Jan Marek, Matthew Fenton, and Sachin Khambadkone Great Ormond Street Hospital for Children; University College London, London, UK Coarctation of the aorta (COA) refers to narrowing of the aortic isthmus. Hypoplasia of the aortic arch and other associated cardiovascular anomalies are common. The term is also used to describe strictures of other segments of the thoracic or the abdominal aorta. The incidence of COA is approximately 36 (range 29–49) per 100,000 live births, representing the seventh most common type of congenital heart disease, or approximately 5–7% of patients with congenital heart disease [1,2]. In ~64% of patients, COA presents soon after birth, whereas in the remaining 36% it presents at an older age. COA is 1.3–1.7 times more likely in males [3,4]. In a prenatal series, COA constituted up to 10% of all fetal diagnoses of congenital heart disease [5]. No single etiologic cause has emerged for COA but evidence points to an interplay between genetic, environmental, and hemodynamic factors [4–8]. Abnormal flow distribution during fetal life with decreased aortic flow has long been suspected. This is supported by the observation that 71% of cases of aortic atresia also have COA [6]. The role of genetic factors is increasingly recognized, and more recently variations in the NOTCH1 gene have been associated with COA [7]. Particular syndromes have been associated with COA; it occurs in 12% of patients with Turner syndrome (45,XO). The incidence of chromosome 22q11 microdeletion is increased in patients with interruption of the aortic arch [8,9]. COA is often attributed to the presence of ductal tissue in the aortic wall which constricts the isthmus during closure of the ductus arteriosus after birth. The aortic arch is divided into three segments: (i) the proximal arch, between the brachiocephalic (innominate) artery and the left common carotid artery; (ii) the distal arch, between the left common carotid artery and the left subclavian artery (known as the transverse arch); and (iii) the isthmus, between the left subclavian artery and the ligamentum or ductus arteriosus (Figure 22.1). The anatomic criteria for defining arch hypoplasia are: (i) a proximal aortic arch that measures 60% of the ascending aortic diameter; (ii) a distal aortic arch that measures 50% of the ascending aortic diameter; or (iii) isthmus narrowing of 40% of the descending aorta. The commonly used echocardiographic definition determining transverse aortic arch hypoplasia in neonates is an internal diameter <1 mm for every 1 kg body weight [10]. Another commonly used criterion is diameter z‐score less than −2 standard deviations of the normal population’s mean. COA usually results from narrowing of the aortic isthmus at its junction with the proximal descending aorta where the ductus arteriosus inserts into the arch (juxtaductal coarctation). The anatomic spectrum is wide but different morphologic patterns can be distinguished based on age at diagnosis – before birth, early infancy, childhood, or adulthood. In the fetus and infant, the distal transverse aortic arch between the left common carotid artery and left subclavian artery is often elongated and hypoplastic (tubular hypoplasia), the angle between the ascending aorta and transverse arch is acute, the aortic isthmus is often diffusely hypoplastic [5], and the ductus arteriosus is almost always widely patent. In neonates, gradual development of COA is reported during closure of the ductus arteriosus and histologic studies have demonstrated ductal tissue circumferentially surrounding the juxtaductal portion of the aorta [11]. Although the stenotic site is typically located at the distal isthmus, other locations or multiple sites can be found (e.g., adjacent or proximal to the origin of the left subclavian artery). In older children and adults, aortic arch hypoplasia is less common, the COA segment is usually discrete, and collateral arteries bypassing the COA are common. These collateral vessels develop from increased flow through the internal mammary, intercostal, and scapular arteries. Regardless of age at diagnosis, the COA segment is characterized by luminal narrowing due to thickening of the intima and media layers, hypoplasia, and tortuosity of the vessel. The endoluminal protrusion of the intimal–medial ridge (or “shelf”) is typically circumferential but is often more prominent along the posterior and lateral wall of the aortic isthmus. The length of the stenotic segment varies from discrete (2–3 mm) to long segment (7–20 mm). The proximal descending aorta immediately past the COA often exhibits post‐stenotic dilation, a feature more commonly found in older children and adults. Figure 22.1 Schematic drawing of individual aortic arch segments in relationship to the ductus arteriosus or ligament (asterisk). AO, aorta; LCCA, left common carotid artery; LPA, left pulmonary artery; LSA, left subclavian artery; PA, pulmonary artery; RPA, right pulmonary artery; RCCA, right common carotid artery; RSA, right subclavian artery. A high incidence (>50%) of associated cardiac abnormalities suggests that COA is a more complex defect than isolated narrowing of the aorta. COA is commonly associated with bicommissural aortic valve, ventricular septal defect (VSD), subvalvar and valvar aortic stenosis, and common atrioventricular canal. It can be seen in other more complex cardiac defects particularly when the aortic outflow is narrowed. COA is particularly common in patients with multiple left heart obstructive lesions and is part of the Shone complex. Rarely, COA affects other segments of the aorta. The morphology and pathophysiology of abdominal COA is different to the typical COA of the aortic isthmus in that the involved segment is often long, the aortic media is markedly thickened, and involvement of the renal and mesenteric vessels is common. Involvement of the thoracic and abdominal aorta and branches has been reported in Takayasu aortitis, supravalvar aortic stenosis (Williams syndrome, familial aortic stenosis, and sporadic supravalvar aortic stenosis), and midaortic syndrome. “Pseudo‐coarctation” of the aorta refers to deformity of the aortic isthmus characterized by tortuosity but without luminal narrowing. Internally, the caliber of the isthmus is not narrowed and blood flow is not obstructed. The hemodynamic consequences of COA are usually tolerated well in the fetus because blood flow to the lower body and the placenta is supplied predominantly through the ductus arteriosus with only ~10% of the combined cardiac output crossing the aortic isthmus. Narrowing of the aortic isthmus results in diversion of blood flow from the pulmonary artery to the descending aorta by way of the ductus arteriosus. As a result, left ventricular afterload increases and its output decreases, whereas right ventricular output increases. Consequently, the right ventricle dilates while the left ventricle is pressure loaded [12], resulting in the ventricular size disproportion. The pathophysiology of COA in the neonate depends on a complex interplay between the severity of aortic narrowing, the rate at which the COA progresses, patency of the ductus arteriosus, pulmonary vascular resistance, and associated cardiac anomalies. The hemodynamic burden imposed by severe COA manifests after birth as the ductus arteriosus constricts and ultimately closes. As a result, the left ventricle supplies the entire cardiac output and flow to the lower body must cross the narrow aortic segment(s). Under these conditions, the left ventricle faces pressure overload and systolic blood pressure in the upper body is increased. In contrast, distal to the COA the systolic blood pressure is low, manifesting as reduced pulse amplitude in the femoral arteries. When COA occurs proximal to the left subclavian artery, decreased pulse amplitude is noted in the left arm as well. In the rare circumstance of COA proximal to the left subclavian artery associated with aberrant origin of the right subclavian artery, blood pressures and pulse volumes in the arms are similar to those in the lower extremities. In neonates with severe COA, the increased impedance to left ventricular emptying leads to elevated end‐diastolic pressure and left atrial, pulmonary venous, and pulmonary arterial hypertension. A widely patent ductus arteriosus allows right‐to‐left systolic flow from the main pulmonary artery to the descending aorta, providing adequate perfusion to the lower body and normal volume of the femoral pulse. In the absence of an atrial level right‐to‐left shunt or associated cardiac lesions, oxygen saturation in the upper extremities (preductal) is higher than that in the lower extremities (postductal), a phenomenon called differential cyanosis. Constriction and ultimate closure of the ductus arteriosus in neonates with severe or rapidly progressing COA lead to left ventricular dysfunction. Increased afterload due to the COA, decreased preload secondary to increased pulmonary vascular resistance, and and decreased myocardial perfusion contribute to left ventricular dysfunction. Failure of compensatory mechanisms ultimately leads to decreased cardiac output, lower body hypoperfusion, oliguria, metabolic acidosis, and shock with ultimate cardiovascular collapse. If the COA develops slowly and pulmonary vascular resistance normalizes, the left ventricular myocardium hypertrophies in response to the pressure load, wall stress is maintained, and systolic function is preserved. Later in life, COA is usually diagnosed either due to a heart murmur, low pulse amplitude in the lower extremities, claudication, or recognition of systemic hypertension in the upper extremity. The clinical course of this type of COA is often insidious because of the development of collateral vessels between the high‐pressure aortic branches proximal to the COA and the low‐pressure distal aorta. When the collateral vessels are extensive, the pulse volume and blood pressure in the lower extremities can be near normal. Left ventricular pressure overload is gradual in onset and myocardial failure usually develops over decades in response to systemic hypertension and coronary artery disease. Adults with COA can also develop cerebral aneurysms and stroke [13]. Echocardiography provides adequate diagnostic information in most newborns, infants, and young children. However, older children and adults often require other diagnostic modalities (e.g., magnetic resonance imaging (MRI) or computed tomography (CT)), because echocardiographic imaging of the aortic isthmus and proximal descending aorta may be difficult due to increased distance from the transducer and intervening airways. It is imperative that comprehensive evaluation of the aortic arch, arch branches, isthmus, and descending aorta is performed before selecting a management strategy. 2D echocardiographic imaging as well as color and spectral Doppler techniques are used from suprasternal and right and left infraclavicular approaches to evaluate the anatomy and to quantify the degree of obstruction. The entire aortic arch, isthmus, ductus arteriosus, and descending aorta are imaged from the suprasternal notch and from the right and left infraclavicular windows. Extension of the neck and upper thorax by placing a rolled towel or cushion under the shoulders facilitates imaging. Because the distance between the transducer and the aorta is short, these authors recommend using probes with frequencies ranging from 7.5 to 12 MHz to provide high‐resolution imaging. Imaging is first performed in the transverse plane, following the ascending aorta cranially to demonstrate the arch branching pattern, and to image the transverse arch. This view is essential to characterize the anatomy and sidedness of the aortic arch and to exclude associated abnormalities such as right or double aortic arch and/or aberrant origin of a subclavian artery (Figure 22.2, Video 22.1). Long sweeps from the suprasternal notch with a rotating probe clockwise from 1 o’clock to 5 o’clock may offer a comprehensive image of the aortic arch with the COA shelf and the branching pattern (Video 22.2). The oblique sagittal plane with appropriate rotation of the probe is then used to assess aortic arch sidedness and to visualize the long axis of the ascending aorta, arch, isthmus, and proximal descending aorta (Figure 22.3, Video 22.3). In neonates, the right infraclavicular area is particularly helpful for depicting most of the thoracic aorta in one plane (Figure 22.4, Video 22.4). The distal aortic arch (between the left common carotid and left subclavian arteries) is often elongated and hypoplastic. The hypoplasia may extend from the origin of the brachiocephalic trunk or the left common carotid artery up to the site of COA (Figure 22.5, Video 22.5). A protruding posterior ridge is typically present at the site of COA but additional sites of obstruction can coexist due to the presence of another ridge, hypoplasia of the isthmus, or both (Figure 22.6, Video 22.6). Imaging of the COA site at the junction of the isthmus and proximal descending aorta can also be accomplished from the left subclavicular area in a sagittal plane. This view demonstrates the anatomy of the COA in detail and complements imaging from the right subclavicular area. The site of maximal narrowing is determined by 2D imaging and color flow mapping and the diameter of the narrowest aortic segment is then measured on a 2D image (Figure 22.7). Using body surface area‐adjusted normal values (see https://parameterz.blogspot.com/), the z‐score of the narrowest arch segment is calculated. The arch is defined as hypoplastic if its diameter z‐score is less than −2.0. When assessing flow through the aortic isthmus, the suprasternal or right subclavicular windows usually provide an optimal angle for Doppler interrogation. The sample volume is placed at the site of maximum narrowing to avoid overlap with ductal flow. Flow across the isthmus is forward and continuous throughout the cardiac cycle, producing the characteristic appearance of diastolic run‐off. When the ductus arteriosus is restrictive or closed and left ventricular systolic function is preserved, the peak instantaneous pressure gradient across the COA is typically high. The Doppler flow tracing shows a characteristic “serrated” pattern with rapid early acceleration and high‐velocity systolic peak, followed by gradual deceleration throughout diastole (Figure 22.8). Evaluation of the severity of COA with preserved left ventricular systolic function is based on the peak and not the mean gradient because inclusion of the diastolic phase of the antegrade flow would not accurately reflect the systolic gradient. When multiple obstructions are present, usually in the context of associated aortic arch hypoplasia, the “corrected” COA gradient should be measured if the pre‐COA velocity is greater than 1 m/s and is calculated as: 4(V2distal aorta – V2proximal aorta) (Figure 22.9). Figure 22.2 Unusual aortic coarctation in the presence of a right aortic arch with aberrant left subclavian artery. (a) Discrete narrowing (asterisk) seen between the right common carotid artery (RCCA) and right subclavian artery (RSA). (b) The first nonbifurcating neck artery courses to the left suggesting the presence of an aberrant left subclavian artery. DAO, descending aorta; LCCA, left common carotid artery; LPA, left pulmonary artery; RPA, right pulmonary artery. Figure 22.3 Two‐dimensional imaging of neonatal coarctation of the aorta (COA) from the suprasternal notch view. The COA ridge is located ~5 mm below the origin of left subclavian artery. The descending thoracic aorta (DAO) below the obstruction is dilated. AOA, aortic arch. Echocardiographic assessment in evolving or mild COA may differ from the more severe forms (Figure 22.10, Video 22.7). Instead of a typical ridge, 2D imaging demonstrates only mild isthmic narrowing. The Doppler signal in this situation shows only systolic acceleration rather than a typical “serrated” flow pattern. Imaging of the ductus arteriosus is performed from the high left parasternal or so‐called “ductal” view, a position that provides an optimal angle to assess flow. When COA is severe, flow through the ductus arteriosus is typically bidirectional – from the main pulmonary artery to the descending aorta during systole and from the aorta to the pulmonary artery during diastole (because the pulmonary vascular resistance is lower than the systemic vascular resistance) (Figure 22.11, Video 22.8). In patients without critical COA, flow may be from the aorta to the main pulmonary artery throughout the cardiac cycle. Figure 22.4 Neonatal severe juxtaductal coarctation of the aorta (COA). (a) Left infraclavicular view in color compare mode. (b) A patent ductus arteriosus (PDA) with right‐to‐left shunt is demonstrated on color Doppler flow mapping. The COA is readily seen. AO, aorta; DAO, descending aorta; LA, left atrium; LAA, left atrial appendage; PA, pulmonary artery. Figure 22.5 Neonatal coarctation of the aorta (COA) with severe transverse aortic arch hypoplasia seen from the suprasternal view. An elongated, hypoplastic arch can be seen with marked narrowing between the left common carotid artery (LCCA), left vertebral artery (LVA), and left subclavian artery (LSA), which is predominantly supplied by the ductus arteriosus (asterisk). AOA, aortic arch; BCT, brachiocephalic trunk, DAO, descending aorta. Figure 22.6(a) Imaging of neonatal coarctation from the suprasternal notch view in color compare mode. The long proximal transverse arch with short isthmus and coarctation ridge (yellow arrow) is located just below the origin of left subclavian artery (LSA). The descending thoracic aorta (DAO) below the obstruction is dilated. (b) Color aliasing indicates tight isthmus narrowing. AOA, aortic arch; LCA, left common carotid artery; INV, innominate vein. Figure 22.7 Measurements of the aortic arch performed on a 2D image taken from the suprasternal notch. The optimal position of the pulsed‐wave Doppler sample is in the middle of the ductus arteriosus. Repeat echocardiographic examination should be performed shortly after beginning prostaglandin administration to confirm ductal patency or enlargement if there is constriction (Figure 22.12). In newborn babies, evolving COA should be suspected if systolic right‐to‐left shunting is detected in the ductus arteriosus on Doppler interrogation in association with a mildly narrow isthmic area even in the absence of a visible COA shelf (Figure 22.13, Videos 22.7 and 22.9). Reducing the dose of prostaglandin with subsequent ductal restriction may unmask a COA shelf. In some cases, this is required to accurately determine if a COA is present. The vast majority of patients present with a COA shelf located opposite the aortic ampulla of the ductus arteriosus (juxtaductal COA). However, in patients with an elongated isthmus, the COA shelf may obstruct the aorta distal to the ductus arteriosus (postductal COA). In this situation, the ductus arteriosus communicates with the aortic arch proximal to the obstruction resulting in exclusively left‐to‐right shunt throughout cardiac cycle (Figure 22.14, Videos 22.10 and 22.11). Following determination of abdominal situs, cardiac position, and segmental anatomy from the subxiphoid window, the characteristics of blood flow in the descending aorta at or below the level of the diaphragm are evaluated by spectral Doppler. Care is taken to align the ultrasound beam with the long axis of the descending aorta. In neonates with severe COA, and a patent ductus arteriosus, the amplitude of the pulse wave is usually slightly decreased or normal and the diastolic component is absent or slightly reversed. This essentially normal flow profile results from unimpeded systolic flow from the right ventricle to the descending aorta through the ductus arteriosus. The right ventricle generates the pulse wave, and when systolic function is preserved, normal velocity is demonstrated in the aorta below the diaphragm (~20–40 cm/s; no angle correction). In contrast, the flow profile in the abdominal aorta is strikingly different in neonates with severe COA and a closing or a closed ductus arteriosus; it typically exhibits low‐velocity systolic flow with minimal phasic variations and diastolic run‐off (Figure 22.15). In addition to evaluation of the flow profile in the abdominal aorta, the entire aortic arch and the ductus arteriosus can be viewed from the subxiphoid oblique sagittal plane (Video 22.12). Figure 22.8 Coarctation in a small child with a closed ductus arteriosus from the suprasternal sagittal view. (a) In color compare mode, an elongated proximal transverse aortic arch (AOA) with typical juxtaductal posterior shelf (arrow) is demonstrated with (b) color flow turbulence seen at the level of the narrowing. (c) Continuous‐wave Doppler through the aortic isthmus demonstrating high‐velocity systolic amplitude (4.3 m/s) with continuous antegrade flow throughout diastole. DAO, descending aorta. Imaging of the atrial septum from the subxiphoid long‐ and short‐axis views typically demonstrates a patent foramen ovale. The direction of the shunt is depicted by color flow mapping and by spectral Doppler. In neonates with severe pulmonary hypertension and elevated right atrial pressure, flow across the foramen ovale may be bidirectional. In neonates with less severe pulmonary hypertension, flow is predominantly left to right with velocity proportional to the mean pressure gradient between the atria. The apical four‐, five‐, and three‐chamber views are important for assessment of left ventricular inflow and outflow, measurements of left ventricular volume and mass, and for estimation of right ventricular systolic pressure by the tricuspid regurgitation jet velocity. The mitral annulus in isolated neonatal COA may be small; however, mitral valve function is usually normal. Color flow mapping and pulsed‐wave Doppler are used to evaluate the valve with particular attention to restriction of blood flow at the level of the annulus, leaflets, chordae tendineae, or at multiple levels (see Chapter 15 for further details). In an otherwise structurally normal mitral valve and nondilated left ventricle with preserved systolic function, significant mitral regurgitation is uncommon. Figure 22.9 Flow characteristics in the aortic isthmus in a neonatal coarctation. Summation of accelerated forward flow in systole (2.56 m/s) and lower precoarctation velocity systolic flow (1 m/s) is shown. When the proximal velocity is greater than 1 m/s, it should be included in the “corrected” gradient. The low‐velocity diastolic flow (arrow) with respiratory variation through the aortic isthmus is a result of reduction in diastolic blood pressure in the pulmonary artery because of the ductus arteriosus. Figure 22.10 Evolving coarctation (arrows) in a 6‐week‐old infant. (a) 2D imaging in suprasternal sagittal view. (b) Color Doppler flow mapping. (c) Spectral Doppler. Color aliasing indicates flow acceleration across the mild isthmic narrowing in the absence of a ductus arteriosus. Spectral Doppler indicates systolic acceleration (3 m/s) with no persistent diastolic run‐off pattern. AOA, aortic arch; DAO, descending aorta; RPA, right pulmonary artery. Figure 22.11 Ductal flow pattern in severe neonatal coarctation. (a) The sample volume is placed in the middle of the ductus arteriosus. (b) Bidirectional low‐velocity flow with systolic right‐to‐left direction and diastolic left‐to‐right direction typical of critical COA. D, diastole; PDA, patent ductus arteriosus; S, systole. Figure 22.12 Serial echocardiograms in neonatal coarctation in the suprasternal view. (a) Restrictive ductus arteriosus (arrow) demonstrated by color flow mapping prior to prostaglandin administration. (b) Significant ductal widening (arrow) after prostaglandin administration visualized by 2D imaging. AOA, aortic arch; DAO, descending aorta; LCCA, left common carotid artery; LSA, left subclavian artery; PA, pulmonary artery. Evaluation of the left ventricular outflow from the apical window is particularly important due to the common association of COA with subvalvar and/or valvar aortic stenosis. The apical five‐chamber view is used to evaluate the morphology and flow characteristics across the left ventricular outflow tract. Color flow mapping and spectral Doppler are used to determine the presence and severity of obstruction and/or regurgitation (see Chapter 20 for further details). Figure 22.13(a–c) Tubular aortic isthmus hypoplasia (asterisk) in a patient with a large patent ductus arteriosus (PDA). (a) 2D suprasternal view demonstrating no obvious coarctation shelf. (b) Color Doppler demonstrating low‐velocity antegrade flow through the descending thoracic aorta (DAO). (c) Flow velocity in the DAO is only 1.5 m/s. (d–f) Ductal view in the same patient. (d) The PDA is larger than the aortic isthmus (*) from the high parasternal short‐axis view. (e) Color Doppler demonstrating systolic right‐to‐left shunting. The arrow indicates the PDA entry. (f) Pulsed‐wave Doppler demonstrating the unrestrictive right‐to‐left flow in systole (arrow) and left‐to‐right flow in diastole in the PDA. These findings suggest significant coarctation even without obvious findings of a coarctation shelf. AOA, aortic arch; BCA, brachiocephalic artery; LCCA, left common carotid artery; LSA, left subclavian artery; PA, pulmonary artery; RPA, right pulmonary artery. The parasternal long‐ and short‐axis views are used to evaluate left ventricular size and function, mitral valve morphology and function, left ventricular outflow tract, aortic valve morphology, size, and mechanism of stenosis or regurgitation, tricuspid regurgitation, and right ventricular size and function. Coarctation of the aorta in older children and adults is caused by slow progression of the aortic ridge into the lumen of the aortic isthmus (Figure 22.16, Video 22.13). The presence of collateral arteries bypassing the COA is common. Suboptimal acoustic windows and distance from the transducer may hamper imaging of the distal aortic arch, isthmus, and proximal descending aorta (Video 22.14). Adjustment of patient position (e.g., extension of the neck), use of a low‐frequency transducer, and harmonic imaging are tools that can aid in optimizing image quality (Figure 22.17, Video 22.15). In addition, use of the left subclavicular window in the sagittal plane to visualize the aortic isthmus and the descending thoracic aorta is advised. Placing the patient in the left lateral decubitus position aids in obtaining this view. 2D imaging can highlight the “3” sign where the indentation from the shelf occurs (Figure 22.18, Video 22.16). Doppler assessment of COA severity is similar to that in the neonate (see Figure 22.5). Optimal assessment of the velocity profile requires continuous‐wave Doppler with a low‐frequency filter to obtain a clear signal. As in neonatal COA, the gradient is estimated based on peak systolic velocity, not the mean gradient. When multiple obstructions of the aortic arch and isthmus coexist the contribution of each stenosis can be challenging to evaluate. Figure 22.14 Postductal coarctation. (a) Suprasternal view showing the discrete narrowing in the aorta (arrow). (b) Color Doppler demonstrating low‐velocity antegrade flow. (c) Pulsed‐wave Doppler showing estimated maximum velocity of 1.6 m/s. (d) Ductal view demonstrating the coarctation shelf distal to the left subclavian artery (LSA) and to the ductus arteriosus (asterisk). (e) Color Doppler demonstrating all left‐to‐right shunting in the ductus arteriosus in this case. (f) Spectral Doppler of the ductus arteriosus confirms the continuous left‐to‐right flow in systole and diastole. AOA, aortic arch; BCA, brachiocephalic artery; DAO, descending aorta; LCCA, left common carotid artery; PA, pulmonary artery; PDA, patent ductus arteriosus; RPA, right pulmonary artery. The abdominal aorta Doppler flow profile in adult COA is characterized by a low‐velocity systolic signal and diastolic run‐off, which can be visualized by spectral Doppler and by color M‐mode (Figure 22.19). When significant collateral vessels are present the difference between the amplitudes of the systolic and diastolic components of the flow curve is inversely proportional to the size of arterial collaterals. The entire thoracic as well as abdominal aorta should also be imaged to exclude distal COA, which can involve the origins of the celiac, mesenteric, and renal arteries (Figure 22.20, Video 22.17). Similar to neonatal COA, comprehensive echocardiographic evaluation of cardiac anatomy and function is crucial in older children and adults due to the high frequency of associated anomalies. Bicommissural aortic valve and dilation of the ascending aorta are common abnormalities in older children and adults with COA. Coarctation of the aorta diagnosed in the fetus is characterized by narrowing of the aortic isthmus and by frequently associated transverse arch hypoplasia [5]. The main pulmonary artery communicates with the descending aorta through a widely patent ductus arteriosus, creating a large ductal arch. Associated left heart obstructive lesions are also common. Asymmetry of ventricular size (right ventricle larger than left ventricle) demonstrated in the four‐chamber view is frequently the first clue to prenatal diagnosis of COA (Figure 22.21, Video 22.18). The right ventricle is enlarged and tricuspid regurgitation is frequently detected. Right ventricular dilation, however, is not specific for COA and is present in other forms of fetal cardiac defects (e.g, partial or total anomalous pulmonary venous connection, interrupted inferior vena cava) and extracardiac anomalies (e.g., congenital diaphragmatic hernia). Ventricular function is usually normal and unlike other causes of right ventricular enlargement, no other signs of fetal–placental circulatory failure are present and myocardial fibrosis and/or endocardial fibroelastosis is rare. After postnatal COA repair, the left ventricle can remodel and expand to its normal size and volume (Figure 22.22). Despite progress in fetal echocardiography, prenatal diagnosis of COA continues to be challenging. In a recent large nationwide epidemiologic study, only 20% of patients with COA were diagnosed antenatally [14]. In another report, only one‐third of fetuses suspected of having COA were ultimately confirmed after birth [15]. A recent systematic review emphasized the difference in several parameters measured in fetal life. These related particularly to left ventricular inflow and outflow size and the presence of a COA shelf [16]. Figure 22.15 Pulsed‐wave Doppler flow pattern in the abdominal aorta from the subxiphoid sagittal view. (a) Coarctation with restrictive ductus arteriosus and preserved systolic ventricular function in a 5‐day‐old neonate. Systolic waveform amplitude is low; antegrade diastolic run‐off flow indicates the obstruction proximal to sample volume. (b)
CHAPTER 22
Aortic Arch Anomalies: Coarctation of the Aorta and Interrupted Aortic Arch
Coarctation of the aorta
Definition
Incidence
Etiology
Morphology and classification
Pathophysiology
Fetal coarctation
Neonatal coarctation
Adult coarctation
Imaging
Echocardiographic evaluation of neonatal coarctation
Imaging of the aortic arch and isthmus
Subxiphoid views
Apical views
Parasternal views
Adult coarctation
Prenatal assessment
Stay updated, free articles. Join our Telegram channel

Full access? Get Clinical Tree
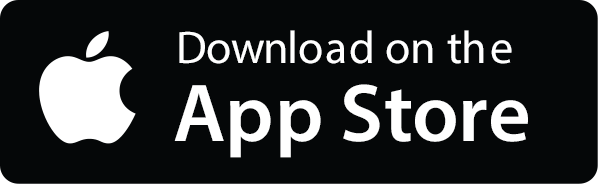
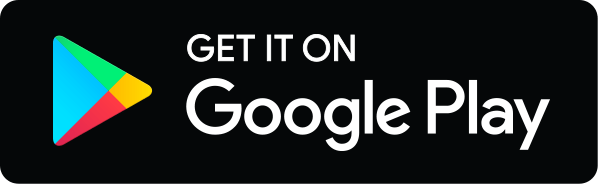