Jami C. Levine Boston Children’s Hospital, Boston, MA, USA Pulmonary atresia with intact ventricular septum (PA/IVS) is a relatively uncommon congenital heart defect characterized by luminal discontinuity between the right ventricular outflow tract and the main pulmonary artery in the absence of a ventricular septal defect. Peacock published the first case series of patients with PA/IVS in 1869 [1]. This included a historical review in which he credits John Hunter for the first description of this anomaly in 1783. Grant first described the associated coronary artery abnormalities in 1926 [2]. There were anecdotal case reports in the mid‐1900s, including a series of 10 cases described by Abbott in her seminal Atlas of Congenital Heart Disease [3]. Glaboff et al. published the first detailed description of PA/IVS with a dilated right ventricle (RV) in 1950 [4]. From 1955 through 1970, a number of reports described the anatomic features of PA/IVS [5–12]. The first attempts at surgical palliation were reported in the 1960s [6,8,13]. Initially, surgical therapy was technically successful but did not significantly improve outcome. Over the ensuing decades, the diagnostic evaluation has become more refined and the treatment plans more tailored to the anatomic variants, resulting in dramatically improved prognosis for infants with PA/IVS [14,15]. In the New England Regional Infant Cardiac Program, 75 of 2251 infants (3.3%) had PA/IVS, and 85% of these were diagnosed in the first week of life [16]. Estimates of the incidence of PA/IVS vary from one in 8000 live births [17] to one in 144,000 live births [16]. Without treatment, 50% of infants die within 2 weeks, and 85% die by 6 months of age [7,17–20]. Death is typically due to complications of cyanosis secondary to insufficient pulmonary blood flow. Both genetic and environmental factors have been implicated in the etiology of PA/IVS, but little is known about specific contributing factors. It is likely that genetic factors, when important, are multifactorial. From empiric data, it appears that PA/IVS is not one of the more heritable forms of congenital heart disease [21] and is only occasionally associated with specific chromosomal anomalies. There are, however, anecdotal reports of pedigrees that include more than one family member with PA/IVS, including a few reports of affected siblings [22,23]. The role of environmental factors in the etiology of PA/IVS is also unknown. Evidence that environmental factors can directly influence the development of the right heart comes from a report by Baetz‐Greenwalt et al. [24]. This paper described 14 infants with right heart hypoplasia, all diagnosed within a 2‐year period in a small area of Ohio. Of this group, 79% had extracardiac anomalies, prompting speculation that an environmental exposure in the second month of pregnancy may have produced a new syndrome, which included abnormalities of right heart growth. There are several theories about the morphogenesis of pulmonary valve atresia [925–27]. Most popular is the flow theory, which assumes that an anatomic abnormality early in development decreases blood flow through the right heart, resulting in diminished growth of right heart structures. The lack of growth leads to further decrease in flow through the right heart. Eventually, this cycle ends in acquired atresia of the pulmonary valve. Several structural defects have the potential to disrupt flow through the RV, including primary abnormalities of the foramen ovale, Eustachian valve, tricuspid valve, and pulmonary valve. It is likely that the timing of valve atresia is important and that infants with the smallest right heart structures are the ones who have experienced the earliest progression to atresia, whereas those with dilated right hearts may have had antegrade flow for a longer period, thus allowing for more in utero growth of the right heart. It is worth noting, however, that the cause and effect of diminished flow through the right heart and its etiologic association with PA/IVS have not been established. There is significant morphologic heterogeneity among patients with PA/IVS [7,9,11,14,25–32]. Initial anatomic studies suggested that patients could be divided into two relatively distinct groups depending on the size of their right heart structures [5,7–9,25–31]. Those with pulmonary atresia and hypoplasia of the RV were designated as type I, whereas those with pulmonary atresia and normal or enlarged right heart structures were designated as type II. Type I patients had small, abnormal tricuspid valves, severe right ventricular hypertrophy, and coronary artery fistulae, whereas type II patients were more likely to have severe tricuspid regurgitation, a thin‐walled and dilated RV, and a massively enlarged right atrium, but no significant coronary artery fistulae. It is clear from more contemporary anatomic studies that there is a continuum with respect to right heart disease, yet the majority of patients tend to have features that grossly fit into one of these two subtypes. The vast majority of patients with PA/IVS have a patent foramen ovale, although ~20% of patients have a secundum atrial septal defect (ASD) [9]. The right atrium and its associated caval connections may be dilated and the right atrial wall may be hypertrophied, although this is probably dependent on the amount of tricuspid regurgitation, the size of the foramen ovale, and the length of time that the hemodynamic abnormalities were present. Studies suggest that 70–90% of patients with PA/IVS have hypoplasia of both the tricuspid valve and RV, with up to 60% of patients having right ventricular hypoplasia that is severe [14,26,28,30,33]. Hypoplasia typically involves all segments of the RV, although some portions may be so underdeveloped as to be absent [34]. The RV sinus (inlet) is often foreshortened (Figures 18.1 and 18.2). The moderator band may be poorly defined, although when present can be severely hypertrophied. The infundibular portion of the RV is frequently hypoplastic, and in about 10–25% of patients is atretic [31,32,34]. The ventricular endocardium is typically fibrotic and in some cases there may be diffuse endocardial fibroelastosis as well as myocardial fiber disarray [25,35–38]. The size of the tricuspid valve typically correlates with the size of the RV [25–27,30–33]. In the majority, the valve annulus is small and the leaflets and supporting apparatus are morphologically abnormal. The Congenital Heart Surgeons’ Society (CHSS) multicenter study found that in 50% of patients, the tricuspid valve annulus diameter z‐score is less than −2 and in 10% it is less than −5 [14]. In addition to annular hypoplasia, the leaflets are often thickened, the chordae tendineae are short, and the papillary muscles are small or poorly defined. These abnormalities often result in tethered leaflets with reduced mobility [6,7,9,15,25,32,39]. Figure 18.1 Severely hypoplastic right ventricle (RV) in pulmonary atresia with intact ventricular septum. Four‐chamber view from the apical window demonstrating a diminutive right ventricular cavity and a severely hypoplastic tricuspid valve annulus. LA, left atrium; LV, left ventricle; RA, right atrium. Figure 18.2 Moderately hypoplastic right ventricle (RV) in pulmonary atresia with intact ventricular septum. Four‐chamber view from the apical window. The RV reaches about two‐thirds of the way to the apex with a normal tricuspid valve annulus diameter. The ventricular septum bows into the left ventricle (LV) due to the suprasystemic right ventricular pressure. Abnormalities of the coronary artery origins are relatively common in PA/IVS [14,25,27,32,40–43]. In addition, RV sinusoids are often present. These are endothelial‐lined channels within the myocardium that communicate with the RV cavity. These are remnants of the sinusoidal spaces that nourish the myocardium in early fetal life. When they persist in postnatal life, they can be a site of communication between the RV cavity and the coronary arteries. These fistulous communications are found in as many as 50% of patients with small, hypertensive RVs [14,28,40–45]. Their prevalence increases with increased RV pressure. The smaller the RV and tricuspid valve, the more likely one is to find fistulous communications from the ventricle to the coronary arteries. Stenotic lesions within the coronary arteries or atresia of one or both coronary artery ostia at the aortic root can also be present. Severe coronary artery stenoses and/or ostial atresia disrupt the flow of oxygenated blood from the aortic root to the myocardium. The myocardium that does not receive antegrade flow from the epicardial coronary arteries may be perfused entirely with desaturated blood from the RV through the persistent sinusoids [42,43,46]. This condition is known as a right ventricular‐dependent coronary artery circulation (Figures 18.3 and 18.4, Video 18.1) and is present in 10–20% of patients with PA/IVS [14,31,40,42,45,47–49]. These severe coronary artery abnormalities are much more common in the minority of patients with muscular atresia of the infundibulum [50]. The epicardial coronary arteries may also be abnormal, exhibiting intimal proliferation, nodularity or endarteritis [28,37,45,51]. Figure 18.3 Coronary fistula in right ventricular‐dependent coronary artery circulation. Angiography is done in the aortic root. The left coronary can be seen proximally and the stenosis is seen in the mid‐portion of the left anterior descending artery. The aneurysm is not obvious at this stage of the cardiac cycle. LMCA, left main coronary artery. Figure 18.4 Coronary fistula in right ventricular‐dependent coronary artery circulation. This image is identical to that in Figure 18.3, but depicts a different phase of the cardiac cycle. Now the aneurysm of the coronary artery is easily visualized just distal to the stenotic area. LMCA, left main coronary artery. By definition, there is luminal discontinuity between the RV and the main pulmonary artery. In almost all cases the pulmonary valve is atretic but the subpulmonary infundibulum is patent. Approximately 70–80% of the patients have a small but patent infundibulum and membranous atresia of the valve, often with recognizable pulmonary valve tissue and definable commissures (Figures 18.5 and 18.6, Video 18.2) [20,25,27]. The remainder have muscular infundibular atresia with or without overlying fibrous valve tissue (Figure 18.7, Video 18.3) [14,18]. Rarely, the infundibulum is atretic and the pulmonary valve is patent [52]. When there is membranous atresia, the pulmonary valve annulus diameter is usually normal or mildly hypoplastic and is rarely diminutive. The main and branch pulmonary arteries are usually normal in size [9,18]. In the CHSS database, only 6% of patients had significant branch pulmonary artery hypoplasia [14]. Rarely, the main pulmonary artery is absent. An absent main pulmonary artery and no identifiable pulmonary valve annulus is much more likely if there is muscular atresia of the right ventricular infundibulum [50]. In all types, pulmonary blood flow is typically through a patent ductus arteriosus [45,51]. Figure 18.5 Pulmonary valve (PV) morphology in pulmonary atresia with intact ventricular septum. This en face image is taken from the high left parasternal window with the marker pointing straight left. The main pulmonary artery sinuses appear essentially normal. The commissures are frequently well delineated, but thickened and fused. Figure 18.6 Right ventricular outflow tract in membranous pulmonary atresia seen from the subxiphoid short‐axis view of the outflow tract. Note the small but well‐formed right ventricular outflow with a good‐sized pulmonary valve (PV) annulus. Infund, infundibulum; RV, right ventricle. The RV is dilated in ~5–10% of patients with PA/IVS [14,28,31,32]. RV enlargement may be mild, but patients in this group can have massive cardiac enlargement. Figure 18.7 Right ventricular outflow tract in muscular pulmonary atresia. This subxiphoid short‐axis view demonstrates a small right ventricle (RV) with no obvious infundibular cavity. Instead there is muscular obliteration of the outlet and no identifiable pulmonary annulus. LV, left ventricle. In contrast to patients with right ventricular hypoplasia, patients in this group typically have severe tricuspid regurgitation. The right atrium is often severely dilated and there may be associated dilation of the venae cavae. The patent foramen ovale is often stretched with right‐to‐left atrial level shunting. The tricuspid valve annulus and RV are both larger than normal. In many, the right heart becomes so dilated that it compresses the left heart, compromising cardiac output (Figure 18.8). The heart may have a “wall‐to‐wall” appearance on chest radiography, similar to severe Ebstein anomaly (Figure 18.9). In some patients with PA/IVS, Ebstein anomaly coexists, with significant apical displacement of the septal leaflet, leading to poor coaptation and severe tricuspid regurgitation [29,32,34,53,54]. In others, the tricuspid valve is hinged normally at the annulus but the leaflets are redundant, dysplastic, and sail‐like. Because of the tricuspid regurgitation, the RV is unlikely to be hypertensive and endocardial fibroelastosis is uncommon. The RV tends to be thin‐walled and dilated. Uhl anomaly of the RV is uncommon, but has been reported [55]. Patients with a dilated RV typically have membranous atresia of the pulmonary valve, although rarely they may have an associated muscular atresia of the infundibulum. Because the RV in this group of patients decompresses during systole through tricuspid regurgitation, significant right ventricular hypertension is uncommon and anomalies of the coronary arteries are rare. Figure 18.8 Apical four‐chamber view demonstrating a severely dilated right ventricle (RV) and right atrium (RA). The tricuspid valve annulus is severely dilated and the tricuspid valve leaflets are elongated. The RV is significantly larger than the left ventricle (LV). The RA is severely dilated and the atrial septum (S) deviates leftward, making the left atrium (LA) appear much smaller than usual. Figure 18.9 Chest radiograph in newborn with severe right heart dilation and pulmonary atresia with intact ventricular septum. Note that the borders of the cardiac silhouette extend from the right chest wall to the left chest wall. Similar to the right ventricular hypoplasia group, the pulmonary valve annulus, main pulmonary artery, and branch pulmonary arteries are likely to be normal in size or mildly undersized. It is unusual for branch pulmonary arteries to be diminutive, discontinuous, or absent. Normal cardiac position and segmental anatomy are present in ~98% of patients with PA/IVS. There have been reports of associated morphologic abnormalities of the left heart, but hemodynamically significant left heart disease is rare [31,56,57]. Fetuses with PA/IVS and normal‐sized or hypoplastic RVs usually survive pregnancy. Conversely, those with severe tricuspid regurgitation and massive dilation of the right heart are at higher risk for arrhythmias, hydrops fetalis, and intrauterine demise. After birth, the systemic venous return crosses the atrial septum through the foramen ovale and mixes with the pulmonary venous return in the left atrium, which results in cyanosis. The ductus arteriosus remains the only source of pulmonary blood flow, and survival depends on maintaining ductal patency. The systolic pressure in the RV depends on the pathways available for blood to exit the chamber. In the presence of a competent tricuspid valve the systolic pressure is usually very high, whereas in those with severe tricuspid regurgitation the RV pressure may be normal or only mildly elevated. In the postnatal circulation, coronary artery abnormalities can begin to have a negative impact on myocardial function in a number of ways. In those with right ventricular‐dependent coronary artery circulation, oxygen delivery to the myocardium depends on the perfusion pressure and oxygen content in the RV. If either of these falls significantly, myocardial ischemia can result. In addition, with or without right ventricular‐dependent coronary artery circulation, increased intracavitary RV pressure may lead to chronic subendocardial ischemia. Finally, the presence of RV‐to‐coronary artery communications can allow for a right‐sided circular shunt [51]. Blood can go from RV to coronary artery to coronary vein to coronary sinus to right atrium and back to RV without passing through the systemic capillary bed. Any or all of these factors can cause chronic and/or intermittent ischemia, which can adversely affect both systolic and diastolic myocardial properties [56,58–61]. Postoperative physiology varies greatly depending on the operation performed. The most dramatic changes are those occurring after decompression of the RV. As ventricular pressure falls, myocardium that was perfused through sinusoids may experience at least a transient decrease in blood flow, leading to myocardial ischemia. The degree of ischemia depends on the extent to which blood from the aortic root can reach those myocardial segments through the epicardial coronary arteries. If there are significant coronary artery stenoses, the myocardial segments distal to those stenoses may become profoundly ischemic and severe ventricular dysfunction may ensue [47,48]. Even without coronary stenoses, if there are large coronary–cameral fistulae, then these may cause “steal” of blood flow from the coronary arteries as RV pressure falls [58,59]. Ventricular dysfunction can be transient, although for some patients it is profound and irreversible. Evidence from prenatal studies indicates that early atresia of the pulmonary valve can be associated with an arrest of right heart growth in utero [62]. This observation has led to speculation that in utero restoration of antegrade pulmonary blood flow may stimulate growth of the RV and tricuspid valve. Over the past decade, reports of successful in utero pulmonary valve dilation have been published [62,63]. Although in some cases growth of the RV, tricuspid valve, and outflow tract has been observed after the intervention, this was not seen in all [62,64–68]. Intervention for restrictive flow across the pulmonary valve has been technically more challenging with less predictable outcome than has been seen in interventions for restrictive aortic valve flow. Because PA/IVS is rare, the number of attempted interventions remains small. As experience accumulates, it is reasonable to expect that better information will become available concerning the risks, benefits, and criteria for patient selection. Treatment strategy is often based on the anatomy of the coronary arteries as well as the size of the right heart. The goal of treatment is to achieve a biventricular circulation in as many infants as possible without compromising myocardial perfusion [1469–98]. For the majority of infants, coronary artery flow is not dependent on the RV and a biventricular repair can be considered. The first step is creation of antegrade pulmonary blood flow. This can be achieved by placement of a right ventricular outflow tract patch, surgical valvotomy, or by transcatheter perforation and balloon dilation of the pulmonary valve [7179–81,90,91,97]. The hope is that restoring antegrade pulmonary blood flow will stimulate right heart growth, ultimately giving the patient an RV that can carry a full cardiac output. If antegrade flow is established and significant cyanosis persists, an alternate source of pulmonary blood flow may be needed. This is most commonly achieved by placement of a Blalock–Taussig shunt or stenting of the ductus arteriosus [91–93,97]. Once stable pulmonary blood flow is established, patients are followed with noninvasive imaging to assess tricuspid valve and RV growth. If the RV grows sufficiently, then an attempt is made to occlude or ligate any accessory sources of pulmonary blood flow and close any existing atrial level shunts, either surgically or with an implantable occlusion device. In many case series, there is a subset of patients who thrive after a right ventricular outflow tract procedure and a shunt, yet fail attempts to remove accessory sources of pulmonary blood flow from the circulation. In some of these patients a strategy has been pursued in which the atrial communication is closed and a bidirectional Glenn shunt is performed (called 1.5 ventricle repair). This allows inferior vena caval flow to be carried by the RV while flow from the superior vena cava is delivered directly to the pulmonary vascular bed [94,95,99]. These strategies are not available to patients with right ventricular‐dependent coronary artery circulation due to their risk for myocardial ischemia and death if the RV is decompressed and the ventricular pressure decreases. Therefore, a single ventricle management strategy is pursued. The first step is to create a stable source of pulmonary blood flow with a systemic‐to‐pulmonary artery shunt or ductus arteriosus stent. Eventually, palliation is achieved with a Fontan‐type circulation in the first few years of life [1486–88]. Without establishment of antegrade pulmonary blood flow, there is no growth of the RV and it usually remains hypoplastic and hypertensive. There is a paucity of long‐term outcome data in these patients, but medium‐term follow‐up suggests that the overall survival into young adulthood for patients with PA/IVS and a single ventricle circulation is ~80% [86–88]. Management of patients with PA/IVS and massive RV dilation is challenging. This group has higher rates of in utero demise, newborn morbidity, and postoperative mortality. There have been some successful attempts at creating a biventricular circulation by performing tricuspid valvuloplasty in addition to placement of a right ventricular outflow tract patch. In some cases, this may be done in association with a surgical reduction in the size of the RV and/or right atrium. Rarely, pulmonary valvotomy or a right ventricular outflow tract patch alone is sufficient. Some of these patients will require single ventricle strategy. If the tricuspid regurgitation is severe, a Starnes procedure can be performed. In the Starnes procedure, a fenestrated patch is placed over the tricuspid valve to exclude it and the RV from the circulation along with a systemic‐to‐pulmonary artery shunt [100]. PA/IVS was first diagnosed by M‐mode echocardiography in the late 1970s [101,102]. By the mid‐1980s, 2D echocardiography had supplanted M‐mode, increasing the available anatomic detail [103,104]. With the addition of color and spectral Doppler technology, noninvasive evaluation has become more detailed and increasingly important for diagnosis and assessment of prognosis. Increasingly, the diagnosis of PA/IVS is being made in utero. Many have shown that the anatomic details seen by echocardiography can help to define which fetuses are at risk for worse postnatal outcomes [50,105–118]. Because this lesion frequently causes a change in chamber size, it is relatively easy to identify on an obstetric screening exam. Unfortunately, right ventricular hypoplasia is not always obvious early in the second trimester and therefore screening ultrasound at 14–18 weeks of gestation may only identify the most severe cases. Early diagnosis can be made more often if color Doppler is used to evaluate tricuspid valve function, flow through the right ventricular outflow tract and pulmonary valve, and the direction of ductal flow. Fetal evaluation includes detailed assessment of the right heart structures, including tricuspid valve size and function; RV morphology, size, and function; presence of coronary artery fistulae; and morphology of the right ventricular outflow tract and the pulmonary arteries. There may also be a role for ventricular strain evaluation in the fetus, although the importance of these data to overall diagnosis and prognosis is still unclear [112]. Evaluation of the tricuspid valve includes measurements of the annulus diameter in the four‐chamber and long‐axis planes and depiction of the valvar and subvalvar apparatus anatomy (Figures 18.10–18.13, Videos 18.4 and 18.5). Color Doppler is used to assess valve inflow and regurgitation (Figure 18.13, Video 18.6). Spectral Doppler is used to assess the inflow pattern and to estimate RV systolic pressure when a regurgitation jet is present. The RV should be evaluated in all views with attention to length, degree of hypertrophy, and degree of systolic dysfunction. Color Doppler is used to screen for coronary artery fistulae. Magnifying the RV and interrogating the cavity and myocardium by color Doppler with a low Nyquist limit allows visualization of coronary artery fistulae in some fetuses. Fistulae are seen as streams of color signals within the myocardium and jets into the cavity during diastole (Video 18.7). The right ventricular outflow tract and main pulmonary artery are imaged to evaluate pulmonary annulus size and outflow tract patency. Color Doppler is used to determine the presence or absence of flow across the pulmonary valve. In the presence of flow from the aorta to the pulmonary artery through the ductus arteriosus, the pulmonary valve may not open even when it is not anatomically atretic (functional atresia). In this case, detection of pulmonary regurgitation excludes pulmonary valve atresia. The main and branch pulmonary artery anatomy and size are documented. The ductus arteriosus is evaluated with 2D imaging and color Doppler. In PA/IVS, flow is from the aorta to the pulmonary arteries, the reverse of normal flow direction (Figure 18.12, Video 18.8). Figure 18.10 Four‐chamber view of a fetal heart illustrating moderate to severe right ventricular hypoplasia. Note that the right ventricular cavity is markedly hypoplastic and the right ventricular apex is shorter than the left ventricular apex. The tricuspid valve annulus diameter is small and the atrial septum deviates into the left atrium. LV, left ventricle; RA, right atrium; RV, right ventricle. Figure 18.11 Four‐chamber view of a fetal heart in pulmonary atresia with intact ventricular septum and right ventricular dilation. The image illustrates that the heart spans the entire width of the chest. It also extends posteriorly, although one can see small amounts of lung tissue behind the left atrium (LA) and left ventricle (LV). The tricuspid valve (TV) annulus is dilated and the leaflets are redundant. RA, right atrium; RV, right ventricle. Figure 18.12 Fetal aortic arch (Ao arch) as viewed in long axis through the back. The flow in the ductus arteriosus is towards the pulmonary arteries, the reverse of the usual intrauterine pattern. PDA, patent ductus arteriosus. Figure 18.13 Four‐chamber view of the fetal heart in pulmonary atresia with intact ventricular septum and right heart dilation, illustrating severe tricuspid regurgitation (TR). TV, tricuspid valve The atrial septum is evaluated to document unrestrictive right‐to‐left flow at the atrial level. As with all fetuses, the echocardiogram includes an evaluation of left heart anatomy and function as well as Doppler evaluation of the umbilical vessel, ductus venosus, and inferior vena cava. The flow profile in the umbilical vessels and hepatic veins can be markedly abnormal in the presence of significant tricuspid regurgitation (Figure 18.14). Studies in fetuses with PA/IVS show that a smaller tricuspid valve diameter and RV size are predictive of worse hypoplasia of the right heart at birth, and a lower likelihood of achieving a biventricular outcome [110–113]. Sequential prenatal imaging can add potentially valuable prognostic information about growth velocity of the tricuspid valve and right ventricle. [109,111]. Although no studies have documented that prenatal diagnosis of PA/IVS leads to improved postnatal outcome, some studies of other duct‐dependent congenital heart diseases have suggested decreased morbidity and neurologic sequelae in prenatally diagnosed patients [117,118]. Similar to the prenatal evaluation, the postnatal echocardiogram includes a comprehensive anatomic survey with particular attention to the right‐sided structures. Surgical strategy will depend on the details of tricuspid valve and RV size and anatomy, coronary artery ostial patency, the presence of coronary sinusoids, and both valvar and ventricular function. While a multimodality approach may be needed to plan the best surgical strategy, the evaluation typically starts with a high‐quality transthoracic echocardiogram [119–127]. Every effort should be made to optimize conditions for high‐quality imaging, including adjustment of lighting, use of high‐frequency transducers, and minimization of patient motion. The ability of the RV to fill and, therefore, to grow depends on the size of the tricuspid valve and on the size and compliance of the ventricle. Numerous studies have demonstrated that tricuspid valve annulus diameter predicts long‐term outcome and should be considered when planning the intervention strategy [1483–85,113,120–122]. Therefore, it is of utmost importance to obtain clear images of the tricuspid valve and its supporting apparatus and to measure the annulus diameters in orthogonal views. The apical four‐chamber view depicts the lateral diameter (see Figures 18.1 and 18.2) and the parasternal long‐axis view angled towards the right hip shows the anterior–posterior diameter (Figure 18.15). An annulus diameter z‐score of less than −3 is associated with a lower likelihood of tolerating RV decompression as the sole intervention [14]. The subvalvar apparatus can be difficult to image due to right ventricular hypertrophy (Figure 18.16). It is often best seen in the short‐axis sweeps from the parasternal and the subxiphoid acoustic windows. 3D imaging of the subvalvar apparatus can help clarify the anatomy in some patients. Color and spectral Doppler are used to assess inflow and regurgitation (Figure 18.17, Video 18.9). Figure 18.14 Spectral Doppler in the hepatic veins in a fetus with pulmonary atresia and intact ventricular septum. Flow below the baseline is toward the heart and flow above the baseline is away from the heart. This tracing shows a prolonged period of no flow in the hepatic veins as well as a short period of retrograde flow (away from the heart). Typically, hepatic venous flow is toward the heart, occasionally with very short periods of no flow. This tracing is consistent with severe tricuspid regurgitation.
CHAPTER 18
Pulmonary Atresia with Intact Ventricular Septum
Definition
Historical background
Incidence
Etiology
Morphology
Pulmonary atresia with right ventricular hypoplasia
Right atrium
Right ventricle and tricuspid valve
Coronary arteries
Pulmonary valve and arteries
Pulmonary atresia with right ventricle dilation
Right atrium
Right ventricle and tricuspid valve
Coronary arteries
Pulmonary valve and arteries
Associated structural heart disease
Pathophysiology
Preoperative
Postoperative
Treatment
Prenatal
PA/IVS with right ventricular hypoplasia
PA/IVS with right ventricle dilation
Imaging
Prenatal assessment
Preoperative assessment
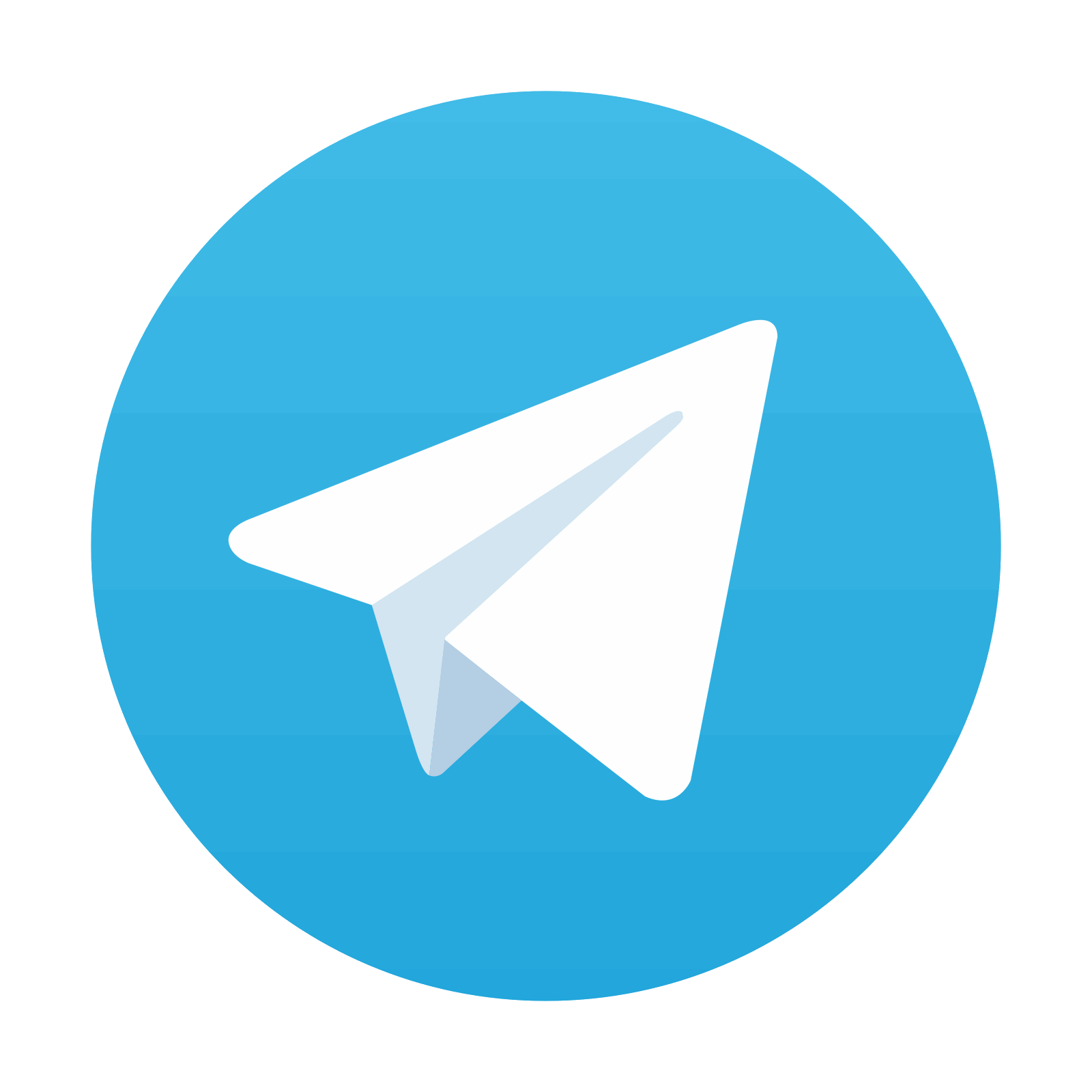
Stay updated, free articles. Join our Telegram channel

Full access? Get Clinical Tree
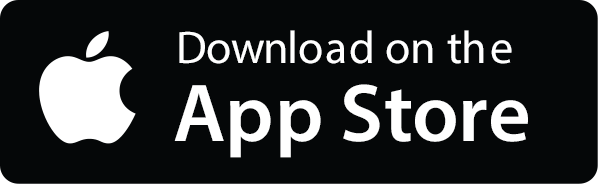
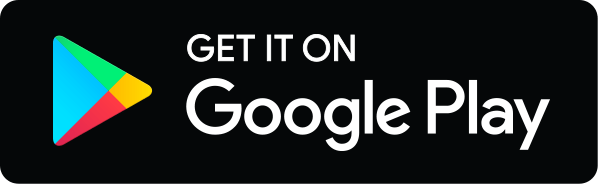