Marc Gewillig1 and Luc L. Mertens2 1 University Hospitals Leuven, Leuven, Belgium 2 The Hospital for Sick Children; University of Toronto, Toronto, ON, Canada For the majority of patients with different types of functionally univentricular hearts, the treatment goal is to achieve palliation through the creation of a total cavopulmonary connection known as the Fontan operation. This operation essentially bypasses the right heart by redirecting the systemic venous return directly into the pulmonary circulation without passing through a ventricular chamber. The Fontan operation creates a unique circulatory physiology, which resulted in improved survival at the expense of long‐term complications. Evaluating a Fontan patient requires a specific echocardiographic approach and careful interpretation of the findings. In this chapter we first review the physiologic characteristics of a Fontan circulation followed by a discussion of the echocardiographic evaluation. A Fontan circulation is offered to patients with severe congenital heart disease who have only one functional single ventricle (FSV) or those in whom the ventricular cavity cannot be partitioned into two adequate pumps. During fetal life and immediately after birth, the systemic and the pulmonary circulations in an FSV are connected in parallel with one ventricular chamber pumping to both circulations (Figure 29.1). Initially these patients are palliated by an aortopulmonary artery shunt (e.g., a Blalock–Taussig shunt or ductal stent), right ventricular (RV) to pulmonary artery (PA) shunt (also termed a Sano‐type shunt), or by PA banding. All these procedures result in persistence of the parallel circulations with mixing of saturated and desaturated blood causing arterial desaturation as well as chronic volume loading of the FSV. In the pre‐Fontan era this was the only type of palliation that could be offered, often requiring upscaling the shunts or loosening the PA band. These procedures were associated with progressive ventricular dysfunction, heart failure, progressive desaturation, and death, with few survivors beyond the fourth decade. In 1971, Francis Fontan, a cardiac surgeon in Bordeaux, France, reported on a novel surgical approach to single ventricles [1], separating the systemic and pulmonary circulations by redirecting the systemic venous blood directly to the pulmonary circulation. In the Fontan circulation, mixing of the circulations is abolished and the postcapillary energy and systemic venous pressure are used as the driving forces to propel the blood through the pulmonary circulation [2]. Advantages of a Fontan circuit include separation of both circulations with normalization of the arterial saturation and significant ventricular volume unloading [3,4]. Initially, different types of connections were used to redirect the systemic venous blood to the pulmonary circulation; however the Fontan operation has been simplified by connecting the caval veins directly to the pulmonary arteries. This is achieved by first performing a bidirectional Glenn shunt by direct anastomosis of the superior vena cava to the pulmonary artery and in a second stage by connecting the inferior vena cava through an intra‐ or extracardiac conduit to the pulmonary artery. A modern Fontan operation is thus an extracardiac operation. The early changes observed at ventricular level are not related to cardiac surgery but are secondary adaptations to the new physiologic stage. After Fontan surgery an additional upstream flow restrictor determines the inflow to the FSV as, by creating a Fontan circuit, the pulmonary circulation is positioned, like a portal system, between the systemic venous return and the systemic ventricle. A portal system is defined as a capillary network that is interposed between another capillary network and the heart. This pulmonary portal system now functions like a dam in the cardiovascular circuit, resulting in upstream congestion and reduced downstream flow. The upstream energy is used to push blood through the pulmonary circulation, which provides systemic oxygenation. The pulmonary circulation thus becomes the main limiting factor in the circulation. This results in pooling of systemic venous blood, increased systemic venous pressure, and reduced cardiac output, which are the basis for the most frequent early and late complications of the Fontan circuit (Figure 29.2). Figure 29.1 Schematic representation of the normal cardiovascular circulation (a), shunted palliation (b), and Fontan circulation (c). (a) The normal circulation. The pulmonary circulation (P) is connected in series with the systemic circulation (S). The right ventricle (RV) is more compliant than the left ventricle (LV), keeping the right atrial (RA) pressure lower than the left atrial (LA) pressure. The RV functions at lower pressure than the LV which is related to lower pressure and resistance in the pulmonary artery (PA). (b) Parallel circulation in a univentricular heart. The systemic (S) and pulmonary (P) circuits are connected in parallel with one dominant single ventricle (V) pumping blood into both circulations. There is complete admixture of systemic and pulmonary venous blood, causing arterial desaturation. (c) Fontan circuit. The caval veins (CV) are connected to the PA, without a subpulmonary ventricle or systemic atrium: the lungs are hereby converted into a portal system which limits flow to the ventricle. In the absence of a fenestration, there is no admixture of systemic and pulmonary venous blood, but the systemic venous pressures are generally elevated. Ao: aorta. Line thickness reflects output; color reflects oxygen saturation. Figure 29.2 Cardiac output during exercise: normal versus Fontan circulation. A normal subject with a biventricular circuit can increase output five‐fold compared to baseline (black line). In Fontan patients output is significantly impaired both at rest and during exercise. In optimal Fontan patients (green line) the output is mildly decreased at rest, with a moderately reduced capacity to increase cardiac output during exercise. This limits exercise capacity in almost all Fontan patients. In failing Fontan patients (red line), the output is significantly reduced at rest with very limited increase during exercise. The optimal Fontan circuit requires unobstructed Fontan connections, good‐sized branch PAs without stenosis, low pulmonary vascular resistance (PVR), unobstructed pulmonary venous connections to the atrium, and low atrial pressures. Atrial pressures are influenced by atrioventricular (AV) valve function and by FSV diastolic function. Good systolic FSV function is required to generate sufficient systemic output. Chronic volume loading caused by residual shunts, AV valve regurgitation, or aortic regurgitation and/or pressure loading related to outflow tract obstruction, residual coarctation of the aorta, or arterial hypertension can be detrimental in patients after Fontan surgery. The FSV volume load evolves from excessive during fetal life and initial palliation to chronic deprivation after creation of the cavopulmonary shunts (Figure 29.3). During fetal life, the dominant ventricle provides the combined output of the systemic and pulmonary circulations. This causes prenatal eccentric remodeling that results in enlarged chamber dimensions of the FSV at birth (large for body surface area (BSA)) [5]. After the initial neonatal palliation (generally an aortopulmonary artery shunt or a PA band), the parallel circulations cause a persistent, chronic volume loading. Between 4 and 8 months of age, the next step typically consists of dividing the initial shunt (which will normalize the preload for BSA) and creating a bidirectional Glenn shunt (a superior cavopulmonary anastomosis where only the superior vena cava is connected to the PA and the inferior vena cava remains connected to the right atrium), which reduces the pulmonary flow. A serial follow‐up study using cardiac MRI in patients scanned before the bidirectional Glenn shunt and again before the Fontan operation demonstrated that the bidirectional Glenn shunt resulted in a reduction of indexed ventricular volume with an increase in ejection fraction (i.e., reverse remodeling) [6]. Quantitative measurements by cardiac MRI have also demonstrated that flow through aortopulmonary collaterals may contribute significantly to pulmonary blood flow and cardiac output after the bidirectional Glenn shunt [7]: up to one‐third of the cardiac output and up to 40% of the pulmonary blood flow comes from collateral flow. A higher burden of collateral flow adversely influences clinical outcomes immediately after the Fontan operation [7,8]. Despite the presence of collaterals, the FSV is further volume unloaded after the Fontan completion by damming off the inferior caval flow. After the Fontan operation, further reverse remodeling of the FSV occurs, characterized by a decrease in ventricular volume with persistence of an abnormal wall thickness (eccentric remodeling) and resulting in increased mass‐to‐volume ratio [9]. Concentric hypertrophy may also develop in the FSV in response to increased afterload, defined as ventricular wall stress. Studies have suggested that increased afterload (i.e., outflow tract obstruction, residual coarctation of the aorta, or systemic hypertension) may persist or develop after the Fontan operation [10,11]. Residual ventricular hypertrophy may contribute to diastolic dysfunction and eventually to failure of the Fontan circulation. Figure 29.3 Effect of different pre‐Fontan palliative strategies on volume loading. A: We assume a normal ventricle in a biventricular circulation functions on average at 100% of normal. Prior to birth the dominant single ventricle remodels and its size adjusts to the chronic volume loading. The patient with a univentricular heart generally is born with an appropriate ventricular size adjusted for chronic volume load. Prior to the 1990s (B, red line), the volume loading to the ventricle was augmented shortly after birth by a shunt procedure to ± 150%. The patient slowly outgrew the shunt, and the ventricle adapted, thereby gradually reducing the volume overload to ± 100% for its size. A second shunt was created, augmenting the volume overload again to 150%. As the patient again outgrew the shunt, a Fontan circuit was made, all of a sudden reducing the volume load to around 25% of the preload it was exposed to prior to the procedure. This sudden preload reduction was often poorly tolerated hemodynamically. This resulted in changes in management strategy. After the 1990s (C, green line), a small neonatal shunt is created for a short time (generally 6 months); the patient slowly outgrows the shunt; the ventricle is progressively volume unloaded in two separate steps: first at the time of the bidirectional Glenn shunt and a second time at the time of the Fontan operation. This stepwise approach is generally hemodynamically better tolerated. The most commonly used parameter to assess global pump function is ejection fraction. This parameter is sensitive to changes in preload and afterload. Serial data obtained with either MRI or echocardiography have demonstrated a decrease in ventricular volumes and ejection fraction immediately after the bidirectional Glenn shunt [6,12]. During the remodeling process a compensatory decrease in end‐systolic volumes may result in an increase in ejection fraction just prior to the Fontan procedure [6]. Despite these findings, ejection fraction is generally preserved in the majority of patients after the Fontan operation suggesting that adaptation or further reverse remodeling occurs in the post‐Fontan period [9]. It also indicates that systolic dysfunction is uncommon in pediatric Fontan survivors. In contrast, in adult patients, ventricular dysfunction seems to be more common and contributes to the failure of the Fontan circuit [13]. Systolic dysfunction may be intrinsic to the FSV or may be related to other comorbidities in adult life. In the Fontan circuit, low atrial pressure is required for a low gradient across the pulmonary vascular bed (transpulmonary gradient). AV valve regurgitation and FSV diastolic function are important determinants of atrial pressure. Diastolic function after the Fontan operation has been poorly studied due to the absence of good methods for assessment of ventricular filling in patients with this physiology. Experimental data have shown that acute volume unloading of the ventricle results in less recoil and decreased suction, resulting in increased filling pressures (Figure 29.4) [14]. Echocardiographic studies in Fontan patients have suggested that the volume reduction associated with the Fontan operation results in early relaxation abnormalities and incoordinate wall motion abnormalities especially affecting the isovolumetric relaxation period and early filling [15–17]. These diastolic changes have typically been observed in FSV patients who underwent a Fontan operation without a previous bidirectional Glenn shunt. In this era, the chronically volume‐loaded ventricles were acutely unloaded and the observed diastolic and systolic abnormalities could be related to the ventricle being “too large” for the volume immediately after the procedure. In patients after the Fontan procedure, chronic volume reduction with absence of exercise‐induced ventricular stretch seems to cause a progressive decrease in ventricular compliance with a left and upward shift in the end‐diastolic pressure–volume relationship. It can be expected that the thicker myocardium may become stiffer with age. The FSV may enter a vicious circle whereby the volume unloading results in adverse reverse remodeling, reduced compliance, and reduced ventricular filling, further limiting cardiac output and increasing systemic venous pressures. A cohort study suggested that the majority of pediatric Fontan patients have abnormal diastolic parameters on echocardiography but the clinical and prognostic significance of these findings is currently unknown [9]. The main issue regarding assessment of diastolic function is that the conventional echocardiographic parameters (AV valve inflow, pulmonary venous flow patterns, and tissue Doppler velocities) are difficult to interpret in Fontan patients with a deprived preload. It is difficult to determine whether the observed changes in diastolic functional parameters are related to intrinsic myocardial diastolic dysfunction or are secondary to volume unloading. Typically, diastolic changes early after the Fontan operation are more likely secondary to unloading. Changes occurring over time are more likely related to changes in ventricular compliance. Progressive increase in ventricular stiffness and reduction in compliance might be one of the major mechanisms explaining Fontan failure with progressive age. Figure 29.4 Diastolic pressure–volume relationship during different stages of palliation. A: The normal ventricle. B: Shunted ventricle with chronic volume loading resulting in enhanced compliance. C: Fontan ventricle early after volume unloading. D: Fontan ventricle late after unloading: chronic volume unloading is thought to cause progressive stiffening of the ventricle with decreased compliance. BSA, body surface area; EDP, end‐diastolic pressure. A characteristic feature of the Fontan circuit is the lack of pulsatile pulmonary blood flow through the pulmonary vascular bed. In the normal biventricular circulation, PVR is generally not a limiting factor for determining cardiac output. In ischemic heart disease and cardiomyopathy, decreased cardiac pump function limits cardiac output. After the Fontan procedure the pulmonary circulation limits the preload reserve to the FSV and determines the cardiac output response [10,18]. This is comparable to obstructed inflow after Mustard repair, primary pulmonary hypertension, constrictive pericarditis, and mitral stenosis. Different factors influence the amount of pulmonary blood flow and cardiac output after the Fontan operation. The first limiting factor is the energy loss in the surgical connections. The presence of unobstructed surgical connections between the caval veins and the PAs is a requirement for optimal Fontan circulation function. Any degree of obstruction within the Fontan connections will reduce cardiac output. The PAs need to be good‐sized and unobstructed. PVR further determines pulmonary blood flow. Even a mild increase in PVR reduces pulmonary blood supply and cardiac output [19]. Pulmonary vasodilators like sildenafil positively influence cardiac output and exercise capacity in Fontan patients but to a limited extent [20–22]. Pulmonary venous obstruction is another important factor determining pulmonary blood flow. Some patients with FSV also have anomalous pulmonary venous connection that requires surgical intervention; they are at high risk for obstruction. The extracardiac Fontan conduit may also compress the adjacent right pulmonary veins. Further, the left lower pulmonary vein may become compressed between the heart, the descending aorta, and the spine. In the Fontan circuit, pulmonary blood flow will increase with inspiration and decrease with expiration following respiratory variations in intrathoracic pressure [23]. Studying respiratory variation in Fontan patients has provided interesting physiologic information. Increased intrathoracic pressure due to airway obstruction or positive pressure ventilation reduces pulmonary blood flow and is detrimental for Fontan physiology [24]. Negative pressure ventilation is considered beneficial and results in increased cardiac output. Since its original description, the Fontan circuit has undergone several surgical modifications (Figure 29.5) [25,26]. The original Fontan circuit in patients with tricuspid atresia included a valved homograft between the RA appendage and the right pulmonary artery in two patients and direct anastomosis without a conduit in one. The use of valved conduits in the Fontan circulation was found to be associated with a high degree of stenosis, and the direct atriopulmonary connection became the most commonly used modification in the 1980s. It was thought that including the right atrium in the Fontan connection would be beneficial for pulmonary hemodynamics due to the presence of the atrial “kick.” However, elevated atrial pressure caused progressive right atrial dilation, reducing the energetic efficiency and becoming a substrate for atrial arrhythmia. Experimental and computational modeling was subsequently performed showing that a total cavopulmonary connection (TCPC) would be hemodynamically more favorable and would result in less energy and power loss in the conduit when compared to atriopulmonary connection [27,28]. In this circuit, the venae cavae are connected directly to the right pulmonary artery (RPA). The superior vena cava (SVC) is connected to the RPA (bidirectional Glenn shunt) and the inferior vena cava (IVC) is connected through an intra‐atrial (lateral tunnel) baffle or by an extracardiac conduit. The benefits of the lateral tunnel Fontan include use in small children and potential for growth. The potential drawback is that there is substrate for atrial arrhythmia as a result of the suture line in the atrium and the exposure of atrial tissue to high pressure. To avoid these problems, the extracardiac conduit was introduced. This procedure consists of a tube graft between the inferior vena cava and the PA placed externally around the right atrium. This circuit leaves the entire atrium at low pressure, has minimal atrial suture lines, and can be performed without aortic cross‐clamping. However, this tube graft has no growth potential and therefore is typically offered to larger patients (generally between 2 and 3 years of life). It remains to be determined whether the extracardiac conduit will decrease the arrhythmia risk and is hemodynamically favorable. When assessing a patient with a Fontan circulation, it is essential to know which type of connection has been made. Figure 29.5 Different modifications of the Fontan operation. (a) Original Fontan operation with valve graft from right atrium to hypoplastic right ventricle connecting to the pulmonary artery. (b) Atriopulmonary connection. The right atrium is connected to the pulmonary artery. (c) Total cavopulmonary connection with intra‐atrial tunnel. (d) Total cavopulmonary connection with extracardiac conduit. In the newborn period it is impossible to create a Fontan circulation because of the elevated PVR, which progressively decreases during the first weeks after birth. Even when PVR falls, a staged approach to Fontan completion is preferred, with the superior and inferior caval veins incorporated into the systemic venous chamber in two separate stages. Thus, the body adapts progressively to the new hemodynamic state and ventricular unloading is staged, reducing the operative morbidity and mortality. In the neonatal period, clinical management aims at achieving unrestricted flow to the aorta (when required: arch repair, Damus–Kaye–Stansel, Norwood procedure), appropriately limited flow to the lungs (when required: PA band, aortopulmonary shunt, stenting of the arterial duct), and unrestricted return of blood to the ventricle (if required: balloon septostomy, pulmonary venous connection). Between 4 and 8 months of age, the bidirectional Glenn shunt is performed. After the Glenn shunt, the patient remains mildly cyanotic because the desaturated blood from the IVC still connects to the systemic ventricle and the aorta. In infants the distribution of blood flow between the upper and lower body is around 2 : 2 which helps the efficiency of the bidirectional Glenn shunt in the first 2 years of life. As the lower body grows faster than the upper body this ratio changes and is reversed in adults with around twice as much blood coming from the IVC compared to the SVC. Typically, the Fontan circuit is completed between 2 and 5 years of age, incorporating the IVC into the Fontan circuit. At the time of Fontan completion, often a small fenestration is created between the systemic venous pathway and the pulmonary venous atrium, either routinely or only in “high‐risk” patients. The fenestration allows a residual right‐to‐left shunt, thereby reducing systemic venous pressure and increasing preload of the systemic ventricle at the expense of cyanosis. A fenestration has been shown to reduce operative mortality and morbidity (e.g., pleural drainage). It can be closed by a percutaneous intervention weeks or months after adaptation of the body to the new hemodynamic condition, particularly if marked cyanosis occurs with exercise. Complications after the Fontan operation are common and relate to the increased systemic venous pressure and chronic low cardiac output. After surgery, there are clinically important early and late adverse outcomes, including mortality, exercise intolerance, ventricular dysfunction, rhythm and conduction abnormalities, hepatomegaly with secondary liver dysfunction, and potential for development of liver fibrosis or cirrhosis and adenocarcinoma, lymphatic dysfunction, protein‐losing enteropathy, plastic bronchitis, thrombus formation, ascites, and peripheral edema. Before scanning a patient who has undergone a Fontan operation, it is essential to first review the underlying cardiac anatomy and previous surgical history. The ventricular morphology (see Chapter 28) needs to be known as this influences measurements and interpretation of functional information. It is essential to be aware of the type of Fontan connection as well as any additional cardiac procedures before starting to scan. This includes information on previous arch surgery, the presence of a Damus–Kaye–Stansel (DKS) connection, previous valve surgery, type of previous shunt if performed (modified Blalock–Taussig shunt or other), interventions on the pulmonary arteries (e.g., patch arterioplasty, balloon dilatation, stent implantation), and interventions on the pulmonary veins. Additionally, the echocardiographer should know the clinical indication for the scan and the patient’s current clinical status, including absence or presence of symptoms and any specific clinical concerns. Some issues such as residual cyanosis, ascites, protein‐losing enteropathy, or other signs of Fontan failure may require specific image acquisition as detailed later. Access to previously performed imaging studies greatly facilitates interpretation of findings and detection of changes. Comparison between studies should be easy and should be routine in the review process. To facilitate this comparison, digital storage of clips and usage of standard scanning protocols is highly recommended. As these patients have frequently undergone multiple surgeries, acoustic windows may be challenging, especially with increasing age.
CHAPTER 29
Echocardiographic Assessment of Functional Single Ventricles after the Fontan Operation
Introduction
The Fontan circulation
Functional single ventricle in a Fontan circulation
Ventricular dimensions and wall thickness
Effect of loading changes on parameters of systolic function
Diastolic function of the functionalsingle ventricle
The pulmonary circulation limits cardiac output after the Fontan operation
Fontan modifications
Current strategy towards a Fontan circulation
Imaging
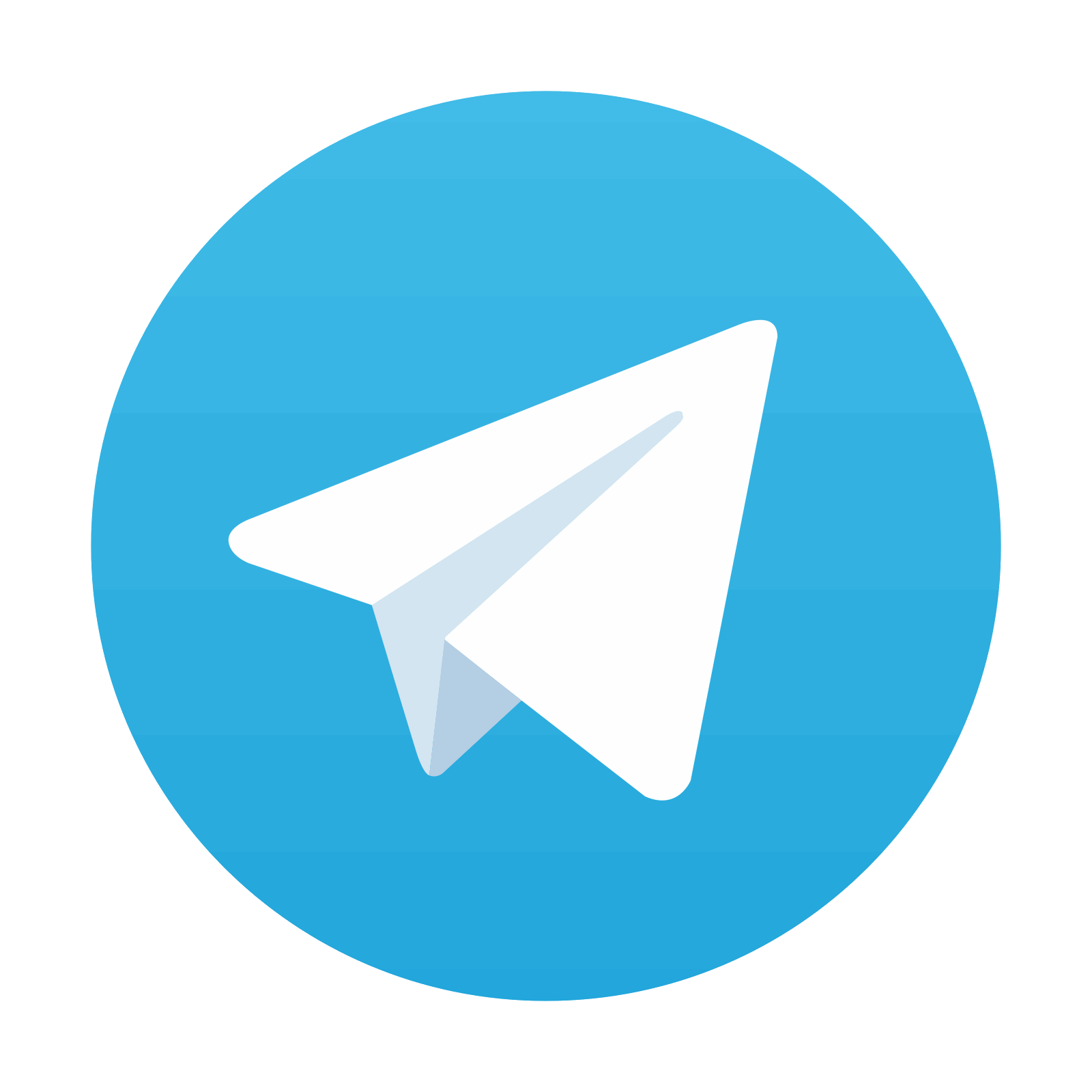
Stay updated, free articles. Join our Telegram channel

Full access? Get Clinical Tree
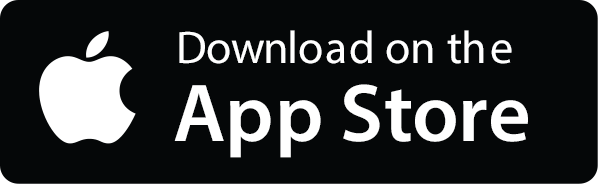
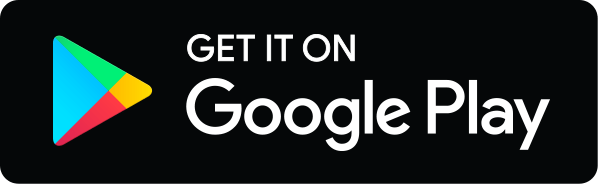