Timothy C. Slesnick1, Ritu Sachdeva1, Joe R. Kreeger2, Maria A. Pernetz3, and William L. Border1 1 Children’s Healthcare of Atlanta Sibley Heart Center; Emory University School of Medicine, Atlanta, GA, USA 2 Children’s Healthcare of Atlanta Sibley Heart Center, Atlanta, GA, USA 3 Emory Healthcare, Atlanta, GA, USA In 1942 Lev and Saphir defined truncus arteriosus as an anomaly in which a single arterial trunk leaving the heart gives rise to the aorta, pulmonary arteries, and coronary arteries [1]. This definition has persisted and is supported by other authors [2–5]. The earliest known descriptions of this defect were made by Wilson in 1798, as reported by Van Mierop et al. [6], and by Buchanan in 1864 [7]. Primary surgical repair in infants is the treatment of choice and is usually undertaken upon diagnosis. Perioperative mortality has decreased markedly over time, with current Society of Thoracic Surgeons (STS) data demonstrating under 5% mortality at many centers (aggregate operative mortality of 9.5%, interquartile range 0–15.4%) [8]. Truncus arteriosus is defined as an anomaly in which a single arterial trunk arises from the ventricles and gives rise to the aorta, pulmonary arteries, and coronary arteries. Although not part of the definition, an associated subtruncal ventricular septal defect (VSD) is found in the majority of patients with truncus arteriosus. This lesion is also called common arterial trunk. Truncus arteriosus occurs in approximately 1–4% of patients with congenital heart defects [2,3,5,9]. The estimated incidence of truncus arteriosus is 9–11 per 100,000 live birth [10]. Without surgical intervention, truncus arteriosus is usually fatal, with a mean age of death of 2.5 months [3], and 80% of children dying by one year of life [11]. The cause of death is typically the development of pulmonary vascular disease from longstanding unprotected pulmonary blood flow. Investigations using chick and mammalian embryos [12,13], as well as human studies, implicate a microdeletion at the 22q11.2 locus as at least one factor in the genetic etiology of truncus arteriosus. This microdeletion manifests clinically as DiGeorge syndrome (also known as velocardiofacial syndrome) [14]. Recent work suggests that TBX1 (T‐box transcription factor 1), which is located within the 22q11 locus, is essential in formation of the outflow tracts, and that disruption of TBX1 function may lead to a spectrum of cardiac disease, including truncus arteriosus [15]. The responsible gene within the 22q11.2 locus has not yet been definitively identified. Although the relationship between a chromosome 22q11 deletion and conotruncal lesions, including truncus arteriosus, is well established, preliminary studies suggest other putative mechanisms [13]. The single tube that comprises the outflow tract in embryos undergoes septation into the aorta and the pulmonary trunk by means of a structure originating from the neural crest, the aortopulmonary septum. This structure is affected in DiGeorge syndrome, resulting in truncus arteriosus. However, the remainder of the septation occurs by a different, less well understood mechanism, involving mesenchymal cells. It has been speculated that bone morphogenetic proteins may be involved in this process, and mouse embryo studies have demonstrated mutations in these proteins as causing truncus arteriosus and type B interrupted aortic arch [13]. Clearly, disruption of multiple embryologic steps is required for truncus arteriosus to develop. Patients with conotruncal anomalies are at increased risk for chromosome 22q11 microdeletion [16,17]. Fluorescence in situ hybridization (FISH) analysis for a chromosome 22q11 abnormality will detect a deletion in 35–40% of patients with truncus arteriosus [17–19]. Certain associated cardiac anomalies such as right aortic arch, abnormal aortic arch branching pattern, or a combination of these two features, place these patients at even higher risk for the deletion [19]. In addition to the cardiac defects, DiGeorge syndrome can be characterized by facial dysmorphism, thymic hypoplasia, parathyroid hypoplasia resulting in hypocalcemia, learning disabilities, and psychiatric disorders later in life. Patients with a 22q11 deletion and DiGeorge syndrome have been grouped under the unifying acronym CATCH 22 syndrome to emphasize their phenotypic components (cardiac defects, abnormal face, thymic hypoplasia, cleft palate, and hypocalcemia) [20]. Due to the high prevalence of a chromosome 22q11 microdeletion in patients with truncus arteriosus, the current recommendations are to screen all patients identified with this cardiac lesion. In the embryologic heart, two ridges that appear in the conotruncal segment at 4–5‐weeks’ gestation create the normal differentiation of the truncus into the aorta and pulmonary artery. These two ridges grow toward the midline and fuse to form the conotruncal septum which divides the truncus into the proximal aorta and main pulmonary artery [21]. Ectomesenchymal cells derived from the cardiac neural crest contribute directly to aorticopulmonary septation [22]. The absence of conal cushion tissue, which during normal embryogenesis fuses with the muscular part of the interventricular septum to complete ventricular septation, results in the typical infundibular or outlet VSD. Ablation of the cardiac neural crest in chick embryos results in truncus arteriosus, and partial ablation results in other conotruncal abnormalities, such as double‐outlet right ventricle, transposition of the great arteries, and tetralogy of Fallot [12]. The ectomesenchymal cells also contribute to the development of the pharyngeal arches and thus to the thymus and parathyroid glands. An alternative theory proposed by Van Praagh and Van Praagh [3] is that persistent truncus arteriosus is a form of tetralogy of Fallot combining pulmonary and infundibular atresia with failure of truncal separation – in essence an aortopulmonary window. Notably, neural crest abnormalities are found in tetralogy of Fallot, aortopulmonary window, and truncus arteriosus. However, in contrast to the aortic valve in tetralogy of Fallot, the truncal valve is often abnormal as described later in this chapter. Collett and Edwards classified truncus arteriosus into four major types based on the sources of pulmonary blood supply (Figure 24.1, top panel) [2]: Figure 24.1 Classification of truncus arteriosus by Collett and Edwards (top) and Van Praagh and Van Praagh (bottom). See text for details. The classification of Van Praagh and Van Praagh [3] (Figure 24.1, bottom panel) modified the original classification of Collett and Edwards and is more typically used: In each of the above types of truncus arteriosus, using the Van Praagh classification, a modifier “A” (with VSD) or “B” (intact ventricular septum) is used, although truncus arteriosus with no VSD is exceedingly rare. In the overwhelming majority of cases there is a subtruncal VSD over which the truncal valve sits, similar to tetralogy of Fallot. The VSD sits in the “Y” of the septal band or the septomarginal trabeculation. Rarely, the ventricular septum is intact [23,24]. The conal septum is absent and the truncal valve is typically in direct fibrous continuity with the mitral valve. In contrast to tetralogy of Fallot, the tricuspid valve is usually separated from the truncal valve by the muscularized posterior limb of the septal band. In rare circumstances, the truncal valve may be supported by a complete infundibulum and relates exclusively to the right ventricle. Figure 24.2 Parasternal short‐axis imaging plane demonstrating the quadricuspid morphology of the truncal valve. LA, left atrium; RA, right atrium; RV, right ventricle. One of the most common morphologic features of truncus arteriosus is an abnormal truncal valve. The truncal valve leaflets are often thickened because of the expansion of the spongiosa and fibrosa layers, and the leaflets are often described as nodular [25–27]. The number and morphology of the leaflets are variable [2–5]: trileaflet valve in 65–70% of patients, quadricuspid in 9–24% (Figure 24.2, Video 24.5), and bicuspid in 6–23%. Rarely, unicuspid [28] or pentacuspid valves are present. Truncal valve stenosis has been reported in ~33% of patients, and some degree of regurgitation is present in ~50% of patients (Video 24.6) [29]. In the majority of patients, the branch pulmonary arteries arise from the posterior‐left lateral aspect of the common trunk, either with a very short main pulmonary artery (type I/1; 48–68% of patients) or directly via two distinct orifices (type II/2; 29–48%) [2,4,5,28,30]. In ~6–10% of patients only one branch pulmonary artery arises from the ascending aspect of the common trunk (Van Praagh type 3). The coronary artery pattern in truncus arteriosus is variable. The most common abnormality includes a higher and more posterior origin of the left coronary artery, which may result in the origin being in close proximity to the origin of the pulmonary artery [31,32]. A single coronary artery has been reported in 13–18% of cases (Video 24.7). Importantly, the coronary ostia may be narrowed or slit‐like, resulting in significant coronary artery stenosis. Despite their highly variable origin, the distal coronary branches tend to follow a normal course [33]. There have also been reports of prominent diagonal branches off the right coronary artery; they parallel the course of the conus branch but are further down the anterior surface of the right ventricle. In addition, the conal branch off the right coronary artery may be prominent, distributing a network of vessels to the right ventricular outflow tract. The presence of coronary arteries in the infundibular free wall can complicate placement of the right ventricle‐to‐pulmonary artery conduit that is part of the surgical repair [34]. In addition to abnormalities of coronary ostial position, cases of an intramural course opening into the pulmonary artery have also been reported [35]. The cardiac anomalies most frequently associated with truncus arteriosus involve the aortic arch. The aortic arch is right‐sided in ~33% of patients [5]. Interrupted aortic arch occurs in approximately 19% of patients with truncus arteriosus (Van Praagh type 4) [36]. Conversely, approximately 12% of interrupted aortic arch anomalies have an associated truncus arteriosus. Type B interruption, between the left common carotid artery and the left subclavian artery, is the most common variant (Video 24.8). Other forms of interrupted aortic arch, such as type A, occur rarely (Video 24.4). An aberrant origin of the left or right subclavian artery (depending on aortic arch side) is common in patients with truncus arteriosus. A patent ductus arteriosus is almost uniformly absent in these patients except in those with an interrupted aortic arch. Whereas a left superior vena cava is found in approximately 12% of patients with truncus arteriosus, other anomalies of systemic and pulmonary venous return are less common. Retroaortic position of the left innominate vein is an anatomic variant of systemic venous anatomy associated with truncus arteriosus, particularly in those with a right‐sided aortic arch. Partially anomalous pulmonary venous return has been reported in approximately 1% of patients but total anomalous pulmonary venous connection is rare [3,4,37]. Other anomalies rarely reported to accompany truncus arteriosus include: secundum atrial septal defect, partial and complete atrioventricular canal defects, mitral atresia, mitral stenosis, tricuspid atresia, straddling tricuspid valve, Ebstein malformation, aortic atresia, hypoplastic left ventricle, double‐inlet left ventricle, heterotaxy syndrome, and left pulmonary artery sling [38,39]. The clinical presentation of truncus arteriosus is variable, but in most cases the dominant physiology is that of a large left‐to‐right shunt with pulmonary overcirculation. Typically, this physiology becomes clinically apparent over the first few days to weeks of life as the pulmonary vascular resistance decreases. The resistances in the pulmonary and systemic vascular beds determine the relative proportion of systemic and pulmonary blood flow. A widening pulse pressure accompanies the worsening pulmonary overcirculation as the pulmonary vascular resistance decreases, and the diastolic run‐off into the pulmonary arteries results in reduction of diastolic arterial pressure. The oxygen saturation in patients with truncus arteriosus depends on the amount of pulmonary blood flow. Because the pulmonary blood flow is generally excessive, most infants with truncus arteriosus have only mildly reduced systemic oxygen saturation (typically ≥90%). In addition, the phenomenon of “streaming” can occur when there is a favorable spatial orientation of the branch pulmonary arteries relative to the VSD. This separates the oxygenated from the deoxygenated blood despite the absence of anatomic aortopulmonary septation and can result in relatively normal oxygenation. If severe congestive heart failure develops, intrapulmonary right‐to‐left shunt due to inefficient gas exchange can lead to later cyanosis. If left unrepaired, increased pulmonary vascular resistance associated with obstructive pulmonary vascular disease may develop [40]. Abnormalities of the truncal valve, either regurgitation or stenosis, may play an important role in the clinical course of infants with truncus arteriosus. In most patients, the stenosis and/or regurgitation are not severe enough to alter the presentation and initial clinical course. If severe truncal stenosis is present, the dominant physiology is that of biventricular outflow obstruction. In patients with moderate or severe truncal valve regurgitation, the combination of left‐to‐right shunt and retrograde flow through the incompetent truncal valve results in severe left ventricular volume overload. In addition, the low diastolic blood pressure may result in compromised coronary perfusion causing myocardial ischemia. The systemic circulation in infants with interrupted aortic arch or severe coarctation depends on flow through a patent ductus arteriosus and, therefore, requires prompt diagnosis and administration of intravenous prostaglandin E1. Closure of the ductus arteriosus will lead to low cardiac output, symptoms of shock, and ultimately death. The management of truncus arteriosus consists of neonatal surgical repair. Improvements in surgical techniques and perioperative management have enabled successful early repair, with a reported mortality of 5–11% [8,35,41–43]. Delayed surgical repair can result in development of pulmonary vascular obstructive disease and myocardial dysfunction. Surgical repair is performed under deep hypothermia with either low‐flow continuous cardiopulmonary bypass or intermittent periods of circulatory arrest [44]. The pulmonary arteries are removed from the truncus, and the resultant opening is either closed primarily or with a patch. The VSD is closed with a patch. Continuity between the right ventricle and the pulmonary arteries is established using a cryopreserved homograft, or a valved heterograft conduit. When significant truncal valve regurgitation is present in infancy, some authors now advocate repair of the truncal valve, however valve stenosis may improve without intervention because of decreased flow after repair [43–45]. Replacement of the truncal valve at the time of initial repair is done only in the most severe cases and is considered high risk [43,46]. Associated anomalies that have been identified as independent risk factors for surgical mortality include interrupted aortic arch, coronary artery anomalies, and truncal valve pathology necessitating truncal valve surgery at the time of initial repair [41,42,47,48]. Following repair, progressive obstruction of the right ventricle‐to‐pulmonary artery conduit requiring replacement over time is expected and progression of truncal valve dysfunction is common [49–56]. Truncus arteriosus can be accurately diagnosed using 2D and Doppler echocardiography [57–60]. In most cases, echocardiography is sufficient to provide a complete preoperative assessment. In assessing truncus arteriosus by echocardiography, it is crucial to have a clear understanding of the data required from the study, but also the limitations of this diagnostic modality so that alternative testing can be employed when needed. The echocardiographic examination should be systematic and organized so that details are not missed. The subxiphoid view provides an image of all aspects of the anatomy and is the preferred initial imaging approach in many centers. In the parasternal long‐axis view, the presence of an overriding single semilunar valve, often with thickened leaflets, and a malalignment‐type VSD provides a clue to the diagnosis of truncus arteriosus (Figure 24.3). Often one can also image the pulmonary artery arising from the posterior aspect of the truncus from that view (Figure 24.4). The truncal valve is well seen, and truncal regurgitation can be assessed by color Doppler. In many instances truncal stenosis can be suggested by the anatomic appearance of the valve. When there is truncal stenosis, the valve appears thickened and leaflet excursion is reduced. This view is inadequate for assessing the severity of truncal stenosis, because the Doppler cursor cannot be aligned parallel to the direction of flow across the valve. If there is an associated interruption of the aortic arch, the ascending aorta can be seen arising from the rightward aspect of the truncus (Video 24.10). Figure 24.3 Parasternal long‐axis imaging plane demonstrating truncal override of the ventricular septal defect (VSD). LA, left atrium; LV, left ventricle; TR, truncal root. From the parasternal short‐axis view with the transducer angled superiorly above the level of the truncal valve, the origin of the pulmonary arteries from the left posterior aspect of the truncus can be readily seen (Figure 24.5). This view is excellent for ascertaining the type of truncus by imaging the branch pulmonary arteries. This view is also ideally suited for the assessment of truncal valve morphology, including its commissures and leaflets. 3D echocardiography may be particularly helpful for assessment of truncal valve anatomy. At the level of the truncal valve, the location of the VSD can be determined. The VSD is within the “Y” of septal band. In some cases, a rim of muscle appears adjacent to the tricuspid valve in the short‐axis view. If the VSD extends to the tricuspid valve (tricuspid‐to‐truncal valve fibrous continuity), it involves the membranous septum. The coronary arteries may be imaged in this view by rotating the transducer clockwise. To image the relationship of the coronary artery to the pulmonary artery, one must angle the transducer superiorly and inferiorly such that the coronary artery and the pulmonary artery are imaged in the same plane. The parasternal long‐axis view and the subxiphoid coronal view are also helpful in imaging the relationship of the coronary artery to the pulmonary artery, as they can often be seen in the same plane. Figure 24.4 Parasternal long axis‐axis view showing the truncal valve overriding the ventricular septal defect (VSD), with the main pulmonary artery (MPA) arising from the posterior aspect of the truncal root (TR). LA, left atrium; LV, left ventricle; TR, truncal root. Figure 24.5 Parasternal short‐axis view demonstrating separate origins of the pulmonary artery branches from the posterior aspect of the truncal root (TR) (truncus arteriosus type 2). LPA, left pulmonary artery; RPA, right pulmonary artery. Figure 24.6 Apical four‐chamber view swept anteriorly to show the truncal valve (TrV) (overriding the right ventricle). There is a broad jet of truncal regurgitation noted emanating from the TrV and extending deep into the right ventricle. AO, aorta; MPA, main pulmonary artery. Parasternal as well as suprasternal views allow assessment for the presence of a patent ductus arteriosus. Although typically a patent ductus arteriosus is only seen in the presence of aortic arch hypoplasia/interruption, rarely it may occur in those with a normal aortic arch [61]. The parasternal long‐axis and apical four‐chamber views are most helpful in identifying additional muscular VSDs through the use of color Doppler. The typical large VSD is seen when the transducer is angled anteriorly toward the outflow tract (apical five‐chamber view), and the degree of truncal override can be assessed. This view is also particularly helpful for assessing truncal regurgitation and stenosis (Figure 24.6). The Doppler beam can be aligned parallel to the flow from this view, and an accurate Doppler gradient can be measured when truncal stenosis is present. The relationship between the pulmonary artery origin and the truncal root can be clearly imaged by angling anteriorly (Figure 24.7, Video 24.11). The subxiphoid view provides details of all aspects of the anatomy. At the atrial level, the atrial septum can be imaged and the pulmonary veins can be seen entering the left atrium. As the transducer is swept superiorly, the truncal valve overriding the VSD can be displayed. In addition, the branch pulmonary arteries and their origin from the truncus can be well seen (Figure 24.8, Video 24.12). The right pulmonary artery can usually be imaged just inferior and posterior to the common trunk. The main pulmonary artery segment and the branch pulmonary arteries can be seen arising from the truncus, and the coronary arteries can sometimes be imaged in this view. In type 1 truncus, the aorta is the main portion of the trunk and thus it is sometimes called “aortic type” (Figure 24.9). In type 4 truncus, the pulmonary artery makes up the main portion of the trunk and thus it is called “pulmonary type” (Figure 24.10). Subxiphoid imaging helps with this distinction. In type 4 truncus, the subxiphoid view provides an image of the small ascending aorta, arising slightly rightward and superior to the truncal valve. If one tilts the transducer superiorly, the bifurcation of the ascending aorta into the innominate and left carotid arteries can often be imaged. Doppler interrogation of the abdominal aorta in the subxphoid long‐axis view is utilized to detect diastolic flow reversal related to run‐off into a low resistance pulmonary vascular bed and/or truncal valve regurgitation. Figure 24.7 Apical four‐chamber view swept anteriorly to show the main pulmonary artery (MPA) segment arising from the left lateral aspect of the truncal root (TR), with subsequent bifurcation into the left and right branch pulmonary arteries. LPA, left pulmonary artery; RA, right atrium; RPA, right pulmonary artery; RV, right ventricle. Figure 24.8 Subxiphoid coronal view swept anteriorly to show the truncal root (TR) and the origin of the main pulmonary artery (MPA) segment from the left lateral aspect of the truncus, with subsequent bifurcation into the right and left pulmonary arteries. LPA, left pulmonary artery; RPA, right pulmonary artery; RA, right atrium; RV, right ventricle. Figure 24.9 Subxiphoid coronal view swept anteriorly to show the larger ascending aorta in the case of a type 1 truncus (“aortic type”). MPA, main pulmonary artery segment. Figure 24.10 Subxiphoid coronal view swept anteriorly to show the larger main pulmonary artery segment in the case of a type 4 truncus (“pulmonary type”). MPA, main pulmonary artery segment. The suprasternal notch view provides the best imaging of the aortic arch and allows for determination of arch sidedness. Long‐axis views can be very helpful in demonstrating aortic arch interruption (Video 24.4). However, careful suprasternal notch short‐axis views are extremely helpful to document truncus with aortic arch interruption (Video 24.13). When the aortic arch is interrupted, the descending aorta is supplied by the ductus arteriosus, which forms a ductal arch. Tilting the transducer to the left of the patient’s sagittal plane can image the ductal arch. The ductal arch can be differentiated from the aortic arch by sweeping to the right and left and imaging the ascending aorta as a separate structure. Doppler interrogation of the descending aorta typically elicits diastolic flow reversal related to flow into the pulmonary arteries and/or presence of truncal valve regurgitation. Many cases of truncus arteriosus are now diagnosed prenatally by fetal echocardiography. The pregnant woman in whom the diagnosis of a fetus with truncus arteriosus is considered may come to attention because of: (i) a family history of congenital heart defect (typically a relative with a conotruncal defect); (ii) abnormal screening amniocentesis or chorionic villus sampling (often a 22q11 microdeletion by FISH); (iii) concern regarding a cardiac defect when the fetus is found on obstetric ultrasound to have extracardiac anomalies (e.g., an abnormality of the palate in the patient with 22q11 deletion); or (iv) the identification of a cardiac anomaly on routine obstetric ultrasound screening. The frequency of prenatal diagnostic screening has increased dramatically over the past two decades, as obstetric ultrasonic cardiac screening techniques have become more standardized [62]. The accuracy of the identification of complex fetal cardiac anatomy has been well established. Tometzki et al. [63] reported their ability to diagnose a constellation of conotruncal defects, including fetuses with truncus arteriosus. Focused studies on the prenatal diagnosis of truncus arteriosus have also been performed, including the assessment of associated cardiac anomalies [64,65]. These studies have shown a high degree of diagnostic accuracy for the overall identification of truncus arteriosus, including identification of VSD position, aortic arch anatomy, truncal valve pathology, and pulmonary artery architecture (Videos 24.14 and 24.15). However, the differentiation between truncus arteriosus (origin of the branch pulmonary arteries from the truncus) and tetralogy of Fallot with pulmonary atresia (no pulmonary arteries arising from the ascending aorta) continues to be challenging in the fetus. In addition, there are well‐recognized variables in the accurate definition of fetal cardiac anatomy, including operator experience, fetal position, maternal body habitus, and gestational age. Nevertheless, the ability to provide accurate prenatal information to families and caregivers has greatly enhanced their options to make timely management decisions. The role of evolving technologies such as “real‐time” 3D fetal echocardiography [66,67] and fetal cardiac magnetic resonance imaging (MRI) [68] awaits further technological refinements and clinical validation. The data regarding postnatal outcomes of prenatally diagnosed truncus arteriosus has grown. Duke and colleagues from the UK [64] reported that of 17 prenatal cases, pregnancy was terminated in four (24%), and eight of the remaining 13 live births underwent neonatal surgery. Two newborns with severe truncal valve stenosis suffered preoperative sudden death. From this subset, 30‐day surgical mortality was 25%. More recent studies of outcomes following prenatal diagnosis have shown a lower early postoperative mortality of 10% [69]. Echocardiographic imaging in the adult with repaired truncus arteriosus contains the essential components of the initial study with additional emphasis on evaluation of the truncal valve and right ventricle‐to‐pulmonary artery conduit. 3D echocardiography can be especially helpful in evaluating the truncal valve (Figure 24.11). By adulthood there will be many patients who have developed severe truncal valve dysfunction such that valve replacement is required. The adult with progressive truncal valve disease is assessed in a manner similar to those with isolated aortic valve pathology. This involves evaluation of the severity of truncal regurgitation based upon color Doppler imaging, extent of diastolic flow reversal in the descending aorta, pressure half‐time of the regurgitant jet, and left ventricular dilation and function. The assessment of truncal valve stenosis is similar to that of aortic valve stenosis, including Doppler assessment of peak and mean gradient, valve morphology, and left ventricular hypertrophy and function. Truncal root dilation is common and can be followed in serial echocardiograms. Criteria for root replacement are similar to other conotruncal anomalies such as tetralogy of Fallot or transposition of the great arteries. Right ventricular dysfunction, particularly in the setting of conduit dysfunction, is also an important component of the echocardiographic assessment and is similar to what is performed in adult patients with tetralogy of Fallot. Right ventricular pressure estimate by tricuspid regurgitation jet is helpful to assess the severity of conduit stenosis. Those with pressure at three‐quarters systemic or greater, generally require reintervention by catheter‐directed therapy or surgical replacement. Figure 24.11 Three‐dimensional echocardiography showing an en face view of a quadricuspid truncal valve with reduced central coaptation (viewed from the truncal root perspective). Miniaturization of transesophageal echocardiographic (TEE) probes has allowed intraoperative and postoperative assessment of truncus arteriosus repair before and after cardiopulmonary bypass [70]. In this way, undetected anomalies may be clarified prior to cardiopulmonary bypass, and the adequacy of repair can be assessed for such lesions as residual VSDs, obstruction across the right ventricle‐to‐pulmonary artery conduit, and competency of the truncal valve (Figure 24.12, Video 24.16) [71]. Rarely, the pathway from the left ventricle to the truncal valve may be narrowed, particularly in those with a trunk that sits entirely over the right ventricle or subtruncal infundibulum. As with other cardiac defects, TEE allows postoperative decision‐making to be made in a timely fashion so that the need for further interventions can be determined in the operating room. Epicardial imaging can also be useful particularly for evaluation of the branch pulmonary arteries after conduit placement as they can be difficult to visualize with standard TEE views. Figure 24.12 Postoperative transesophageal echocardiography in the sagittal plane showing a bicuspid truncal valve with poor coaptation and mild insufficiency. The echo‐bright ventricular septal defect (VSD) patch is visualized. There are instances in which additional diagnostic evaluation is necessary. As acoustic windows become restricted in older children and adults with repaired truncus arteriosus, cardiovascular MRI is the ideal alternative to echocardiography. This modality allows comprehensive anatomic and functional assessments, including accurate quantification of truncal valve regurgitation and biventricular size and function as well as anatomic evaluation of the pulmonary arteries and aorta [72]. Computed tomography (CT) can be used as an alternative modality in patients with contraindications to MRI (e.g., pacemaker or implanted defibrillator). However, physicians recommending CT should carefully consider the risk of cancer due to ionizing radiation, which is considerably higher in young children [73]. That risk notwithstanding, CT can also be used in unrepaired neonates with particularly complex cardiovascular malformations, such as truncus arteriosus with interrupted aortic arch or concern for vascular ring (Figure 24.13, Video 24.17). In selected patients in whom conduit obstruction may be alleviated by transcatheter balloon angioplasty or stent placement, cardiac catheterization may be indicated for hemodynamic assessment and for possible intervention. Although there have been significant trends toward improved survival in the current era, ongoing morbidity related to conduit obstruction and/or regurgitation, truncal valve abnormalities, aortic root dilation, and left and right ventricular dysfunction continue to dominate long‐term outcomes. Interval echocardiographic imaging allows timely detection of progressive pathology prior to irreversible ventricular dysfunction, development of a life‐threatening complication, or loss of opportunity to intervene via nonsurgical routes. As noted above, judicious use of other diagnostic modalities is particularly important during follow‐up of patients with repaired truncus arteriosus. Figure 24.13 Three‐dimensional volume reconstruction of computed tomography angiogram in a patient with truncus arteriosus and interrupted aortic arch (IAA) type A. View of carotid and subclavian arteries arising from the ascending aorta, with pulmonary artery branches slightly offset in position as they arise from the truncal root. DAo, descending aorta; LPA, left pulmonary artery; RPA, right pulmonary artery. Figure 24.14 Apical four‐chamber view swept anteriorly to show the aortopulmonary window (APW) located proximally between the main pulmonary artery (MPA) and the aorta (type I). AO, aorta; LPA, left pulmonary artery; RPA, right pulmonary artery. An aortopulmonary window is a defect between the ascending aorta and the pulmonary artery above the semilunar valves. It is an extremely rare cardiac malformation accounting for only 0.1–0.2% of congenital heart defects [74,75]. Even though it can occur in isolation, more than half the patients have other associated cardiac anomalies [76]. These include type A interrupted aortic arch, right aortic arch, aortic origin of right pulmonary artery, anomalous origin of one or both coronary arteries from the pulmonary artery, tetralogy of Fallot, transposition of the great arteries, and VSD. Based on a classification proposed by Mori et al. [77] there are three types of aortopulmonary defects: Figure 24.15 High parasternal short‐axis showing a type II (distal) aortopulmonary window (APW). Note the aortic origin of the right pulmonary artery (RPA). AO, aorta; LPA, left pulmonary artery; MPA, main pulmonary artery. All three types of aortopulmonary windows typically present with symptoms of a large left‐to‐right shunt due to the unimpeded flow from the ascending aorta into the pulmonary arteries. Less commonly, the communication may be smaller and pressure‐restrictive, resulting in a lesser degree of left‐to‐right shunting and pulmonary overcirculation. Management may initially consist of pharmacologic therapy aimed at decreasing the pulmonary overcirculation. However, definitive therapy is provided by closure of the defect, either surgically or using a transcatheter device. In general, large defects and those associated with other cardiac malformations are closed surgically using a patch. Isolated small aortopulmonary windows or residual defects can be closed using transcatheter devices [78,79]. In some cases where the aortopulmonary window is missed or there is delay in diagnosis, pulmonary vascular disease develops because of the unprotected pulmonary blood flow and can become irreversible.
CHAPTER 24
Truncus Arteriosus and Aortopulmonary Window
Truncus arteriosus
Definition
Incidence
Etiology
Morphology and classification
Developmental considerations
Classification
Morphology
Ventricular septal defect
Truncal valve
Pulmonary arteries
Coronary arteries
Associated cardiac lesions
Pathophysiology
Management
Imaging
Imaging of truncus arteriosus
Prenatal assessment
Imaging of the adult
Intraoperative assessment
Ancillary imaging modalities
Follow‐up assessment
Aortopulmonary window
Definition, incidence, and morphology
Pathophysiology
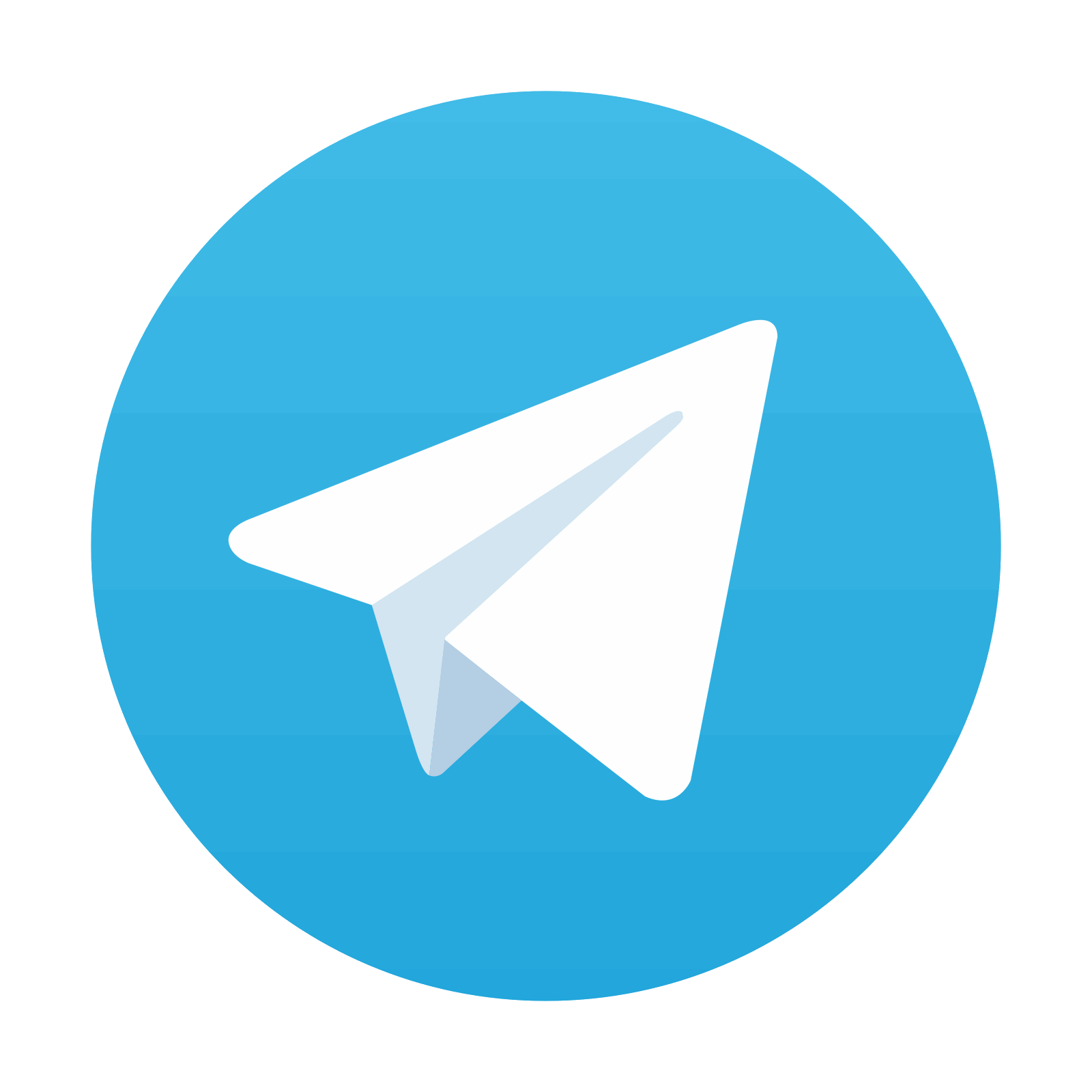
Stay updated, free articles. Join our Telegram channel

Full access? Get Clinical Tree
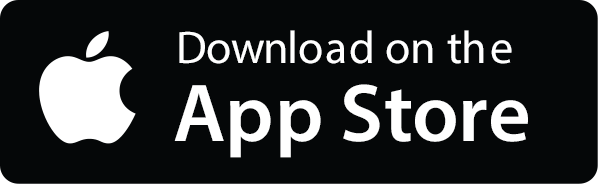
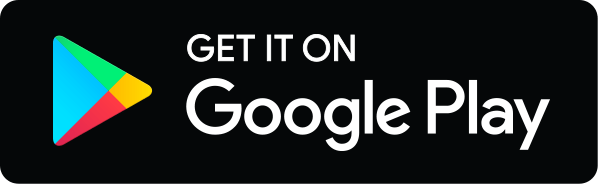