developed that have the potential to extend TEE to low birth weight infants. Micro‐TEE probes may also be used in older patients via a nasal route whilst awake using local anesthesia. The passage of the smaller instrument, similar in size to a nasogastric tube, may be well tolerated. Despite their potential, widespread use of these microprobes has been limited by their reliability and durability. The controls on current multiplane TEE probes include an ability to electronically vary the sector plane angle continuously from 0° to 180° and two additional physical controls that allow the operator to induce a bend at the probe tip, both in the anteroposterior and lateral planes. Using the convention of the patient being supine and the imaging surface at the tip of the probe facing forwards, inducing an anterior bend is termed anteflexion and inducing a posterior bend is called retroflexion. Finally, a lateral bend may be induced and is described as rightward or leftward in relation to the patient’s right and left, respectively. Lateral flexion controls are usually present on adult‐size probes but may not be present on all pediatric or other miniaturized probes. Currently available 3D TEE probes that are suitable for patients who weigh >30 kg [52,53] have incorporated the real‐time 3D “matrix” arrangement of ultrasound crystals into a very small footprint, appropriate for transesophageal use. 3D images can be obtained as a volume dataset over several cardiac cycles providing a large field of view and increased volume/frame rate or as a “live 3D” for more focused real‐time imaging but usually with a smaller field of view. Applications of 3D TEE, including both surgical and interventional, are discussed later. Whichever probe is selected for a particular patient, careful attention needs to be paid to the safety of the instrument itself. This includes careful inspection for surface cracks in the probe lining, which may represent both an infection risk and an electrical risk. Manufacturers endorse various cleaning and maintenance protocols ranging from antiseptic immersion to surface cleaning. In our institution we use a strictly audited surface disinfection protocol before and after every use of the probe in addition to a latex‐free disposable sheath for each procedure [54,55]. To assist any future audit for potential cross‐infection, a detailed log is kept for each probe with date, time, patient demographics, and batch number for the disinfectant product. Transthoracic echocardiography (TTE) has evolved standard imaging planes based primarily on accessible sonographic windows. These standard imaging planes allow interoperator comparison and form the substrate for echocardiographic reporting of cardiac structure and function. TEE also has well‐described standard views [20]. These predefined views are, however, based on an assumption of levocardia and normal intracardiac connections. For the great majority of TEE done in the adult cardiovascular setting these tenets hold true. Indeed, for many pediatric patients, these “standard” TEE views can be used. The standard views are particularly useful when imaging the valves [56–61] or the interatrial septum [62–64], or when looking for intracardiac thrombi [65–67]. Importantly, TEE should be seen as adding additional information to existing TTE images. Although TEE indications may include poor TTE windows, the decision to proceed to TEE may more commonly relate to better sonographic windows for certain specific cardiac structures such as the atrial septum, the atrioventricular (AV) valves, and the aortic valve. For this reason, TTE and TEE are not mutually exclusive; indeed, it is our view that most TEE examinations should be accompanied by TTE where necessary. TEE should be performed to obtain the missing diagnostic information that could not be obtained by TTE. Expertise in TEE requires a high level of familiarity with both normal and abnormal cardiac structures, and, for cases of congenital heart TEE, the operator must be an expert in the full range of CHD TTE to fully exploit the potential of TEE in this population [68–70]. When TEE studies are performed under general anesthesia (GA), it is our usual practice to use the same GA to acquire 2D and 3D transthoracic images and then proceed with acquiring the relevant TEE images. For 3D TEE, breath‐holding allows the acquisition of higher frame‐rate multibeat full‐volume 3D acquisitions without respiratory artifacts. Standard predefined TEE views can reliably predict normal cardiac structures seen at a particular probe depth or imaging angle. However, in patients with structural CHD, cardiac position and intracardiac connections can be highly variable, thus adaptation from standard views is frequently required to obtain optimal imaging of structures of interest. In the congenitally abnormal heart, there may be both positional abnormalities such as dextrocardia and/or significant variation in connections and alignments, such as in AV and/or ventriculoarterial discordance. Furthermore, target structures such as the semilunar valves may not be seen in the standard planes due to their abnormal position. A comprehensive TEE protocol used outside of CHD may include up to 20 standard cross‐sectional views to evaluate all heart structures in the patient with normal cardiac anatomy. Despite anatomic variations across the spectrum of CHD, these standard views provide a good starting point for a comprehensive TEE examination in CHD. More recent pediatric and congenital recommendations extend this to 28 standard TEE views [37]. In both TTE and TEE, the imaging sector can be displayed both with the sector apex at the top and also inverted with the sector apex at the bottom of the screen. Although many noncongenital echocardiographers will perform all TTE and indeed TEE views without inverting the sector, it has become accepted practice in congenital cardiology to invert the image for certain standard views when performing a comprehensive echocardiogram to allow more anatomically accurate projection of cardiac structures. In TTE this usually relates to subxiphoid and apical four‐chamber views where the cardiac apex is lowermost on the screen. When performing pediatric TEE for CHD it is our practice to invert the image for transgastric views to allow a more intuitive display of the ventricles with the LV on the left and the outlets towards the top of the screen. Some laboratories similarly prefer to display certain esophageal views (e.g., four‐chamber view) with the apex lowermost on the screen, resulting in image orientation analogous to TTE. In many instances, a full TEE study including all the different standard views is not feasible due to unstable patient hemodynamics or concurrent surgical or catheter interventions. Additionally, TEE performed under conscious sedation rather than GA will limit the duration of the study and may reduce the possibility of completing a comprehensive TEE. In these cases, it may be advantageous to concentrate on the missing imaging information and on the structures of interest so that if the study is curtailed or study time is limited, then at least the most important TEE data would have been acquired. Regarding applicability of 3D TEE, including both multibeat full‐volume acquisitions (needing further post‐processing) and “live” real‐time 3D TEE, comment will be made on suitability and approach for 3D TEE over and above a routine 2D TEE approach for selected lesions described in the following sections. The standard TEE views predominantly describe probe depths: upper esophageal (UE), midesophageal (ME), transgastric (TG), and deep transgastric (DTG) along with the sector plane angle. Although the TEE in CHD will need to be adapted to the morphology, the following standard views are described with an initial assumption of a normally connected heart so that the core standard views can be understood and then adapted as required. Despite the possibility of cardiac malposition and abnormal connections in CHD, the ideal starting point for most TEE in CHD is still the midesophageal (ME) view, at 0°; a view analogous to the TTE apical four‐chamber view. Axial rotation of the probe may be required to center the sector immediately posterior to the atria, which is usually the morphologic left atrium. The cardiac mass should be visible, and the sector depth is adjusted to include both the atrial and ventricular chamber(s). The apex of the sector is at the top of the screen, with the heart represented in anatomic format with the atrial mass uppermost and the ventricular mass toward the widest part of the imaging sector. Even in the presence of major structural problems, this starting view will allow orientation of left–right, posterior–anterior, and superior–inferior at the initial phase of a sequential examination (Figure 42.1). This starting view can be readily achieved by inserting the probe, already selected for live scanning, with the imaging surface of the probe tip facing anteriorly, the multiplane angle set at 0°, and the probe depth assessed “live” during gentle introduction. The echocardiographic sector at 0° from this ME depth will cut through the atria, AV junction, and ventricular mass from base to apex. However, as the cardiac apex is usually more inferior to the base, the ventricular chambers may appear foreshortened. Gentle retroflexion of the probe tip allows the true apex of the heart to be visualized and this retroflexion maneuver should be routine for the ME 0° four‐chamber cut. Without retroflexion, there can be a misinterpretation of ventricular hypoplasia (Figure 42.2). Figure 42.1 Transesophageal echocardiogram in a midesophageal position demonstrating a four‐chamber view. LA, left atrium; LV, left ventricle; RA, right atrium; RV, right ventricle. Figure 42.2 Transesophageal echocardiogram in a midesophageal position demonstrating a four‐chamber view, but without retroflexion. In this example the apex of the left ventricle (LV) is somewhat foreshortened. LA, left atrium; RA, right atrium; RV, right ventricle. From this view, the intracardiac AV connections can be defined, the size and function of the AV valves can be described, and spectral Doppler assessment of the AV valve inflow and regurgitation can be achieved at generally acceptable insonation angles. From this initial four‐chamber cut, one system of interrogation is to then perform a sequential segmental study starting at the venous connections. The superior vena cava (SVC) can be seen in short axis by withdrawing the probe towards the upper esophagus at 0° while centering the image on the atrial septum. Advancing the probe into the lower esophagus until the inferior vena cava (IVC) is seen in cross‐section will demonstrate the atrial septum from its inferior to its superior border (Video 42.1). The presence of any atrial septal abnormalities is noted during this sweep and can then be displayed in closer detail at optimal depth and then at multiple sector angles. Assessment of an atrial septal defect is described in the section “Atrial septal defect.” Note should be made of other identifying characteristics of the right atrium, including the broad base of the right atrial appendage, the Eustachian valve, Chiari network, and drainage of the IVC to the atrium. Failure to identify these right atrial structures should raise the possibility of a laterality defect. Having demonstrated systemic venous connections and right atrial morphology, the operator can return to ME 0° to then describe the pulmonary venous connections. Gross axial rotation of the probe, at ME 0–20° multiplane angulation, allows visualization of structures adjacent to the main cardiac mass. Pulmonary veins first from the right and then from the left can be seen at each heart border and may require gentle manipulation of the probe depth to bring in the inferior and superior pulmonary veins sequentially as these may not be visible at the same probe depth within the esophagus (Figure 42.3). Once visualized, the pulmonary veins should be interrogated with color flow mapping (CFM) and pulsed‐wave (PW) Doppler to establish a pulmonary vein flow profile. This is particularly important in pulmonary vein stenosis, mitral valve abnormalities, and other forms of left heart dysfunction. As well as capturing a trace with cyclical ventilation, a second capture of the pulmonary venous Doppler trace should be obtained without artificial ventilation or positive end expiratory pressure (PEEP), as mechanical ventilation will affect pulmonary venous return. It is important to avoid extreme flexion of the TEE probe in smaller patients as this may itself cause compression of the pulmonary veins leading to an erroneous diagnosis of pulmonary veins stenosis. For both right and left pulmonary veins, a long sweep usually at 0–20° will allow the operator to follow the pulmonary veins from their extrapericardial origin to their atrial entry (Video 42.2). Abnormalities of pulmonary venous return are best visualized during this sweep and confirmed following a 3D TTE or 3D TEE acquisition. Failure to identify all pulmonary veins should prompt a detailed evaluation of possible sites for anomalous pulmonary venous drainage, including an evaluation of a greater length of the SVC, both at 0° and at 90°. Finally, in the heart with atrial situs solitus, the left atrial appendage is best seen at the midesophageal depth with 60–90° multiplane angulation. Figure 42.3 Transesophageal echocardiogram demonstrating the entry of the right upper pulmonary vein (RPV) to the left atrium. The right pulmonary veins pass behind the superior vena cava (SVC) which is cut in cross‐section with the probe at 0° of rotation. RA, right atrium. Demonstration of the AV junction can also start with the ME 0° view. Axial rotation of the probe allows specific visualization of the left and right AV junctions. In normally connected hearts, the tricuspid valve can be best seen with a slight clockwise rotation of the probe to improve the insonation angle for any color Doppler or spectral Doppler interrogation. The mitral valve is best seen with slight anticlockwise rotation of the probe and it is from this view that optimal real‐time 3D imaging of the atrial aspect of the mitral valve is obtained. Further detail of 3D optimization will follow. Keeping the multiplane angle at 0–20°, the probe can be gently withdrawn to be slightly higher than the midesophageal position; this minor alteration in probe depth should allow visualization of the left ventricular outflow tract (LVOT) and the great artery connection arising from the center of the cardiac mass. In the normally connected heart, this great artery is the aorta and keeping a low multiplane angle 0–20° establishes a quasi five‐chamber view of the LVOT. Despite this analogy to the TTE view, it is not always possible to obtain a reliable Doppler angle from this position and this view offers much less detail of other CHD lesions such as VSDs, subaortic stenosis, or, in some patients, aortic valve pathology. All of these clinical/morphologic questions are better answered by further 2D TEE views described later or by a full‐volume 3D dataset acquisition. After completing the sequence of 0° views mentioned earlier, the operator can then return to the standard ME depth, select a multiplane angle of between 30–45°, and withdraw the probe slightly, at all times watching the image display to see when the aortic valve comes into view. Rotation of the probe to position the aortic valve in the center of the sector and adjustment of the imaging depth will allow fine detail of the aortic valve to be displayed. In this view, the aortic valve cusp aligned with the interatrial septum is the noncoronary cusp, the cusp to the right and anterior is the right coronary cusp, and the remaining cusp is the left coronary cusp (Figure 42.4). Figure 42.4 Transesophageal echocardiogram short‐axis view of the aortic valve at 40° rotation. The three leaflets of the aortic valve can be clearly identified as well as the right ventricular outflow tract wrapping around the aortic valve. PV, pulmonary valve; RA, right atrium; RV, right ventricle. Advancing the multiplane angle to 60–70° will bring the body of the right ventricle (RV) and the pulmonary valve into view. This view also contains part of the membranous interventricular septum between the tricuspid valve on the left of the display screen and the aortic valve in the center (Figure 42.5). A membranous VSD would be seen as a defect in the otherwise smooth outline of the aortic annulus, and color flow mapping will demonstrate the shunt. Once demonstrated, further definition is possible at 120° (see later). The pulmonary valve is an anterior structure and therefore not ideally demonstrated by TEE. It is, however, possible to visualize the valve annulus and any obvious dysplasia of the leaflets. Color flow mapping may show aliasing in cases of pulmonary valve stenosis, but the Doppler angles are generally not ideal for velocity estimation. Information about the pulmonary valve obtained by TEE should be compiled together with data obtained by TTE, which is usually a better method for displaying this valve. The transgastric outflow tract view (see section “Transgastric views”) can also be used for better Doppler alignment. The right ventricular outflow tract can be included in a 3D TEE volume dataset; it is then our practice to display this post‐processed image in an anatomically correct orientation, which requires the whole dataset to be rotated to show the pulmonary valve in its superior‐anterior position (Figure 42.6). Figure 42.5 Transesophageal echocardiogram of the right ventricular outflow tract which shows all components from the tricuspid valve (TV) through to the pulmonary artery. AoV, aortic valve; LA, left atrium; PV, pulmonary valve; RA, right atrium; RV, right ventricle. Figure 42.6 3D Transesophageal echocardiogram post‐processed full‐volume acquisition at midesophageal depth, 0° rotation, to demonstrate the right ventricular outflow tract as viewed from the right side and displayed anatomic orientation. Ao, aorta; IVS, interventricular septum; RA, right atrium; RVOT, right ventricular outflow tract. Gentle withdrawal of the probe into the upper esophagus (UE) will demonstrate the origin and course of the right pulmonary artery but the left pulmonary artery is less well demonstrated. If more detailed intraoperative imaging of the pulmonary arteries is required, epicardial echocardiography can be very useful, especially after tetralogy of Fallot repair or a cavopulmonary anastomosis [39]. Counterclockwise rotation at a multiplane angle of 60–70° may bring the left atrial appendage into view to the right of the screen (Figure 42.7). As discussed earlier, this assumes normal atrial situs. Figure 42.7 Midesophageal view at 60–70° to view the left atrial appendage (LAA). LA, left atrium; LV, left ventricle. Oriented with the transducer facing anteriorly, the ME 90° view allows composition of a “two‐chamber view” of the left atrium and LV. Anterior structures (left atrial appendage, anterior mitral valve leaflet) are to the right of the screen and posterior structures (inferior papillary muscle, posterior mitral valve leaflet) are to the left of the screen. Detailed descriptions of the standard multiplane and multidepth images of the complete TEE examination showing all three major scallops on both the anterior and posterior mitral valve leaflets in an anatomically normal mitral valve exist in the adult literature and will not be further discussed here. These conventions describing which multiplane angle cuts through which part of the valve annulus can be useful even in congenital heart practice but cannot be relied upon to predefine any specific valve cusp in the congenitally malformed left AV valve. Once again, 3D TTE and TEE has proven more useful in the spatial description of AV valve abnormalities and their anatomic relations [29,69,70]. Importantly, in congenital heart practice, the ME 90° view when rotated axially to image posteriorly, clearly displays the atrial septum in long axis; with gentle withdrawal it can demonstrate the SVC–right atrial junction (Figure 42.8) and when advanced can show the IVC–right atrial junction albeit with less predictable image clarity. The presence and size of any atrial septal defect (ASD) already shown at 0° can be seen orthogonally and dimensions of both the defect and critical caval rims for possible device closure can be measured. In the normally connected heart, this view (110–130°) will demonstrate the LVOT, can confirm mitral–aortic continuity, if present, and will visualize a portion of the interventricular septum in the membranous region (Figure 42.9). The aortic valve cusp seen adjacent to the interventricular septum towards the bottom on the display is usually the right coronary cusp and this 120° angle best demonstrates any coronary cusp prolapse or cusp involvement in any adjacent VSD (Video 42.3). The other aortic valve cusp seen in this midesophageal long‐axis view can be either the left or the noncoronary cusp depending on cardiac position and actual multiplane angle. Identification of the other cusp is best done by 3D or 2D sweep from short to long axis of the valve. Other aortic valve pathology and dysfunction, including aortic valve incompetence, is also seen well at 120° in augmented color Doppler, and in patients of an appropriate size by a 3D TEE color acquisition to allow a multiplanar view of any regurgitant jet or indeed jets. Figure 42.8 Bicaval view of the atria showing the curved atrial septum. The left atrium (LA) is posterior and right atrium (RA) anterior. The Eustachian valve is seen close to the entry of the inferior vena cava (IVC) to the RA. SVC, superior vena cava. Figure 42.9 Midesophageal view at ~120° to demonstrate left ventricular outflow tract, aortic valve, and proximal aortic root in long axis. Ao, aorta; LA, left atrium; LV, left ventricle; MV, mitral valve; RV, right ventricle. Transgastric TEE views are best obtained by returning any flexion to neutral, returning the multiplane angle to 0°, and gently advancing the probe beyond the lower esophagus until the heart is no longer seen. Once in the stomach, the probe is now anteflexed and gently withdrawn until the heart comes into view. It is our practice to invert the imaging sector and allow a more intuitive anatomic projection of the cardiac chambers and outflow tracts. Figure 42.10 Transgastric echocardiogram view of the left ventricular outflow tract (135°). The chordal support of the mitral valve (MV) is well seen in this projection. The left ventricular outflow tract is seen in long axis and the pulmonary valve (PV) in short axis. The projection also facilitates Doppler interrogation of the left ventricular outflow tract. AO, aorta; LA, left atrium; LV, left ventricle.
CHAPTER 42
Transesophageal and Intraoperative Echocardiography
Introduction
Patient considerations
Available TEE probes and technical considerations
Standard transesophageal views
Midesophageal view
Midesophageal view at 0 degrees (ME 0°)
Venous connections and the atrial septum
Atrioventricular junction
Left ventricular outflow tract
Midesophageal view at 30 degrees (ME 30°)
Midesophageal view at 60 degrees (ME 60°)
Midesophageal view at 90 degrees (ME 90°)
Midesophageal view at 120 degrees (ME 120°)
Transgastric views
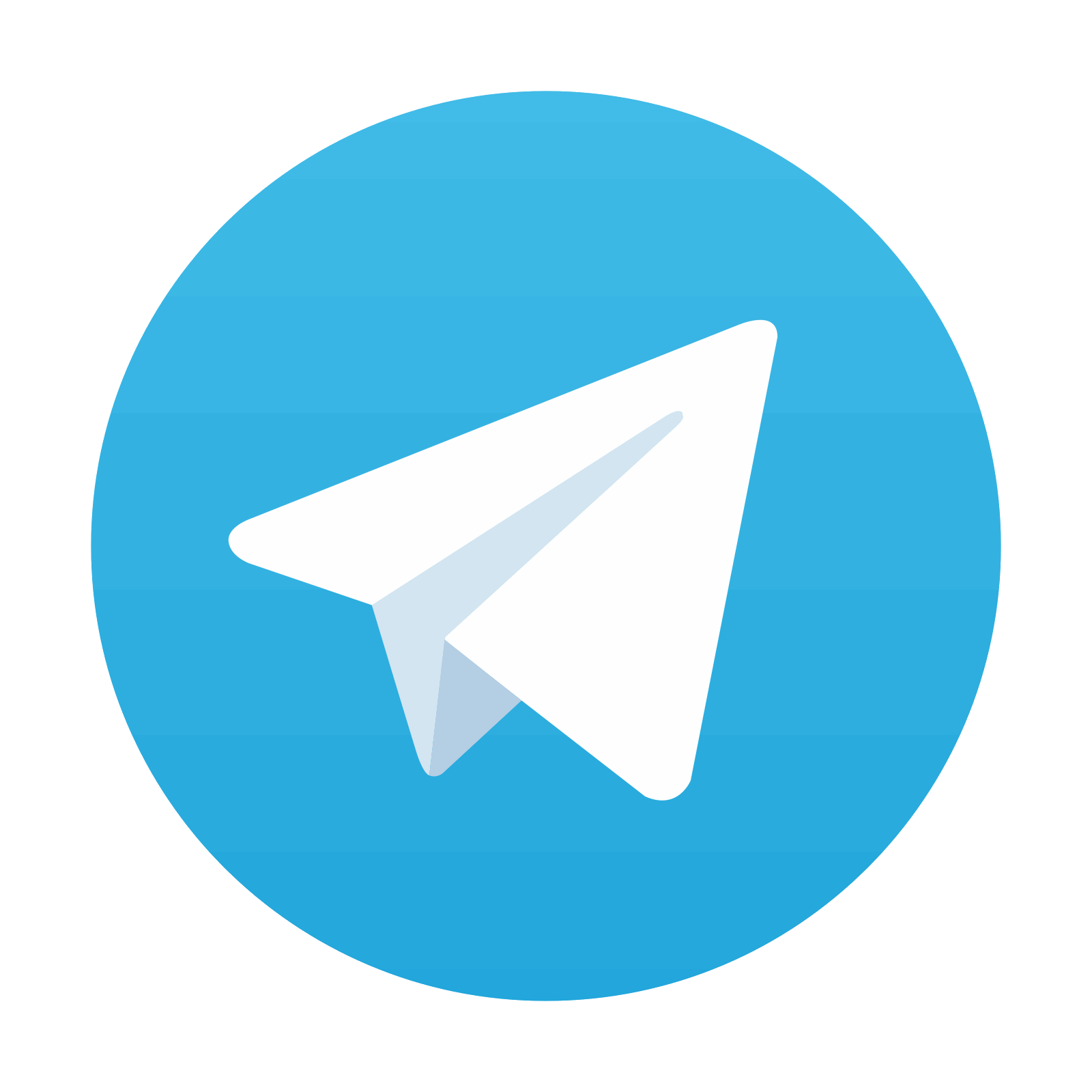
Stay updated, free articles. Join our Telegram channel

Full access? Get Clinical Tree
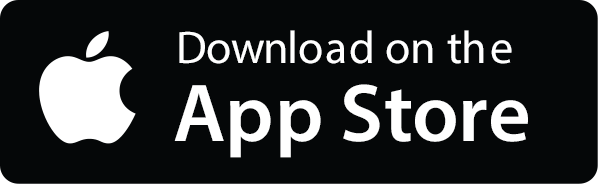
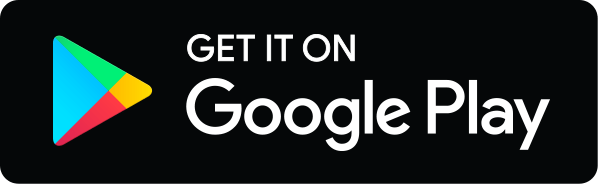