Brian R. White, David J. Goldberg, and Jack Rychik The Children’s Hospital of Philadelphia; Perelman School of Medicine, University of Pennsylvania, Philadelphia, PA, USA Hypoplastic left heart syndrome (HLHS) describes a collection of heterogeneous congenital heart anomalies in which the left ventricle (LV), by virtue of structural abnormalities, is incapable of providing adequate systemic perfusion. HLHS may include abnormalities such as mitral stenosis or atresia, LV hypoplasia, aortic valve stenosis or atresia, and ascending aortic hypoplasia (Figure 21.1, Video 21.1). The syndrome was first reported by Lev in 1952, and the term HLHS was first described by Noonan and Nadas [1,2]. It is one of the most severe forms of congenital anomalies and accounts for significant morbidity and mortality. The epidemiology of HLHS is difficult to determine precisely due to varying definitions. Since Hoffman and Kaplan’s seminal study [3] suggested a birth prevalence of 266 per 1 million live births, subsequent studies have published slightly lower estimates in the range of 100 to 200 per 1 million live births with significant differences in rates between countries [4]. Birth prevalence has been decreasing over time [5], which may relate to improved maternal nutrition as well as higher rates of pregnancy termination. The overall prevalence of HLHS in the population is even more difficult to determine, as large database studies are rare, but is likely around 0.02% [6]. However, with continued improvements in postnatal care leading to improved survival, it is likely that the prevalence of HLHS will continue to increase [7]. Modern genetic testing has highlighted the genetic heterogeneity of HLHS [8]. A particularly important genetic association is Turner syndrome (chromosomes 45,XO), which should be investigated in every female infant with HLHS [9]. Many other syndromes and nonsyndromic genetic mutations have been associated with HLHS, including trisomy 18, 22q11.2 deletion, Jacobsen, Kabuki, Rubinstein–Taybi, Smith–Lemli–Opitz, Adams–Oliver, Beckwith–Wiedemann, Meckel–Gruber, and VACTERL [10]. The American Heart Association (AHA) recommends genetic evaluation for all patients with HLHS and extracardiac malformations [11]. There is a strong familial clustering of HLHS with a recurrence risk of 21% in children of an affected parent and of 8% if a sibling is affected [10]; this association is stronger when extended to other forms of congenital heart disease, especially other left heart obstructive lesions. Perhaps the most significant abnormalities associated with HLHS are neurologic. Patients with HLHS have an increased risk of congenital brain abnormalities such as decreased cortical volumes, agenesis of the corpus callosum, holoprosencephaly, and cortical mantle malformation, as well as an increased risk of developing acquired injury such as hypoxic‐ischemic lesions and intracranial hemorrhage [12]. Patients with HLHS are subsequently at high risk for neurodevelopmental impairment [13]. It is unclear whether these findings are due to underlying genetic factors, abnormalities in cerebral blood flow and oxygenation, or a combination of both [14,15]. Beyond genetic etiologies, some cases of HLHS likely have origins in altered fetal hemodynamics. Specifically, decreased flow through the developing LV due to mitral or aortic valve abnormalities has been implicated in causing progressive LV hypoplasia [16]. This hypothesis has motivated studies of fetal intervention for aortic stenosis [17]. Another hypothesis proposes that abnormalities of the atrial septum limit right‐to‐left flow early in gestation leading to reduced filling of the LV and resultant hypoplasia [18]. Intrinsic abnormalities of LV myocardial development may be another mechanism of HLHS [18]. Hypoplastic left heart syndrome can be roughly divided into the following major anatomic groups based on the size and functionality of the mitral and aortic valves [19]: While some centers have reported that the mitral stenosis and aortic atresia subtype is at an increased risk of mortality [20], other large studies have reported no definitive difference in terms of outcomes [21,22]. However, it is in this group that coronary cameral fistulae are sometimes observed, and some have felt that these fistulae are a risk factor for coronary ischemia and subsequent ventricular dysfunction or acute arrhythmia leading to hypoperfusion and/or sudden cardiac arrest [23]. There is another rare form of HLHS with mitral atresia and aortic stenosis; in all such cases a ventricular septal defect provides flow to the aorta. Unbalanced atrioventricular canal defect with extreme unbalance to the right ventricle can also be considered a variation of HLHS. Measures of systolic function do not seem to be different between subtypes, although the absence of a visible LV has been associated with better diastolic performance [24]. Figure 21.1 Apical four‐chamber view of HLHS. The left‐sided structures are small and incapable of supporting systemic output. LA, left atrium; LV, left ventricle; RA, right atrium; RV, right ventricle. In HLHS the right superior vena cava and the inferior vena cava typically return to the right atrium normally. A review of pathology specimens with HLHS found that 5% also had a left superior vena cava (LSVC) [25]. In the initial surgical management of HLHS, the presence of an LSVC can alter the technique of placement onto cardiopulmonary bypass. In the second stage of palliation, the presence of an LSVC without a left innominate vein requires anastomosis of the LSVC to the left pulmonary artery (a bilateral bidirectional Glenn procedure). In the presence of a large enough bridging innominate vein, the LSVC may be ligated [26]. The right atrium is generally unaffected in HLHS, although it may be dilated to accommodate both systemic and pulmonary blood flows. In patients with HLHS, the left atrium is usually small. However, if there is any restriction to flow across the atrial septum, the left atrium may become hypertensive and distended. Abnormalities of the atrial septum are common in HLHS and may include secundum atrial septal defects or posterior and leftward attachment of septum primum (Figure 21.2). However, it is those patients with an intact atrial septum (approximately 15% of cases [21]) who are at greatest risk of immediate postnatal instability [27,28], although outcomes of those who survive to palliative surgery have improved [21]. Figure 21.2 Subxiphoid left anterior oblique view in color compare mode demonstrating posterior deviation of the septum primum. Note how the right pulmonary veins (RPV) connect normally to the left atrium, but may drain on the right atrial side of the septum primum. Left‐to‐right color flow is seen across the defect. RA, right atrium; SVC, superior vena cava. Deviation of the septum primum can result in anomalous pulmonary venous drainage of the right pulmonary veins to the right atrium without anomalous connection (Figure 21.2). Truly anomalous pulmonary venous connections are present in 5% of patients with HLHS, are a risk factor for poor outcomes, and can complicate staged palliation [21]. The combination of HLHS with total anomalous pulmonary venous connection has a particularly poor prognosis [29]. In the setting of normal pulmonary venous connections with a restrictive atrial septum, a decompressing vein (often termed a “levoatrial cardinal vein”) can allow decompression of the left atrium to the systemic venous circulation and should not be confused with an LSVC [30]. The mitral valve in HLHS may be stenotic or atretic. The presence of a patent mitral valve implies the presence of an associated LV cavity. Such a cavity will be limited in its contribution to systemic perfusion, will typically contract poorly, may have endocardial fibroelastosis, and will exist under high (perhaps suprasystemic) pressure. As mentioned earlier, such differences likely have minimal effect on survival [21] or systolic function [24] unless the LV is very large and non‐functional. In the mitral stenosis and aortic atresia subtype of HLHS, the LV may develop fistulous ventricular–coronary artery connections; although overall such anomalies are not clearly associated with worse outcomes, in severe cases they may have hemodynamic effects [31]. Morphologic and functional abnormalities of the tricuspid valve are common in HLHS. Tricuspid regurgitation (TR) can arise from annular dilation, leaflet dysplasia (Figure 21.3, Videos 21.2 and 21.3), and papillary muscle displacement [32]. The presence of at least moderate TR is a risk factor for poor outcomes [33,34]. In the unoperated, neonatal state, and following initial first‐stage palliation, there is a volume load on the right ventricle (RV), as it supports both systemic and pulmonary blood flows. Such volume load may alter annular dimensions leading to incomplete leaflet coaptation. Although one might expect TR to improve with the reduction of the volume load after superior cavopulmonary anastomosis, recent studies have not found this to be the case [35,36]. However, tricuspid valve repair can be a successful strategy to improve the competency of the valve and is sometimes performed simultaneously with the Glenn procedure [37,38]. While the degree of TR does not consistently improve following valvuloplasty, RV dysfunction may improve after the volume‐unloading procedure (Glenn procedure) [39]. Due to changes in loading conditions, longitudinal comparisons can be difficult, but trends are important as decreasing RV function has been shown to be a risk factor for mortality [40]. In patients who have undergone palliation with an RV‐to‐pulmonary artery (PA) conduit, regional wall motion abnormalities may persist in the area of the ventriculotomy [41]. In HLHS, a patent ductus arteriosus (PDA) is necessary for postnatal survival. In the fetus with HLHS, the PDA is the predominant avenue for systemic flow, and the ductal arch consequently develops with a continuous, smooth transition into the descending aorta. The ascending aorta in HLHS varies in size related to the degree of antegrade flow (Figure 21.4, Videos 21.4 and 21.5). In cases of aortic atresia, the ascending aorta may be markedly hypoplastic and may serve as a “common coronary artery” carrying blood in a retrograde manner to the aortic root. In cases of aortic stenosis, the ascending aorta may be relatively larger depending on the ratio of antegrade flow from the LV to retrograde flow from the PDA. The transverse aortic arch often exhibits varying degrees of hypoplasia and elongation. Interrupted aortic arch has also been reported in HLHS [42]. In such cases, coronary perfusion and survival depend on sufficient antegrade flow across the aortic valve. The descending aorta beyond the ductal arch is generally of normal caliber as it carries the normal systemic output to the lower half of the body. Figure 21.3 A patient with HLHS with an Ebsteinoid tricuspid valve. The valve is demonstrated in systole (a) and diastole (b). The anterior leaflet is elongated and sail‐like. The septal leaflet is apically displaced and tethered. (c) With color Doppler there is moderate tricuspid regurgitation from a broad coaptation defect. LA, left atrium; RA, right atrium; RV, right ventricle. Figure 21.4 Imaging from multiple views demonstrates aortic hypoplasia in HLHS. (a) Parasternal long‐axis view demonstrating a hypoplastic left ventricle (LV), aortic valve, and ascending aorta. This view is useful for determining the diameter of the aorta. (b) Suprasternal long‐axis view demonstrating the ascending aorta and transverse arch hypoplasia (in a different patient). (c) Color Doppler in the same view as (b) showing flow from the ductus arteriosus antegrade to the descending aorta and retrograde through the transverse aortic arch in the setting of aortic atresia. LA, left atrium; RV, right ventricle. The coronary arteries in HLHS typically have normal ostia which arise normally from the aortic root, and follow a normal course [43]. However, cases of anomalous origin of a coronary artery from the main and right pulmonary arteries have been reported [44,45]. As described earlier, in the subset of HLHS characterized by mitral stenosis and aortic atresia, patients may be at risk of fistulous connections, with or without stenoses, from the LV to the coronary arteries. With current obstetric ultrasound screening, HLHS is prenatally diagnosed in more than 60% of patients [46]. Additional cases may be detected with newborn pulse oximetry screening [47]. Even in the absence of screening, infants with HLHS often present within the first 24 hours of life, and all will present within the first weeks of life [48]. Symptoms are mediated by pulmonary overcirculation and cardiovascular collapse from PDA closure. Symptoms include tachypnea and cyanosis. Peripheral pulses may be weak, with cool extremities due to poor perfusion. Chest radiography will usually reveal a large heart with increased pulmonary vascular markings. Once the PDA begins to constrict, blood supply to the body decreases, and an increasing amount of blood is driven into the pulmonary circulation, resulting in improved oxygenation, but at the cost of systemic hypotension and circulatory shock. An open PDA supplies most, if not all, of the blood flow to the lower body. Depending on the anatomic type of HLHS, retrograde flow through the PDA into the aortic arch may also be the major or only source of blood supply to the upper extremities, head, and coronary arteries. Because there is limited or no contribution to systemic blood flow from the LV, flow through the PDA will be from the pulmonary artery to the aorta during systole. During diastole, however, it is the ratio of vascular resistances between the pulmonary and systemic circulations that dictates flow. Following birth, pulmonary vascular resistance is normally lower than systemic vascular resistance, and diastolic flow across the PDA is therefore typically left to right (aorta to pulmonary artery). If the amount of left‐to‐right diastolic shunting is large relative to cardiac output, this can result in a state of systemic hypoperfusion. In conditions in which the pulmonary vascular resistance is abnormally elevated – such as in the presence of a very restrictive or intact atrial septum, lung parenchymal disease, persistent pulmonary hypertension, or lung prematurity – the left‐to‐right shunt in diastole may be minimized or completely eliminated [49]. Such infants will manifest severe cyanosis. When HLHS is identified, prostaglandin E1 infusion should be initiated immediately in order to maintain patency of the PDA. Preoperative management focuses on balancing the systemic and pulmonary blood flow to avoid the complications described. A patient with an intact or restrictive atrial septum may require an urgent intervention to open the atrial septum in order to allow unobstructed pulmonary venous return [50]. Following stabilization, the surgical approach to HLHS is variable. Since Norwood’s initial reports of successful palliation [51], modifications of the technique and associated care have led to substantial improvements in survival. Currently, there are a number of strategies available for neonates with HLHS. Figure 21.5 Diagrammatic representation of first‐stage palliation for HLHS. Both variants involve the creation of a neoaorta from the native aorta, the native pulmonary valve, and patch augmentation. They differ in the method to establish pulmonary blood flow. (a) The Norwood procedure with a modified Blalock–Taussig shunt (BTS) connecting the systemic circulation to the pulmonary circulation. (b) The Sano modification with a right vein‐to‐pulmonary artery conduit in place of the modified BTS. Source: Modified from Gardner TJ, Spray TL (eds) Operative Cardiac Surgery, 5th edn. London: Arnold Publishers, 2004. Primary cardiac transplantation is practiced at a small number of institutions in the United States, with good results [52]. However, limited donor availability has curtailed the widespread use of this practice as a primary means of therapy. As outcomes following staged reconstructive surgery have continued to improve, many centers have reserved primary transplantation for high‐risk infants such as those with dysplasia of the tricuspid valve and severe TR and/or marked RV dysfunction. Primary transplantation is now the management strategy in less than 1% of cases [53]. The most common surgical approach for HLHS continues to be the stage 1 reconstruction, also known as the Norwood procedure [53]. This operation consists of an atrial septectomy, transection of the pulmonary artery (PA) with oversewing of the distal stump, construction of a neoaorta using the incised native aorta augmented with a homograft patch sewn to the native proximal PA, ligation of the PDA, and a method to establish secure pulmonary blood flow [51]. Pulmonary blood flow can be supplied using either a modified Blalock–Taussig (BT) shunt or an RV‐to‐PA conduit, often known as a Sano conduit [54] (Figure 21.5). In high‐volume centers, the outcome for the Norwood procedure can be quite good, with survival rates of 80–90% [21,33,53]. In the Single Ventricle Reconstruction (SVR) Trial, infants were randomized to a BT shunt or a Sano modification. While initial reports demonstrated an early survival advantage for the Sano modification [55], longer term follow‐up data suggest that transplant‐free survival is similar between the two strategies, and patients with RV‐to‐PA conduits require more frequent reinterventions [56]. An alternative strategy that avoids the use of cardiopulmonary bypass and deep hypothermic circulatory arrest in the neonatal period is the so‐called “hybrid” procedure (Figure 21.6). By placing a stent in the PDA and banding the branch pulmonary arteries, it is possible to create a desirable physiologic situation in which the single RV supplies the systemic and pulmonary circulations and in which pulmonary blood flow is limited, protecting the pulmonary vasculature [57]. This approach achieves physiologic goals that are similar to the Norwood procedure [58]. Ultimately, arch reconstruction takes place in conjunction with a superior cavopulmonary anastomosis – a so‐called “comprehensive” stage 2. Determining the relative benefits of the Norwood and hybrid approaches is difficult, as studies are confounded by indication and biased by surgeon preferences. The hybrid procedure likely does not offer a survival advantage over the Norwood operation, except perhaps in the lowest birth‐weight infants [59]. While a few institutions routinely perform hybrids, most centers use the Norwood operation exclusively or reserve the hybrid for patients too unstable to undergo a major neonatal surgery or be safely exposed to cardiopulmonary bypass [60]. Figure 21.6 Diagrammatic representation of the “hybrid” procedure for HLHS. In this procedure, stents are placed across the atrial septum and the ductus arteriosus, and bands are placed across the branch pulmonary arteries. LPA, left pulmonary artery; PDA, patent ductus arteriosus; RPA, right pulmonary artery. Source: Galantowicz M, Cheatham JP. Lessons learned from the development of a new hybrid strategy for the management of hypoplastic left heart syndrome. Pediatr Cardiol 2005;26:190–9. © 2005, Springer Nature. Once HLHS is suspected in the neonate, a detailed echocardiographic evaluation is undertaken, including 2D, color, and spectral Doppler imaging. A segmental approach to analyzing this complex anomaly is performed in a systematic manner by the congenital echocardiographer. The right atrium is well seen from a variety of views. It is usually dilated and hypertrophied due to the volume load from obligate prenatal left‐to‐right shunting. Subxiphoid long‐ and short‐axis imaging delineates the connections of the venae cavae, which are typically normal into the right atrium. A persistent LSVC to the coronary sinus can be reliably identified by echocardiography and should be ruled in or out in all cases of HLHS. The resulting dilation of the coronary sinus can be visualized from the subxiphoid, apical, and parasternal windows, which provides a clue that an LSVC is present (see Chapter 11 on systemic venous anomalies). In the suprasternal view, the LSVC can be directly visualized and should course from superior to inferior, directly anterior to the left pulmonary artery. Doppler interrogation demonstrating a low‐velocity venous flow pattern distinguishes an LSVC from other structures in the general vicinity, such as a branch pulmonary artery or left atrial appendage. Whereas flow through an LSVC is generally directed into the right atrium via the coronary sinus, the presence of retrograde flow in an LSVC should raise suspicion of coronary sinus ostial atresia. If a cephalad direction of blood flow is noted through an LSVC, further investigation of the nature of the coronary venous drainage should be undertaken as any manipulation of the LSVC in this circumstance could deleteriously impact coronary perfusion. An LSVC should be distinguished from other venous structures such as a vein decompressing a hypertensive left atrium, which is a more posterior venous structure. The presence of a decompressing vein can be confirmed using color Doppler from the subxiphoid and suprasternal views, although other views, including “off‐axis” views such as high left parasternal, may also be helpful to trace the entire course and anatomy of the vein. Such decompressing veins usually connect the left upper pulmonary vein to the innominate vein, but other decompressing veins entering the venae cavae or the azygous vein can be found. Obstruction may be seen anywhere along the course of the vein, hence a full view from the left atrium to the insertion into the systemic venous system is important. A decompressing vein can be difficult to distinguish from the pulmonary arteries or anomalous pulmonary veins, so a comprehensive examination using 2D, color Doppler, and spectral Doppler is essential. A rapid and accurate assessment of the atrial septum is crucial for decision‐making about disposition in the immediate postnatal period. The specific anatomy of the atrial septum can be quickly and accurately diagnosed from subxiphoid imaging, particularly from long‐axis, short‐axis, and left anterior oblique views (see Figure 21.2). Acceleration across the atrial septum can be seen using color and spectral Doppler and should be suspected when the left atrium appears distended (Figure 21.7). Mean gradients across the atrial septum can help estimate the severity of atrial septal obstruction. The connections and drainage of all pulmonary veins must be visualized by 2D and color Doppler. Although the pulmonary veins can be seen from subxiphoid imaging, they are more reliably seen from the suprasternal frontal view. A comprehensive examination for anomalous pulmonary venous drainage, including a high right parasternal view, should be undertaken. Anomalous pulmonary veins may complicate later stages of palliation, but become more difficult to identify after the neonatal period. Spectral Doppler is important to assess for pulmonary vein stenosis, which manifests as high‐velocity turbulent flow with diminished or absent phasic variations. Figure 21.7 Restrictive atrial septum in HLHS. (a) Two‐dimensional image from the subxiphoid long‐axis view profiling a 2‐mm atrial communication. Note the distended left atrium (LA) because of the elevated atrial pressure. (b) With color Doppler in the same view, the high‐velocity flow jet from the LA to the right atrium (RA) is profiled. The jet is aliased and splays out on the anterior wall of the RA. (c) Continuous‐wave Doppler interrogation across the atrial communication. The velocity remains elevated throughout the cardiac cycle without the normal return to baseline. The mitral valve is evaluated for patency (stenosis versus atresia) and morphology. The dimensions of the mitral valve are measured in both the apical four‐chamber view (lateral diameter) and the parasternal long‐axis view (anteroposterior diameter). The inflow pattern into the LV and the degree of mitral regurgitation should be documented using color and spectral Doppler. The presence of inflow into the LV without evidence of egress should alert the echocardiographer to the possibility of ventriculocoronary artery connections as a means for decompressing the LV. Color Doppler interrogation of the LV free wall, apex, and septum with a low Nyquist limit should be performed in search of such connections. Examination of the LV itself is best accomplished from the apical four‐chamber view, but imaging from the subxiphoid and parasternal windows is complementary. The presence of an echo‐bright endocardium, which may represent endocardial fibroelastosis, should be noted. Rarely, the LV may contain calcifications, although these are not usually of hemodynamic significance. The tricuspid valve is imaged from multiple views and angles. The subxiphoid right anterior oblique view demonstrates the tricuspid valve inflow and the pulmonary outflow in one plane. The apical four‐chamber and parasternal long‐axis views are optimal for showing the morphology of the tricuspid valve and the subvalvular apparatus. Leaflet displacement and abnormal chordal or papillary attachments can be well seen from the apical view. It is important to identify and to quantify TR. This is best done from apical four‐chamber and parasternal windows using color Doppler interrogation. 3D echocardiography has emerged as a newer tool to evaluate the tricuspid valve morphology and may be particularly helpful in identifying the mechanism of regurgitation when present [61]. The aortic and ductal arches are well seen from suprasternal oblique sagittal imaging (long‐axis view), and the ascending aorta by itself may be seen from the parasternal long‐axis view (see Figure 21.4). The presence or absence of flow across the aortic valve can be seen by color Doppler interrogation in the apical and parasternal views. The full extent of the PDA is best evaluated from a high left parasternal view. In the ideal “ductal view,” the origin of both the right and left pulmonary arteries can be seen along with the insertion of the PDA (Figure 21.8, Video 21.6). With very slight manipulations of the tail of the transducer, it is usually possible to see the full course of the PDA from the pulmonary artery to its insertion into the aorta. In HLHS, the PDA is typically quite large and takes a curved trajectory with a smooth transition into the descending aorta. With the probe in the suprasternal sagittal position, a similar image can be obtained and the ductal and aortic arches can be compared with mild left–right tilting. The aortic arch frequently has a more acute curve than normal and frequently appears as a “branch” off the larger ductal arch. The direction of flow in the PDA is determined with color and spectral Doppler from the suprasternal or high left subclavicular sagittal views. Doppler sampling in the distal aspect of the aortic arch and aortic isthmus reveals the retrograde flow in systole up into the transverse aortic arch, with antegrade forward steal into the PDA and pulmonary vascular bed in diastole (Figure 21.8). Doppler interrogation of the descending aorta distal to the PDA will reveal a flow pattern similar to that seen in the PDA itself with antegrade flow in systole and retrograde flow in diastole. Figure 21.8 Evaluation of the ductus arteriosus from a high left parasternal view. (a) This 2D image delineates the branch pulmonary arteries as well as the course of the patent ductus arteriosus (PDA) from the main pulmonary artery (MPA) to the aorta. (b) Color Doppler flow mapping in systole demonstrating flow from the MPA into both the right and left pulmonary arteries (RPA and LPA) as well as toward the aorta from the PDA. (c) In diastole, flow across the PDA is retrograde from the aorta toward the MPA with run‐off into the branch pulmonary arteries. (d) Spectral Doppler demonstrating right‐to‐left flow during systole and left‐to‐right flow in diastole that is typical of a ductal‐dependent systemic circulation. The laminar pattern indicates no obstruction to flow. The coronary arteries arise from the small aortic root, and typically have normal origins and distributions. These origins are best visualized in the parasternal short‐axis view with focus on the aortic root just above the level of the aortic valve. The right coronary artery may be more prominent than the left as it perfuses the systemic, dominant ventricle. However, if there are significant coronary‐to‐left ventricle fistulous connections, the left coronary artery may appear enlarged. The right and left coronary artery distribution can sometimes be appreciated from a modified apical view angled anteriorly and superiorly toward the outflow tract. Hypoplastic left heart syndrome is readily diagnosed in the fetus and is one of the most frequently detected forms of congenital heart disease prior to birth [46,62]. It can be easily identified by the abnormal chamber morphology at the time of screening prenatal ultrasound (Figure 21.9). A detailed fetal echocardiographic examination delineates the anatomic details of HLHS, including the morphology of the left heart structures and associated cardiovascular anomalies. Because of the association with extracardiac anomalies and various syndromes, it is advisable for all fetuses with HLHS to have careful obstetric ultrasound evaluation for other defects and consideration of testing for genetic/chromosomal abnormalities [63]. Once diagnosed, serial echocardiographic evaluation during gestation is indicated in the fetus with HLHS as complications may develop or progress in later gestation, thereby affecting perinatal management. Blood flow patterns in the fetus with HLHS are different than normal. In HLHS, the pulmonary venous return to the left atrium must traverse the atrial septum and drain to the right atrium. The presence of such a left‐to‐right atrial level shunt is an important parameter, which defines the inadequacy of the left‐sided structures [64]. In addition to the direction of flow, the size of the interatrial communication is important. Although of limited clinical consequence in utero, a restrictive or intact atrial septum complicates the postnatal course. Patency of the interatrial communication is critical for normal lung development as increased left atrial pressure in this setting is transmitted back to the pulmonary vasculature, leading to pulmonary vein dilation and smooth muscle proliferation in the walls of the pulmonary veins [65,66]. Figure 21.9 Four‐chamber fetal echocardiogram of a fetus with HLHS. The small left ventricle (LV) is a typical feature, although the LV may have been normally sized early in gestation. Note the echobrightness of the LV suggesting endocardial fibroelastosis. LA, left atrium; RA, right atrium; RV, right ventricle. The magnitude of atrial level restriction is assessed by direct inspection of the atrial septum on 2D imaging as well as by color Doppler flow mapping. Pulsed Doppler interrogation of the pulmonary veins is important for assessing the degree of atrial restriction and should be performed in every fetus with HLHS (Figure 21.10) [63]. The greater the degree of reversal of pulmonary venous flow with atrial contraction, the greater is the degree of impediment to left atrial egress [67,68
CHAPTER 21
Hypoplastic Left Heart Syndrome
Definition
Prevalence
Etiology and associated anomalies
Morphology and classification
Types
Anatomy
Systemic veins
Atria
Pulmonary veins
Mitral valve and left ventricle
Tricuspid valve and right ventricle
Aorta, coronary arteries, and ductus arteriosus
Presentation and pathophysiology
Management
Imaging
Preoperative assessment
Right atrium and systemic veins
Atrial septum
Pulmonary veins
Mitral valve and left ventricle
Tricuspid valve and right ventricle
Aorta and ductus arteriosus
Coronary arteries
Prenatal assessment
Stay updated, free articles. Join our Telegram channel

Full access? Get Clinical Tree
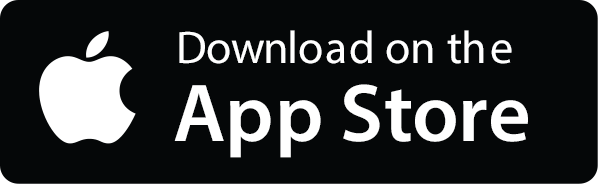
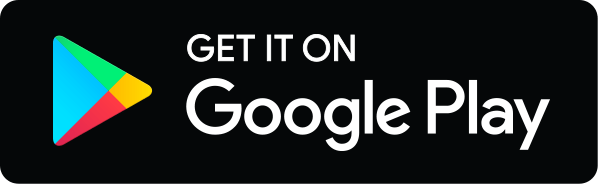