John M. Simpson and Owen I. Miller Evelina London Children’s Hospital, London, UK Obstruction of the left ventricular outflow tract may occur below the level of the aortic valve (subaortic stenosis), at the level of the aortic valve (valvar aortic stenosis), or above the aortic valve, typically at the level of the sinotubular junction (supravalvar aortic stenosis). When there is a ventricular septal defect immediately below the aortic valve there is the potential for one of the aortic cusps (usually the right coronary cusp) to prolapse into the ventricular septal defect, which can lead to regurgitation of the aortic valve. A sinus of Valsalva aneurysm refers to abnormal dilation of the aortic root wall or scalloped area of the cusps of the aortic valve. This condition can be complicated by rupture of the affected sinus into an adjacent area of the heart. Finally, an aortico‐left ventricular tunnel is a lesion where there is abnormal communication between the ascending aorta and the left ventricle through an abnormal pathway (“tunnel”), which has the hemodynamic effect of severe aortic regurgitation. The incidence of aortic valve stenosis in live born infants is around 0.4 per 1000 live [1]. The incidence of bicuspid aortic valve is approximately 13.5 per 1000 births but is likely higher [1]. In a cross‐sectional study, Kitchiner et al. [2] described an incidence of subvalvar aortic stenosis of 0.09 per 1000, whereas that of supravalvar aortic stenosis was 0.05 per 1000. Sinus of Valsalva aneurysm and aortico‐left ventricular tunnel are quite rare lesions without large reported numbers to give an accurate estimation of population incidence. There is good evidence that cardiac defects causing left heart obstruction have a significant genetic component [3,4]. Aortic valve stenosis is seldom associated with major chromosomal abnormalities [5], but empiric studies of familial patterns of recurrence [3] suggest a significant genetic influence. Recent work has implicated the GATA and NOTCH pathways and genes such as KCNJ2 and NKX2‐5 [6–8]. Bicuspid aortic valve has a definite genetic component [9] and may coexist with other forms of left heart obstruction such as coarctation of the aorta. This has led professional groups to recommend family screening where left ventricular outflow tract obstruction, particularly bicuspid aortic valve, has been identified in the index case [10,11]. Supravalvar aortic stenosis is most characteristically associated with Williams–Beuren syndrome, which is due to a deletion in the elastin gene on chromosome 7q11.23 [12]. Familial occurrence of supravalvar aortic stenosis has been observed without the characteristic phenotype or gene deletion found in Williams–Beuren syndrome and may be due to other mutations in the elastin gene [13]. Subaortic stenosis may be familial [14], but in most cases is felt to be the result of the interaction of a number of factors. These factors include abnormal morphologic findings such as ventricular septal defect and abnormal aortoseptal angle producing increased septal shear stress, which in susceptible individuals leads to abnormal cellular proliferation causing subaortic obstruction [15,16].This is supported by recent studies on computational flow dynamics [17]. Subaortic stenosis may occur in isolation or in association with other congenital heart defects such as ventricular septal defect, atrioventricular canal defect, bicuspid (bicommissural) aortic valve, aortic valve stenosis, coarctation of the aorta, interruption of the aortic arch, and double‐chambered right ventricle [18–20]. In addition, subvalvar aortic stenosis may accompany conotruncal anomalies and complex anomalies, including transposition of the great arteries, double‐outlet right ventricle, and single ventricle. Abnormalities of the insertion of the mitral valve or accessory mitral valve tissue may also coexist [21]. Variation in the morphology of the left ventricular outflow tract obstruction can have several causes: Figure 20.1 Transesophageal echocardiography at 148° of a patient with “membranous” subaortic stenosis. There is a discrete area of stenosis below the aortic valve. The aortic valve itself is of normal size. AoV, aortic valve; LV, left ventricle. Figure 20.2 Transesophageal echocardiography at 121° in color compare mode of subaortic stenosis. Color Doppler demonstrates that flow acceleration begins at the level of the subaortic membrane. Ao, aorta; LA, left atrium; LV, left ventricle; Sub AS, subaortic stenosis. Figure 20.3 Transesophageal echocardiogram demonstrating a subaortic membrane immediately below the aortic valve. There is further obstruction of the left ventricular outflow tract due to septal attachments of the left atrioventricular (AV) valve in a patient with a repaired AV canal (AV septal) defect. Ao, aorta; LA, left ventricle; LAVV, left atrioventricular valve; LV, left ventricle; Sub Ao, subaortic. Figure 20.4 Three‐dimensional echocardiogram of the left ventricular outflow tract viewed from the left ventricular aspect. The septal attachment of the left atrioventricular valve (LAVV) chords can be seen as well as a circumferential subaortic membrane (asterisks). RV, right ventricle; RVOT, right ventricular outflow tract. The development of subaortic stenosis is thought to be due to abnormal shear forces in the left ventricular outflow tract, leading to abnormal growth of fibrous tissue [15,17,31]. Such shear forces are increased in the presence of an abnormal aortoseptal angle and other lesions such as a ventricular septal defect. The obstruction to the left ventricular outflow tract leads to compensatory ventricular hypertrophy. Contractile function of the left ventricle is usually well maintained initially but may worsen with time if severe outflow tract obstruction is not relieved. The fibrous tissue below the aortic valve may extend onto the aortic valve leaflets and contribute to obstruction of the left ventricular outflow tract. The turbulent jet across the subaortic region may also interfere with optimal motion of the aortic valve and may contribute to dysfunction of the aortic valve, including aortic valve regurgitation. Consequently, some centers have advocated early surgical resection of the subaortic lesion to reduce the risk of aortic regurgitation [25,26], a strategy that has not been consistently adopted [31,32]. There is a clear indication for surgical intervention where there is severe left ventricular outflow tract obstruction. The indications for intervention in children with relatively mild outflow tract obstruction are less clear‐cut. As a minimum, serial echocardiographic examination is required because rapid progression of subaortic stenosis may occur in infancy and early childhood [26]. For adults with relatively low Doppler‐derived gradients (<50 mmHg), a conservative approach may be adopted although progression may occur during adult life, albeit more slowly than in infancy and early childhood [26,33]. Figure 20.5 Subxiphoid view of an infant with coarctation of the aorta and “tunnel‐type” subaortic stenosis. There is no discrete “membrane” but the subaortic region is diffusely hypoplastic with the potential for a long segment of obstruction to the left ventricular outflow tract. AMVL, anterior mitral valve leaflet; LV, left ventricle; RV, right ventricle. The goals of echocardiographic assessment of subvalvar aortic stenosis are to comprehensively characterize the morphologic features of the obstructive lesion, to evaluate the hemodynamic severity, and to delineate associated anomalies. The assessment of severity of left ventricular outflow tract obstruction can be done in a manner analogous to that of aortic valve stenosis (see “Aortic valve stenosis” later in this chapter). Muscular subaortic obstruction may be accompanied by an increase in the Doppler velocity in late systole leading to an asymmetric Doppler trace consistent with dynamic obstruction. To obtain optimal Doppler signals, imaging is undertaken from the apical and from the suprasternal notch views. PW Doppler is helpful to localize flow acceleration and, in patients with several levels of obstruction, to determine the contribution of each location to the total estimated pressure drop. The maximum instantaneous and mean gradients across the left ventricular outflow are also recorded from both transducer positions by CW Doppler, with particular attention to optimization of the alignment between the ultrasound beam and the direction of flow. A detailed discussion of the principles of assessing stenosis by Doppler can be found in Chapter 6. Imaging of the morphology of the left ventricular outflow tract is crucial for planning management. In the membranous form of subaortic stenosis the full extent of the fibrous membrane should be defined, as such membranes vary in their distance from the aortic valve itself, and some extend circumferentially to involve the anterior leaflet of the mitral valve. This assessment is best achieved from the parasternal long‐ and short‐axis views. 3D imaging allows en face views of the subaortic obstruction from the left ventricular cavity and through the aortic valve (Figure 20.6). In some patients, accessory tissue related to the mitral valve attaches to the subaortic septum, producing or contributing to subaortic stenosis [34]. The aortic valve may be intrinsically abnormal or be restricted in its motion due to encroachment of fibrous tissue onto the ventricular aspect of the leaflets. The aortic valve leaflets may “flutter” during systole as they are hit by the turbulent jet from the subaortic region (Figure 20.7). Comprehensive echocardiographic evaluation is crucial for planning subaortic stenosis surgery. Discrete “membranous” subaortic stenosis may be resected with preservation of the native aortic valve. However, “tunnel” forms of subaortic stenosis may not be able to be managed by resection of a localized area of tissue and may require a more extensive resection [24,28,29,35]. It is therefore essential to have all anatomic information before surgical repair. In the tunnel type of subaortic obstruction, the left ventricular outflow tract, including the aortic valve annulus, is often hypoplastic. In selected patients, extensive subaortic resection may have to be combined with insertion of the patient’s pulmonary valve into the aortic position (Ross–Konno operation) [36,37]. In small infants, transthoracic echocardiographic imaging is usually all that is necessary to plan treatment, but in older children and adults, transesophageal echocardiography (TEE) may be necessary to gain all relevant information. In some patients, subaortic obstruction follows the repair of more complex forms of congenital heart disease [38]. An example of this would be subaortic obstruction following a “Rastelli” type of repair for conditions such as transposition of the great arteries with a ventricular septal defect and pulmonary stenosis. In this repair, the outflow from the left ventricle is baffled to the aorta via the ventricular septal defect, using a patch. The tortuous outflow tract is a substrate for subaortic obstruction (Figure 20.8). Subaortic stenosis may also develop following complete repair of an atrioventricular canal defect which may be related to the unwedged position of the native aorta and elongation of the left ventricular outflow tract (Figure 20.9). Other examples include conditions such as double‐inlet left ventricle with transposition of the great arteries, where the outlet of the left ventricle to the ascending aorta is through a ventricular septal defect, which, if restrictive, effectively causes subaortic obstruction. Figure 20.6 Three‐dimensional echocardiogram demonstrating an en face view of circumferential subaortic obstruction viewed from the left ventricle. The subaortic obstruction is indicated by the asterisks (*) and the ventricular septum by the open arrowheads (>). Figure 20.7 M‐mode echocardiogram of the long axis of the aortic valve. “Fluttering” of the aortic valve leaflets is indicated by the arrows and is due to the turbulent subaortic jet striking the aortic valve leaflets. Ao, aorta; LA, left atrium. Intraoperative imaging, either by an epicardial or TEE approach, is typically performed in cases of subaortic stenosis, whatever the anatomic substrate, to confirm adequacy of the surgical relief of obstruction. Alignment of the Doppler cursor to the direction of flow is typically best achieved by a transgastric approach but this can be difficult or impossible when the chest is open during surgery. The severity of aortic regurgitation should be assessed because this can occasionally worsen following removal of the fibrous subaortic obstruction, particularly in cases where fibrous tissue has extended onto the aortic valve leaflets themselves. The mitral valve should be imaged for evidence of mitral valve regurgitation (four‐chamber, 90°, and 120° views) as damage to the anterior leaflet may occur if a circumferential subaortic “membrane” has had to be surgically resected from the anterior mitral valve leaflet. Finally, it is necessary to guard against iatrogenic creation of a ventricular septal defect due to inadvertent perforation of the ventricular septum so this region should be scanned comprehensively. Figure 20.8 Transesophageal echocardiography of a patient with subaortic obstruction following a “Rastelli” repair of transposition of the great arteries with an associated ventricular septal defect (VSD) and pulmonary stenosis. The outflow of the left ventricle (LV) is across the VSD. Subaortic muscle (infundibulum, conus) can lead to a tortuous subaortic area and obstruction. The asterisk denotes the area of subaortic narrowing in this image. Ao, aorta; PA, pulmonary artery. Figure 20.9 Parasternal long‐axis 3D echocardiogram of subaortic stenosis following repair of an atrioventricular canal defect. This technique allows a depth of view to assist in delineating the extent of the fibrous subaortic stenosis, in this case extending onto the repaired superior bridging leaflet. LA, left atrium; LV, left ventricle; SBL, superior bridging leaflet; SubAS, subaortic stenosis. In the longer term, sequential echocardiography is indicated to exclude the recurrence of subaortic stenosis, which can occur even after initial apparent complete resection of the obstructive tissue. The risk of recurrence is higher when the subaortic “membrane” is closer to the aortic valve and in patients with severe preoperative obstruction [27,39]. Those with previously operated aortic arch interruption are also at particularly high risk for recurrence [40]. Aortic regurgitation should be assessed semiquantitatively and chamber dimensions, wall thickness, and contractile function should be measured. Aortic valve stenosis in children is due to a congenitally abnormal aortic valve rather than degeneration of a normal valve as may occur in late adult life. Morphologically, the anomaly may be due to commissural underdevelopment, myxomatous thickening of the valve leaflets, annular hypoplasia, or a combination of these elements. As a result of commissural underdevelopment, the aortic valve may be unicuspid (when only one commissure, usually the left noncoronary commissure, is well formed), bicuspid (when one commissure is underdeveloped and the other two are well formed), tricuspid (all three commissures are well developed), or, rarely, quadricuspid (when an extra commissure is present). 3D echocardiography has the potential to assist in defining valve morphology by permitting display of a depth of field so that both cusps can be visualized (Figure 20.10, Video 20.7). The morphology of the bicuspid aortic valve has assumed greater importance with recent surgical advances in repair rather than replacement of such valves [41]. In some cases, particularly in infancy, it may be difficult to identify true separate cusps, with the appearance of a single central or eccentric orifice, which has been likened to a “volcano‐type” or “keyhole” orifice [42]. In some patients the valve may be incompetent and stenotic. Aortic regurgitation is almost invariably present following balloon valvuloplasty, either surgical or transcatheter. Post‐stenotic dilation of the proximal ascending aorta also occurs due to the high velocity, turbulent, and often eccentric jet through the valve. Obstruction to the left ventricular outflow tract results in an increased afterload on the left ventricle. The myocardial response to the excess load depends on the stage of development at which it occurs. During fetal life, mild stenosis of the aortic valve is usually well tolerated with good contraction of the left ventricle and normal development of left heart structures. In fetuses with severe aortic valve stenosis, the left ventricle dilates, contractile function decreases, and endocardial fibroelastosis often develops [43]. As gestational age advances, growth of the left ventricle and aorta is inhibited in the majority of cases so that, by term, the left ventricle and aorta are hypoplastic and postnatal management is more akin to that of hypoplastic left heart syndrome [43,44]. Such progression of aortic stenosis provides the rationale for intrauterine intervention to prevent progression of aortic valve stenosis to hypoplastic left heart syndrome, with the potential to improve the likelihood of a biventricular repair postnatally [45–47]. Figure 20.10 Three‐dimensional echocardiogram of a bicuspid aortic valve viewed from the aortic side. In this example there was coexisting subaortic stenosis. The two cusps of the aortic valve are marked by the asterisks. Following birth, once the ductus arteriosus closes, the systemic arterial circulation has to be supported entirely by left heart structures. If there is severe aortic stenosis with significant left ventricular dysfunction and/or underdevelopment of left heart structures, then the left heart may be unable to support the circulation and the infant will present with cardiovascular collapse. In such circumstances, the administration of prostaglandin is life‐saving to allow reopening of the the ductus arteriosus and permit the right heart structures to support the systemic circulation. The term “critical” aortic stenosis is commonly applied to infants where the systemic circulation is dependent on the maintenance of ductal patency. In less severe cases, closure of the ductus arteriosus does not lead to left ventricular failure because the left ventricle hypertrophies in response to left ventricular outflow tract obstruction and maintains an adequate cardiac output. Such infants and children may remain asymptomatic early in life and present for other reasons such as cardiac murmur, reduced exercise tolerance, or exercise‐induced syncope. In adults, aortic valve stenosis may develop due to degeneration of an aortic valve that was not previously stenotic. Individuals with a congenitally bicuspid aortic valve have a higher risk of late degeneration of the valve than if the valve is tricuspid [48]. The goals of echocardiographic assessment of aortic valve stenosis are to comprehensively characterize the morphologic features of the obstructive lesion, to evaluate the hemodynamic severity, and to delineate associated anomalies. The severity of aortic valve stenosis is estimated by measurement of the Doppler‐derived maximum instantaneous and mean pressure drop across the aortic valve. Classification by Doppler‐derived pressure drop is only valid if left ventricular function is well preserved and cardiac output is normal. Assuming this to be the case, and beyond the neonatal age range, mild aortic valve stenosis may be defined as a peak Doppler velocity of less than 3 m/s; moderate aortic valve stenosis has a peak velocity of 3–4 m/s; and severe aortic stenosis is characterized by a peak velocity of more than 4 m/s. Color Doppler flow mapping is extremely helpful in observing the direction of the flow jet, which may be eccentric, and allows optimal alignment of the Doppler cursor with the jet (Figure 20.11). Imaging from the suprasternal notch often has the best alignment with the direction of flow. Use of the low‐frequency CW pedoff Doppler probe is recommended as it provides optimal access to the suprasternal notch, particularly in smaller children. In infants and children an apical three‐chamber projection provides an excellent window for Doppler interrogation of the left ventricular outflow tract. CW Doppler is optimal to determine the maximum instantaneous and mean Doppler‐derived pressure drop, but PW Doppler is extremely helpful in patients with multiple levels of left ventricular outflow tract obstruction. This is because “step up” in velocity at different sites may be observed and allows determination of the relative contribution of the different levels of obstruction. Figure 20.11 Parasternal long‐axis view with color Doppler of valvar aortic stenosis. (a) In systole demonstrating aortic valve (AoV) stenosis with an eccentric jet across the aortic valve, which is helpful to assess when measuring Doppler derived gradients. (b) In diastole demonstrating an eccentric jet of aortic regurgitation. Ao, aorta; LA, left atrium; LV, left ventricle. In older children and in young adults with congenital aortic valve stenosis, an assessment of valve area can be made by employing the continuity equation [49,50] or other formulae [51,52]. In adults with mild aortic valve stenosis, the effective orifice area is >1.4 cm2; in moderate aortic valve stenosis it is 1.0–1.4 cm2; and in severe aortic valve stenosis it is <1.0 cm2 [53]. One important group has relatively low “gradients” across the aortic valve due to reduced left ventricular ejection fraction resulting in a reduced cardiac output. In this group, severe aortic valve stenosis coexists with a relatively low Doppler velocity across the aortic valve. Dobutamine stress echocardiography examines for increases in effective orifice area, contractile function, and pressure gradient under stress conditions, permitting stratification of risk for surgery [53]. The morphology of the aortic valve in the assessment for bicuspid or unicuspid valve, is best seen with a parasternal short‐axis view of the aortic valve. The diameter of the aortic valve annulus is measured during systole from the parasternal long‐axis view. If there is aortic regurgitation, the parasternal short‐axis view is ideal for determining the site of regurgitation through the valve. 3D echocardiography can provide further information because the depth of field permits visualization of the whole cusp rather than the single cut plane of cross‐sectional techniques (Figure 20.12). Assessment of aortic regurgitation is discussed in detail in Chapter 6. Briefly, the severity can be assessed by the width of the vena contracta of the aortic regurgitation jet using color flow mapping from the parasternal long‐axis view, relative to the aortic annulus diameter. CW Doppler interrogation of the regurgitant jet can be used to estimate the pressure half‐time, and the degree and duration of reversal of flow in the distal aortic arch or descending aorta during diastole can be helpful to give a semiquantitative assessment of the degree of aortic valve regurgitation [54]. Holodiastolic reversal in the abdominal aorta is generally considered severe aortic regurgitation. Figure 20.12 Three‐dimensional TEE of an abnormal aortic valve from a patient who had undergone previous balloon dilation of the aortic valve. The noncoronary cusp (N) is deficient, whereas the left coronary cusp (L) and right coronary cusp (R) are more normal. RVOT, right ventricular outflow tract. In all cases of left ventricular outflow tract obstruction at any level, left ventricular dimensions and wall thickness should be carefully measured. These measurements can be obtained by cross‐sectional or M‐mode echocardiography of the left ventricle at the level of the tips of the mitral valve in either a parasternal long‐ or short‐axis projection. Alternatively, left ventricular volume, mass, and systolic function can be measured by 2D or 3D techniques (see Chapter 7 for further details). It is essential that Doppler‐derived pressure drop across a stenotic aortic valve is interpreted in light of left ventricular contractile function and blood flow rate. An example of this occurs in small infants with severe aortic stenosis in whom left ventricular contractile function may be so poor that only low‐velocity jets are observed across a critically stenotic aortic valve. A thorough examination of all left heart structures is essential when aortic valve stenosis is diagnosed. Aortic valve stenosis may coexist with other types of left heart obstruction including supramitral membrane, congenital mitral valve stenosis, subaortic stenosis, and coarctation of the aorta. In infancy, critical aortic stenosis is frequently accompanied by hypoplasia of left heart structures including the mitral valve, left ventricle, and aorta (Figures 20.13–20.15). Endocardial fibroelastosis is observed as a brightly echogenic lining to the left ventricle; in its mildest form this may involve only patchy areas of the papillary muscles but in its severest form it may line the entire left ventricular cavity (Figures 20.14 and 20.15). Estimation of the extent and severity of endocardial fibroelastosis may be assisted by magnetic resonance imaging (MRI) (Figure 20.16). In severely affected infants it may be difficult to judge whether left heart structures are capable of supporting the systemic arterial circulation or not. This poses a major challenge in terms of management because if left heart structures are judged adequate, an intervention to relieve the aortic valve stenosis may be attempted. However, if left heart structures are judged inadequate to support cardiac output a single ventricle (“Norwood”) palliation is generally adopted. Echocardiographic criteria have been developed to aid in decision‐making in this regard [55–57] and an online calculator developed by the Congenital Heart Surgeons’ Society is available (http://www.chssdc.org/content/chss‐score‐neonatal‐critical‐aortic‐stenosis; last accessed January 2021). Figure 20.13 Apical four‐chamber view of a newborn with critical aortic stenosis. In this example the left ventricle (LV) is dilated and more globular than normal. There is evidence of endocardial fibroelastosis (EFE) affecting mainly the papillary muscle apparatus and the ventricular septum. LA, left atrium; RA, right atrium; RV, right ventricle. Figure 20.14 Apical four‐chamber view of a newborn with critical aortic stenosis with associated hypoplasia of the left ventricle (LV) and mitral valve (MV). The LV is not apex‐forming, and the MV has shortened chordae. LA, left atrium; RA, right atrium; RV, right ventricle; TV, tricuspid valve. Figure 20.15 Apical four‐chamber view in a neonate with critical aortic stenosis and severe endocardial fibroelastosis as demonstrated by the echobrightness of the left ventricle. Such extensive endocardial fibroelastosis is regarded as an adverse prognostic sign for a biventricular repair. LA, left atrium; LV, left ventricle; RA, right atrium; RV, right ventricle. Figure 20.16 Magnetic resonance imaging may assist in delineating the extent of endocardial fibroelastosis (EFE) in infants with severe aortic stenosis. In this example late gadolinium enhancement has been used to demonstrate the EFE. LA, left atrium; LV, left ventricle; RA, right atrium; RV, right ventricle. In the preoperative assessment of the aortic valve, the echocardiographer should focus on the morphology of the aortic valve, noting the number of aortic valve leaflets, the morphology of the commissures, and whether or not any leaflets are fused. An accurate measurement of the aortic valve at the hinge points of the leaflets is essential for selection of the appropriate balloon size for valvuloplasty. In addition, the degree of aortic regurgitation should be carefully documented as an increase in the degree of regurgitation may occur following intervention. Balloon aortic valvuloplasty is favored as the initial intervention to relieve critical aortic stenosis although surgical valvotomy is the first treatment of choice in some institutions. Echocardiography may be undertaken during the procedure if a complication is suspected, such as perforation of the left ventricle with a guidewire or damage to the aortic valve. Echocardiography is not routinely performed during the interventional procedure unless a major complication is suspected but is performed shortly after the procedure to assess residual gradients across the aortic valve and to judge the severity of aortic valve regurgitation. Judging the severity of aortic valve regurgitation early after balloon valvuloplasty may be difficult because of reduced compliance of the left ventricle related to muscle hypertrophy or endocardial fibroelastosis. This may lead to underestimation of the degree of aortic regurgitation. Follow‐up echocardiography is indicated in all patients who have had intervention or surgery on the aortic valve. This is to evaluate restenosis of the aortic valve, particularly with growth, and to review the severity of aortic regurgitation, which is almost invariable following balloon aortic valvuloplasty or surgical valvotomy. Aortic valve stenosis may be diagnosed accurately during fetal life [43–45]. In mild cases of aortic stenosis, there is an increased Doppler velocity across the aortic valve with good left ventricular function and antegrade flow around the aortic arch. Growth of left heart structures is well maintained with advancing gestational age. Sequential echocardiography is indicated during fetal life to monitor for progression of aortic valve stenosis, as some fetuses will demonstrate progressively increased severity of left ventricular outflow tract obstruction. In severe cases of aortic stenosis diagnosed during fetal life, the left ventricle is typically dilated early in gestation with reduced systolic function (Figure 20.17, Video 20.8). Endocardial fibroelastosis is a frequent association, although the severity may vary. This appears as a bright echogenic lining of the left ventricular cavity. Mitral valve regurgitation may occur and, if severe, is associated with markedly dilated left atrium and poor prognosis (Figures 20.17 and 20.18, Videos 20.9 and 20.10) [58,59]. Often, retrograde flow can be seen in the transverse arch because antegrade flow across the aortic valve is inadequate (Figure 20.19). The interatrial communication, which normally permits right‐to‐left flow, may reverse due to left heart inflow obstruction. In some cases, the atrial septum may be highly restrictive or even intact (Figure 20.20). Doppler interrogation of the pulmonary veins, with particular attention to the degree and duration of flow reversal during atrial contraction, is extremely helpful in determining the degree of restriction of the atrial septum (Figure 20.21). Figure 20.17 Fetal echocardiogram of severe aortic stenosis. The left ventricle (LV) is dilated and compresses the right ventricle (RV). The left atrium (LA) is markedly enlarged due to severe mitral valve regurgitation. Figure 20.18 Fetal echocardiogram demonstrating severe mitral valve regurgitation (MR) in a fetus with critical aortic stenosis. There is a broad jet of mitral valve regurgitation, which fills a large portion of the dilated left atrium (LA). A pleural effusion is seen suggesting cardiac failure. LV, left ventricle; RA, right atrium. Figure 20.19 Fetal echocardiogram with color Doppler demonstrates the “three vessel” view of the upper mediastinum. In this case of critical aortic stenosis, there is antegrade flow in the ductus arteriosus but reversal of flow in the transverse aortic arch (Ao arch). L, left; R, right. Figure 20.20 Restriction interatrial communication in a fetus with critical aortic stenosis. There is a narrow turbulent jet of blood from the left atrium to the right atrium (RA) representing the only point of decompression of the left atrium. LV, left ventricle; RA, right atrium; RIAC, restrictive interatrial communication. Figure 20.21 Interrogation of the pulmonary veins can be helpful to assess the degree of restriction of the atrial septum in the context of severe left heart obstruction. (a) There is flow into the left atrium during ventricular systole (S) and diastole (D) with only a short low‐velocity period of flow reversal during atrial contraction. (b) There is a short period of normal flow into the left atrium during early ventricular systole only, with major reversal of flow during atrial systole. The fetus in (b) has a highly restrictive atrial communication. With advancing gestational age, growth of left heart structures is almost always limited so that by term, the aorta, left ventricle, and mitral valve are often severely hypoplastic. Judging which fetuses will be managed toward a biventricular circulation versus those that will be managed toward a single ventricle physiology is often difficult. The relative diameters and lengths of the right and left ventricles, presence of endocardial fibroelastosis, degree of mitral valve regurgitation, hypoplasia of the aorta, and direction of flow in the distal aortic arch may all help in prognostication [45]. Fetuses at the severe end of the spectrum will often require management with a single ventricle palliation using a Norwood strategy rather than balloon dilation of the aortic valve or surgical valvotomy. It is the progression of aortic valve stenosis to a situation analogous to hypoplastic left heart syndrome that has led to prenatal intervention being considered for affected fetuses [45–47]. The risks versus benefit of fetal cardiac intervention continues to be controversial but in recent years large international registries have been established to provide data to guide decision‐making in this regard [60]. The echocardiographic approach during fetal life includes all of the assessments listed earlier but in addition should also include: Supravalvar aortic stenosis typically occurs at the sinotubular junction and is characterized by discrete narrowing of the aortic lumen at this point (Figures 20.22 and 20.23, Videos 20.11–20.13). This condition is most often seen due to mutations in the elastin gene which is often seen in Williams–Beuren syndrome [61]. Marked thickening of the aortic media at the sinotubular junction produces the narrowing. The sinuses of Valsalva may be enlarged below the level of stenosis and tethering of the aortic valve leaflets (Video 20.14) and stenosis of the coronary ostia are frequent associations. In addition to narrowing at the level of the sinotubular junction, there may be varying degrees of hypoplasia of the ascending aorta (Figure 20.23), aortic arch, and stenoses of head and neck vessels [62]. Supravalvar aortic stenosis is extremely rare during fetal and early postnatal life but can become progressively more severe with age. It leads to increased afterload on the left ventricle and is associated with left ventricular hypertrophy when outflow tract obstruction is significant. In addition to the narrowing at the level of the sinotubular junction, the anomaly can involve the aortic valve with tethering and restricted leaflet motion. This can contribute further to the increased afterload on the left ventricle. Coronary ostial stenoses can occur in association with supravalvar aortic stenosis, which can lead to a precarious balance of coronary supply to the myocardium in the face of increased myocardial oxygen demand. Thus, patients with this condition are at risk for sudden death. Hypoplasia of the aortic arch and stenoses of the head and neck vessels can further increase the afterload on the left ventricle [62,63]. The abnormal composition of the vessel wall leads to increased medial hypertrophy and reduced elasticity of the vessel wall, which places further load on the left ventricle. The goals of echocardiographic assessment of supravalvar aortic stenosis are to comprehensively characterize the morphologic features of the obstructive lesion, to evaluate the hemodynamic severity, and to assess the entire thoracic aorta. Figure 20.22 Supravalvar aortic stenosis. (a) Parasternal long‐axis view demonstrates “pinching” of the aorta (Ao) at the level of the sinotubular junction. There is associated hypertrophy of the left ventricular walls. (b) With color Doppler in the same view, flow acceleration is seen above the aortic valve. LA, left atrium; LV, left ventricle; LVH, left ventricular hypertrophy; Suprav. AS, supravalvar aortic stenosis. Figure 20.23 Parasternal short‐axis view of a patient with supravalvar aortic stenosis. (a) The aortic valve is trileaflet and of normal size. (b) Slightly higher view of the sinotubular junction in the same case as (a). Note the markedly diminished size of the aorta reflecting a significant degree of stenosis in the supravalvar region. LA, left atrium; LPA, left pulmonary artery; MPA, main pulmonary artery; RA, right atrium; RPA, right pulmonary artery; RVOT, right ventricular outflow tract.
CHAPTER 20
Anomalies of the Left Ventricular Outflow Tract and Aortic Valve
Definitions
Incidence
Etiology
Subaortic stenosis
Morphology and classification
Pathophysiology
Imaging
Intraoperative and postoperative assessment
Aortic valve stenosis
Morphology and classification
Pathophysiology
Imaging
Echocardiographic evaluation before, during, and after intervention for aortic valve stenosis
Aortic valve stenosis in the fetus
Supravalvar aortic stenosis
Morphology
Pathophysiology
Imaging
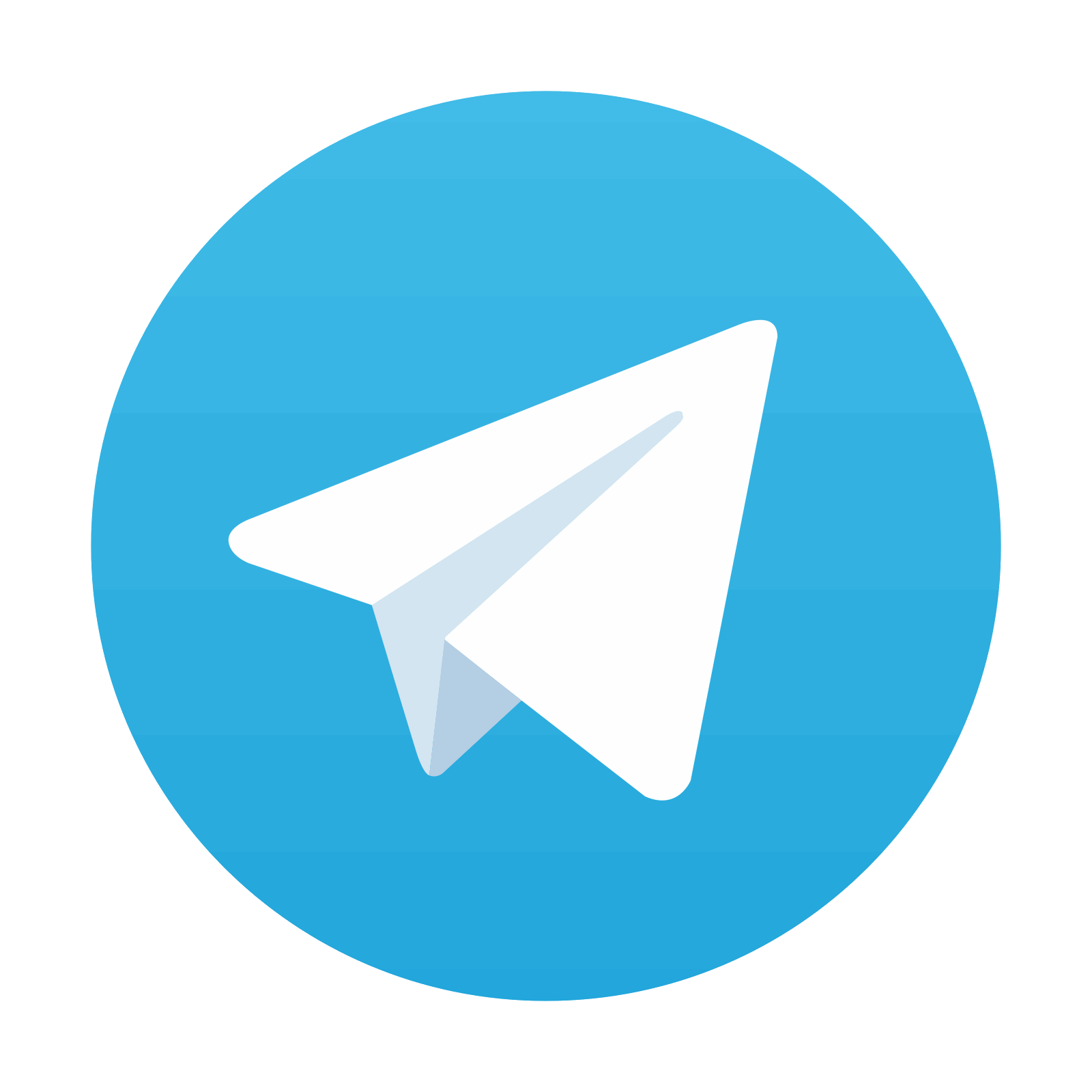
Stay updated, free articles. Join our Telegram channel

Full access? Get Clinical Tree
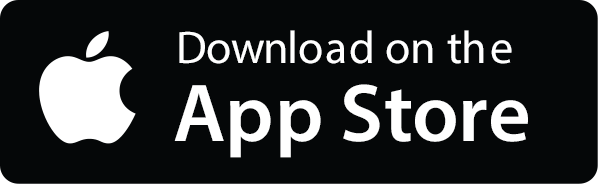
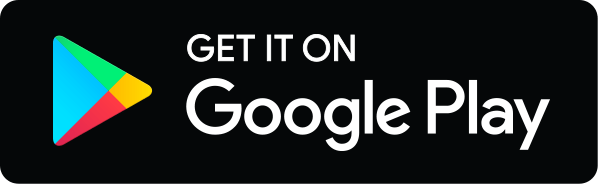