Dale A. Burkett and Adel K. Younoszai Children’s Hospital Colorado, Aurora, CO, USA Restrictive cardiomyopathy (RCM) is a rare form of heart muscle disease, characterized by abnormal ventricular compliance, with normal or decreased volume of both ventricles, biatrial enlargement, normal left ventricular wall thickness and atrioventricular valves, impaired ventricular filling, and normal or near‐normal systolic function and wall thickness [1,2]. The variable natural history depends, at least partially, on the etiology. Outcomes for RCM are worse than for all other forms of cardiomyopathy [3]. The probability of survival at 1, 2, and 5 years is 82%, 80%, and 68%, respectively, although transplant‐free survival is only 48%, 34%, and 22%, respectively [3]. The degree of hemodynamic abnormality and the risk of sudden death are significant, and heart transplantation is the treatment of choice once symptoms are present, given there is no proven medical therapy that will enhance survival [3–7]. Restrictive cardiomyopathy is the least common of all pediatric cardiomyopathies, representing <5% of cases [1]. No known racial or significant gender predilection exists [3]. As summarized in Table 37.1, there are multiple etiologies for RCM, which may result from myocardial diseases (including infiltrative and noninfiltrative processes), storage diseases, endomyocardial diseases, myocarditis, and following particular exposures or transplantation. In adults, endomyocardial fibrosis in the tropics and amyloidosis outside the tropics are the most common causes of RCM [8,9]. Idiopathic RCM represents the majority of cases in children. Familial cases are usually characterized by autosomal dominant inheritance with variable penetrance and expressivity, and include mutations in genes encoding sarcomeric proteins (troponin I, troponin T, alpha‐cardiac actin, beta‐myosin heavy chain, myosin‐binding protein, troponin C, tropomyosin 1, myosin light chain) and, less commonly, nonsarcomeric mutations (desmin, filamin‐C) [1,9]. Restrictive physiology is often seen in patients with hypertrophic cardiomyopathy, which should be excluded before establishing a diagnosis of RCM. The physiology of RCM is characterized by impaired myocardial relaxation and ventricular compliance leading to atrial dilation and dysfunction. As a result, ventricular filling is primarily limited to early diastole. Typical hemodynamic characteristics include increased ventricular end‐diastolic pressures with equalization of diastolic pressure between the atrial and ventricular chambers. Anatomic findings include atrial enlargement, normal ventricular size with normal wall thickness, or concentric hypertrophy. Histology reveals endocardial and interstitial fibrosis with increased collagen deposition, myocyte hypertrophy without myofiber necrosis or disarray, and without inflammatory infiltration. In children, endomyocardial biopsies are usually not helpful in the diagnosis, unless amyloidosis or hemochromatosis is suspected [10,11]. Echocardiography is uniquely suited as a diagnostic tool to detect the anatomic and functional abnormalities associated with RCM and remains the major noninvasive imaging modality employed in the initial assessment of restrictive and constrictive physiology [1,12]. A complete study composed of 2D imaging, M‐mode, color and spectral Doppler interrogation, and speckle‐tracking echocardiography, with attention to both systolic and diastolic function, allows the examiner to identify specific features of RCM, evaluate for concomitant pulmonary hypertension, and to differentiate the process from constrictive pericarditis, which can prove difficult. In RCM, both ventricular cavities are typically small to normal in size. Marked biatrial enlargement is a classic feature of RCM, although it lacks sensitivity (Figure 37.1, Video 37.1). Atrial dimension in adults often measures >45mm in the apical four‐chamber view, and a left atrial dimension >60 mm has been associated with a poor prognosis [13]. Atrial thrombi may be seen, especially in the appendages. Normal wall thickness or concentric left ventricular hypertrophy, with normal or slightly decreased systolic function is typical [14]. A fractional shortening of the z‐score is associated with pediatric RCM outcomes [3]. Thickening of the atrial septum and cardiac valves is common. Valvar dysfunction is often present, especially atrioventricular valve regurgitation (Figure 37.2). In most cases, there is evidence of pulmonary hypertension, as estimated by the tricuspid regurgitation jet or interventricular septal flattening or bowing (Figure 37.3). Right ventricular pressure may be underestimated using the tricuspid regurgitation jet method because the atrial pressure (which must be included in the equation) is often fairly high. Subxiphoid imaging often reveals that the inferior vena cava is dilated and does not collapse with inspiration (plethora), reflecting increased right atrial pressure (Figure 37.4). A pericardial effusion may be present. Myocardial reflectance is usually increased. In the case of amyloidosis, the ventricular walls are thickened with a granular or sparkling myocardium on echocardiography, reflecting amyloid deposits. Table 37.1 Etiologies of restrictive cardiomyopathy Figure 37.1 Four‐chamber view of a patient with restrictive physiology. (a) Both ventricular cavities are small with decreased end‐diastolic dimensions. The marked atrial enlargement (“ice cream cone heart”) is pathognomonic, and atrial enlargement can prevent imaging of the entire heart in a single capture, requiring dedicated left (b) and right (c) heart imaging. LA, left atrium; LV, left ventricle; RA, right atrium; RV, right ventricle. Figure 37.2 Apical four‐chamber view of a child with restrictive cardiomyopathy showing severe tricuspid regurgitation (a), mild mitral regurgitation (b), and pericardial effusion from increased filling pressure. LA, left atrium; LV, left ventricle; PE, pericardial effusion; RA, right atrium; RV, right ventricle. Doppler echocardiography remains the primary clinical tool for providing reliable assessment of diastolic function. Determination of severity of diastolic dysfunction is important not only for diagnostic but also for prognostic reasons, given worsening diastolic function is associated with increased morbidity and mortality [15]. The evaluation of diastolic function is routinely comprised of the measurement of mitral early (E wave) and late (A wave) diastolic inflow, mitral E‐wave deceleration time, pulmonary vein systolic (S wave), diastolic (D wave), and atrial reversal (AR wave) flow, tricuspid E and A waves, and hepatic vein flow waves. Progressive impairment of diastolic function leads to different patterns of mitral, tricuspid, pulmonary, and hepatic venous Doppler flow patterns, as well as changes to myocardial imaging by tissue Doppler imaging. Before further description of the flow patterns in RCM and their interpretation, it is important to briefly cover assessment of diastolic function. The assessment of diastolic function by echocardiography remains challenging in pediatrics. Guidelines and recommendations are derived from adult studies and guidelines, which fail to accurately assess pediatric diastolic dysfunction. In a study that applied adult diastolic dysfunction criteria to pediatric cardiomyopathy patients, Dragulescu et al. found: (i) a high percentage of normal diastolic measures in children with overt (often severe) cardiac dysfunction; (ii) abnormal diastolic measures in normal controls; (iii) frequent discordance between e´ and left atrial volume; (iv) discordant and overlapping criteria precluding precise grading of diastolic function in most patients; and (v) poor interobserver variability agreement [16]. Despite such limitations, we are still left trying to apply adult paradigms to pediatric patients, including the simplified progression of diastolic dysfunction from impaired relaxation, to pseudo‐normalization, to restrictive physiology [17]. However, new guidelines in adults were recently released with the goal of simplifying the approach to diastolic assessment (Figure 37.5) [18]. The four recommended diastolic variables to assess and the abnormal cut‐off values (for adults) are [18]: Figure 37.3 Parasternal short‐axis view in restrictive cardiomyopathy demonstrating systolic flattening of the interventricular septum (arrow) indicative of pulmonary hypertension. This patient also has pericardial effusion from increased filling pressure. LV, left ventricle; PE, pericardial effusion; RV, right ventricle. Figure 37.4 Subxiphoid view of a markedly dilated inferior vena cava (IVC), reflecting increased right atrial pressure. The number of positive variables determines whether diastolic dysfunction is present; additional measures are recommended in indeterminate cases. To estimate left ventricular filling pressure, mitral inflow along with tissue Doppler imaging and left atrial volume, can be assessed (Figure 37.6) [18]. An E/A ≤0.8 and E ≤50 cm/s suggests normal left atrial pressure and grade 1 diastolic dysfunction, with Doppler flow patterns commonly referred to as impaired relaxation. An E/A ≥2 suggests increased left atrial pressures, with grade 3 diastolic dysfunction. If E/A ≤0.8 and E >50 cm/s, or 0.8 < E/A < 2, additional criteria are needed to define diastolic function: (i) an average (between septal and free wall) E/e´ >14; (ii) tricuspid regurgitation velocity >2.8 m/s; and (iii) indexed left atrial volume >34 mL/m2. If two to three of the three criteria are present, it is consistent with grade 2 diastolic dysfunction [18]. Figure 37.5 Algorithm for diagnosis of left ventricular diastolic dysfunction in adults with a normal ejection fraction. E, early transmitral inflow; e´, early myocardial velocity by tissue Doppler imaging at the mitral annulus; TR, tricuspid regurgitation. Source: Adapted from Nagueh SF, Smiseth OA, Appleton CP, et al. Recommendations for the evaluation of left ventricular diastolic dysfunction by echocardiography: an update from the American Society of Echocardiography and the European Association of Cardiovascular Imaging. J Am Soc Echocardiogr 2016;29:277–314. It is important to remember that in young children, especially infants, the mature Doppler patterns of blood flow are not established, making the confident evaluation of diastolic function more challenging than in adults. Despite these limitations, with our growing understanding of myocardial mechanics, diastole should not be ignored in children. Evaluation of relaxation using all of the available tools is important to help identify isolated diastolic dysfunction – such as in RCM – and to monitor the patient’s disease state. Further work to develop clear measures of diastolic function in children is ongoing. Early diastolic dysfunction, grade 1, has Doppler flow patterns often referred to as impaired relaxation [15,18]. In this setting, there is reversal of the normal transmitral E and A waves, with E < A (E/A ratio <0.8) (Figure 37.7). Pulmonary venous flow is marked by reversal of normal S and D waves(D > S normally), with S > D in adults. With progression of diastolic dysfunction, E > A ratio is restored (pseudo‐normalization). With progression to grade 3 (restrictive physiology), often seen in RCM, the E is more prominent with smaller A, and an E/A ratio >2 is present, with shortened deceleration time (DT) (<160 ms; Figure 37.8) and isovolumic relaxation time. In contrast to constrictive pericarditis, there is no significant change in the mitral E wave, DT, or isovolumic relaxation time with respiration (Figure 37.9). Pulmonary venous flow progresses to predominantly D‐wave inflow in restrictive physiology. In addition, increased A‐wave reversal velocity and duration are present, with A reversal duration greater than mitral A duration (Figure 37.10). In contrast to a constrictive pattern, there is no significant respiratory variation of the D wave. Tricuspid inflow patterns are similar to mitral inflow with restriction characterized by an increased E/A ratio and a shortened DT (Figure 37.11). Hepatic venous flow is similar to pulmonary vein flow, with prominent atrial and ventricular reversal with restriction (Figure 37.12), and increased reversal waves with respiration. Figure 37.6 Algorithm for assessment of left atrial (LA) pressure and diastolic dysfunction grading in adults with depressed ejection fraction and those with myocardial disease and normal ejection fraction. A, late transmitral inflow; E, early transmitral inflow; e´, early myocardial velocity by tissue Doppler imaging at the mitral annulus; TR, tricuspid regurgitation. Source: Adapted from Nagueh SF, Smiseth OA, Appleton CP, et al. Recommendations for the evaluation of left ventricular diastolic dysfunction by echocardiography: an update from the American Society of Echocardiography and the European Association of Cardiovascular Imaging. J Am Soc Echocardiogr 2016;29:277–314. Figure 37.7 Mitral valve inflow Doppler showing predominantly late diastolic filling of the left ventricle, with E/A wave reversal (E < A). A, late diastolic wave; E, early diastolic wave. Figure 37.8 Mitral valve inflow Doppler of a patient with restrictive physiology. A large E wave and small A wave with an E/A ratio >2 and a short deceleration time of 93 ms are characteristic of a restrictive physiology. A, late diastolic wave; E, early diastolic wave. Figure 37.9 Mitral inflow Doppler showing no respiratory variation in restrictive cardiomyopathy, as opposed to constrictive pericarditis. A, late diastolic wave; E, early diastolic wave. It is important to remember that these measurements are dependent on loading conditions and assume that the patient is in sinus rhythm. Figure 37.10 Note the prominent A reversal of the pulmonary vein Doppler with both increased amplitude and prolongation (corresponding to the numbers 3 and 4, respectively). The A reversal width is greater than the mitral A‐wave duration in the corresponding patient (same patient as in Figure 37.7). A, atrial reversal wave; D, diastolic wave; S, systolic wave. Figure 37.11 Tricuspid inflow of a patient with restrictive cardiomyopathy showing a large E wave and a small A wave. A, late diastolic wave; E, early diastolic wave. Color M‐mode offers a unique view into the fluid dynamics of left ventricular inflow. The velocity of propagation (Vp) assesses the flow propagation from the mitral valve into the left ventricle, which is dependent on intraventricular pressure gradients, by measuring the slope of the color aliasing velocity at the leading edge of the E wave (Figure 37.13). Vp has been shown to correlate with the time constant of left ventricular relaxation (τ, tau) in patients with depressed left ventricular ejection fraction, but can be misleadingly normal in patients with normal left ventricular volumes and ejection fraction but with known elevated left ventricular filling pressures (as is commonly seen in RCM) [15,18]. Vp is usually decreased in RCM compared with normal patients and also those with constrictive pericarditis. This technique is limited by feasibility and reproducibility, and so is not routinely used in many echocardiography labs. Figure 37.12 Hepatic venous Doppler in restrictive cardiomyopathy demonstrating prominent A‐wave reversal. A, late diastolic wave; D, diastolic wave; S, systolic wave. Using Doppler principles, the movement of the myocardium can be evaluated by tissue Doppler imaging (TDI), which is less load sensitive than blood pool Doppler parameters. Left ventricular filling pressure has minimal effect on the early mitral annular velocity (e′ wave) in the presence of impaired left ventricular relaxation [15,18]. Patients with restrictive cardiomyopathy have an abnormality of both relaxation and compliance, and so typically have reduced (<8 cm/s) lateral and septal e´ (Figure 37.14), with reversal of the e′ and a′ pattern (e´ < a´; Figure 37.15). Early myocardial velocities remain depressed with progression of diastolic dysfunction. In adults, measurement of the ratio of the Doppler transmitral E wave to the tissue Doppler mitral e′ wave (E/e′ ratio) is useful in the assessment of left ventricular filling pressure, with an E/e′ ratio >14 consistent with diastolic dysfunction [18]. In children, however, E/e´ may not correlate with left ventricular end‐diastolic pressure even when elevated, and may even be normal in those with clear diastolic dysfunction [16,19]. Strain represents the relative deformation of the myocardium and strain rate is the rate of myocardial deformation [20]. Strain, a dimensionless measure, represents the percentage change from the original or unstressed dimension and includes both lengthening or expansion (positive strain) and shortening or compression (negative strain). Compared with tissue velocities, these parameters are less influenced by cardiac motion or tethering, are angle independent, and allow more accurate assessment of segmental myocardial function. Strain can demonstrate early impairment in systolic function at a time when fractional shortening remains normal [21]. Global longitudinal strain is decreased in RCM (Figure 37.16), although circumferential and radial strain are often normal; rotational mechanics are typically preserved [1,22,23]. Atrial strain has also proven helpful, with left atrial strain during reservoir phase correlating with left ventricular end‐diastolic pressure more accurately than indexed left atrial volume [24]. Left atrial septal reservoir strain and strain rate are significantly lower than in constrictive pericarditis [25]. Although typically not performed as a primary imaging modality in RCM, transesophageal echocardiography (TEE) typically affords clear imaging windows, and so may add benefit over transthoracic echocardiography in patients with poor transthoracic windows, such as young adults. It also provides a superior evaluation of, and angle of interrogation for, pulmonary vein flow. Transgastric windows can offer a direct visualization of the pericardium when transthoracic windows are limited. However, TEE in pediatrics requires the use of anesthesia, which limits its applicability and access. Electrocardiography may show depolarizing abnormalities such as a bundle branch block, ventricular hypertrophy, and impaired atrioventricular conduction. A chest X‐ray may show cardiomegaly, enlarged atria with prominent right heart border, and enlarged carina angle. Cardiac catheterization can also help in the distinction with evaluation of hemodynamics, with elevated ventricular filling pressures (left > right), and pulmonary hypertension often present. Figure 37.13 Color M‐mode of (a) a normal patient, and (b) a patient with restrictive cardiomyopathy, demonstrating decreased inflow propagation velocity (Vp). Computed tomography (CT) and cardiac magnetic resonance imaging (MRI) imaging are useful to help differentiate RCM from constrictive pericarditis by assessing pericardial thickness, the hemodynamics of constriction, and any myocardial abnormalities. Some forms of RCM are associated with characteristic cardiac MRI findings, such as endomyocardial fibrosis. Cardiac MRI can reliably quantify iron loading in patients with hemochromatosis through the T2 mapping technique (shortened T2 relaxation time) [26]. Figure 37.14 Tissue Doppler imaging in a patient with restrictive cardiomyopathy showing (a) decreased e′ velocity <8 cm/s and (b) prominent e′ and small a′ waves. a′, late diastolic wave; e′, early diastolic wave; s′, systolic wave. Constrictive pericarditis (CP) is defined as inflammation, thickening, and fusion of the thin and easily distensible parietal and visceral pericardial layers, resulting in impaired venous return and ventricular filling of the heart [27]. The process is typically indolent and slowly progressive, with clinical manifestation usually appearing months or even years after the initial inflammatory insult. Constrictive pericarditis is very rare and sporadic in the pediatric population [28]. Different etiologies can produce variable clinical findings, depending on the rapidity and severity of development (Table 37.2). The disease is most commonly a complication of acute pericarditis, either a single intense episode or multiple recurrent flares [29]. The underlying inciting event frequently varies with the ethnicity and country of origin of the patient. Tuberculous pericarditis is the most frequent known cause of chronic constrictive pericarditis worldwide, although mediastinal irradiation and cardiac surgery are the most common causes of constriction in Europe and North America [30]. The diagnosis of constrictive pericarditis is often made after an extensive diagnostic work‐up in the setting of failure of conventional medical therapy for congestive heart failure. Life expectancy is reduced in untreated children and in patients with relatively acute onset of symptoms [31]. There is no statistical difference between race and gender in the prevalence of the disease. Figure 37.15 Tissue Doppler imaging showing reversal of e′ and a′ waves with e′ < a′. Figure 37.16 Speckle‐tracking echocardiography longitudinal strain assessment demonstrating global decrease in longitudinal strain. Table 37.2 Causes of constrictive pericarditis In CP, the size of the heart is usually normal. The pericardium typically shows inflammation, fibrosis, thickening, and obliteration of the space between the visceral and parietal layers. Adhesions also occurs between the pericardium and myocardium. The pericardial thickening and adhesions may be focal or diffuse, although pericardial wall thickness is normal in up to 20% of cases. Calcifications may also be present. Myocardial histologic findings include fibrotic thickening, chronic inflammation, granulomas, and calcification. Development of CP results in decreased cardiac compliance associated with diminished ventricular distensibility, inability to maintain adequate preload, and biventricular diastolic dysfunction. Notably, the presentation of RCM can appear very similar to CP. Both have reduced left ventricular compliance, but where RCM is related to abnormal myocardial properties with impaired relaxation, CP is related to external pericardial constraint with typically normal myocardial relaxation [9]. As a result, measures of myocardial function are typically abnormal in RCM, though often more normal in CP, except potentially at surfaces in contact with the possibly adherent pericardium. As a result of encasement in a rigid pericardium, as the ventricles fill, they meet the inelastic resistance of the stiff, often calcified, pericardium, at which time filling pressure rises rapidly to an elevated plateau. This late diastolic phenomenon is due to a change in the compliance (volume/pressure) curve, such that a small increase in volume results in a considerable increase in end‐diastolic pressure. CP typically results in pronounced ventricular interdependence, evidenced by dramatic respiratory variation in ventricular filling parameters (mitral, tricuspid, pulmonary venous, and hepatic flows) and ventricular septal position due to dissociation between intracardiac and intrathoracic pressures as a result of pericardial rigidity. Importantly, RCM lacks this respiratory variation. Although historically there has been some difficulty in using echocardiography to separate the diagnosis of CP and RCM, newer strategies have improved the ability to differentiate the two (Table 37.3) [9,22,23]. Fluid bolus and Valsalva maneuver can potentially augment some of these differences. In CP, lateral and septal e´ are normal or even increased, and unlike controls, septal e´ is greater than lateral e´ [32,33]. Speckle‐tracking echocardiography adds diagnostic value, as RCM has diffuse abnormal longitudinal mechanics with relative sparing of circumferential and rotational mechanics, where CP has relatively preserved septal longitudinal mechanics but abnormal circumferential and rotational mechanics; the ratio of left ventricular free‐wall and septal longitudinal strain also being helpful to differentiate RCM and CP [25,34]. There remains, however, some overlap in variables between CP, RCM, and controls, making it difficult to use a particular cutoff for a single value to establish CP from RCM, though combining variables may lead to less overlap and improve diagnostic accuracy [19,23,35]. Septal e´ appears to have the better diagnostic value than most parameters [23,33,35,36]. Lastly, there is a small subset of patients with mixed pathology, with evidence of both constrictive pericarditis and myocardial restriction, making diagnosis in this population difficult. Differentiation of CP from RCM remains important as there is a surgical therapy for CP in pericardiectomy. Invasive hemodynamics may also differentiate between the two, with left greater than right ventricular end‐diastolic pressure in RCM, whereas in CP pressures may equalize (<5 mmHg difference) or reverse during respiratory cycle [37]. In addition, pulmonary hypertension is uncommon in CP, though frequently encountered in RCM [37]. Biomarkers such as brain natriuretic peptide (BNP) and NT‐proBNP are elevated in RCM, though not in CP [1]. Echocardiography remains the best tool in the initial assessment of constrictive pericarditis [13]. A combination of 2D imaging, M‐mode, and Doppler information is capable of diagnosing the anatomic and pathophysiologic features of constrictive pericarditis in most patients. Table 37.3 Echocardiographic differences between constrictive pericarditis and restrictive cardiomyopathy (highlighted in italic) Source: Adapted from Lipshultz SE, Law YM, Asante‐Korang A, et al. Cardiomyopathy in children: classification and diagnosis. A scientific statement from the American Heart Association. Circulation 2019;140:e9–e68.
CHAPTER 37
Restrictive Cardiomyopathy and Pericardial Diseases
Restrictive cardiomyopathy
Introduction
Incidence and etiology
Pathophysiology
Imaging
2D echocardiography
Idiopathic
Genetic:
Sarcomeric mutations
Desmin mutations
Filamin‐C mutations
Endomyocardial fibrosis:
Parasitic infection
Autoimmune disorders
Possible dietary deficiency or ingested toxins
Hypereosinophilic syndrome (Löffler endocarditis)
Infiltrative:
Amyloidosis
Sarcoidosis
Iron overload:
Hemochromatosis
Hemosiderosis
Lysosomal storage diseases:
Fabry disease
Gaucher disease
Hurler syndrome
Glycogen storage disease
Carcinoid syndrome
Secondary to radiation therapy
Secondary to anthracycline administration
Flow characteristics
Diastolic function
Color M‐mode
Tissue Doppler imaging
Speckle‐tracking echocardiography
Additional modalities
Cardiac computed tomography and cardiac magnetic resonance imaging
Pericardial diseases
Constrictive pericarditis
Introduction
Incidence and etiology
Idiopathic
Post‐acute pericarditis
Tuberculosis
Mediastinal radiation
Status post‐cardiac surgery (post‐pericardiotomy syndrome)
Infectious:
Viral
Bacterial: staphylococci, streptococci
Fungal: histoplasmosis
Rheumatoid disease
Sarcoidosis
Trauma (hemopericardium)
Uremia
Neoplasia with pericardial infiltration
Metabolic and genetic disorders
Pathophysiology
Differentiating constrictive pericarditis and restrictive cardiomyopathy
Imaging
Constrictive pericarditis
Restrictive cardiomyopathy
Two‐dimensional echocardiography
Left ventricular ejection fraction
Normal
Normal or slightly decreased
Ventricular septal movement
Abnormal, varies with respiration
Normal, no respiratory variation
Pericardial appearance
Likely thickened, bright
Normal
Atrial appearance
Normal or mildly dilated
Significant biatrial dilation
Doppler echocardiography
E/A ratio
Increased (≥2)
Increased (≥2)
E/A ratio response to Valsalva
>25% variation
Minimal variation
E wave deceleration time
Decreased (<160 ms)
Decreased (<160 ms)
e´ lateral
Normal
Decreased
e´ septal
Normal to increased (≥8 cm/s)
Decreased (≤8 cm/s)
e´ septal : lateral
Sepal > lateral
Lateral > septal
s´ septal
>5 cm/s
<5 cm/s
Lateral e´ timing vs mitral E
Shorter (e´ often earlier than E)
Prolonged (e´ after E)
E/e´
<15
>15
Mitral valve inflow propagation (Vp)
Normal or increased (>100 cm/s)
Decreased (<55 cm/s)
Hepatic vein flow
Diastolic flow reversal during expiration
Diastolic flow reversal during inspiration
Speckle‐tracking echocardiography
Longitudinal strain:
Septal
Normal
Decreased
Lateral
Decreased
Decreased
Basal segments
Normal
Decreased
Apical segments
Decreased
Decreased
Global
Decreased
Decreased
Circumferential strain
Decreased
Normal
Radial strain:
Basal
Decreased
Normal
Apical
Normal
Normal
Apical rotation
Decreased
Normal
Twist motion
Decreased
Normal
Left atrial reservoir strain:
Septal
Increased
Decreased
Lateral
Decreased
Decreased
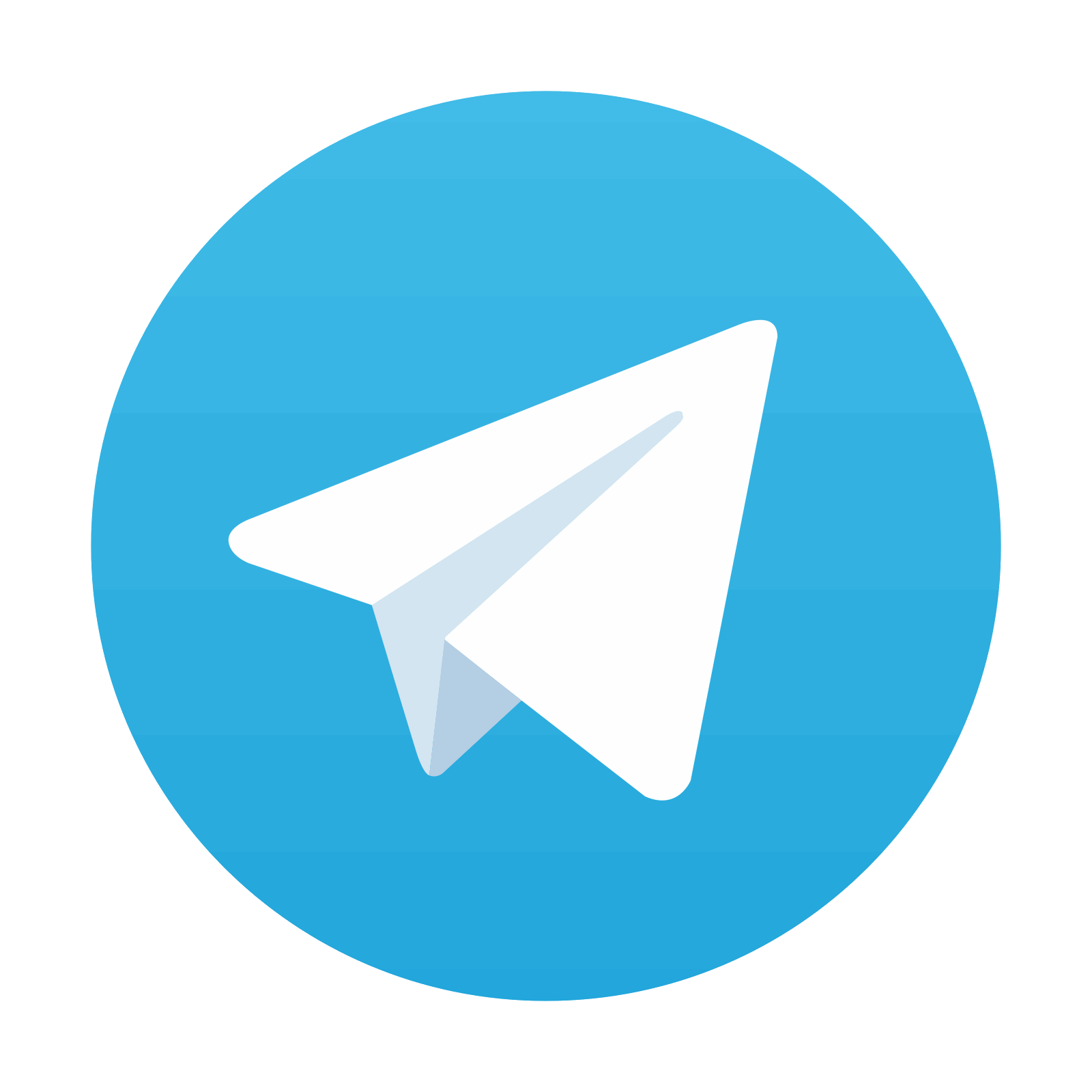
Stay updated, free articles. Join our Telegram channel

Full access? Get Clinical Tree
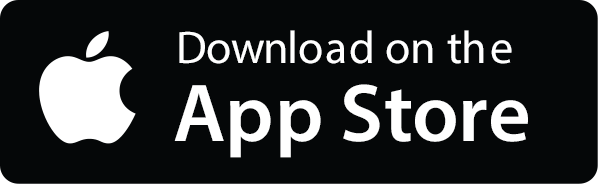
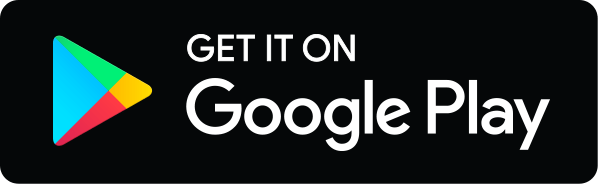