Shobha Natarajan and Meryl S. Cohen The Children’s Hospital of Philadelphia, Philadelphia, PA, USA A ventricular septal defect (VSD) is defined as a communication between the left and right ventricles or between the left ventricle and the right atrium. VSDs are amongst the most common abnormalities of the heart. They can be present in isolation or in association with other congenital cardiac abnormalities. In fact, a VSD is a component of abnormalities such as tetralogy of Fallot, double outlet ventricle, truncus arteriosus, transposition of the great arteries, and common atrioventricular (AV) canal defect. VSDs are also frequently seen in association with aortic arch anomalies. This chapter describes VSDs that occur in the setting of normally related great arteries. VSDs in association with conotruncal anomalies are described in other chapters. The reported incidence of VSDs has varied widely, due at least in part to varying diagnostic methodologies and the natural history of VSDs. Large population‐based studies provide sufficient live births (denominators) at the expense of an inability to detect all with congenital heart disease (numerators), whereas intensive, sensitive studies usually cannot be done on very large populations [1]. Given the high propensity of VSDs to close spontaneously, starting as early as in utero, the incidence of VSD would be much higher if all fetuses were examined, somewhat lower if all newborns were examined, even lower if only those newborns with murmurs were examined, and lower still if subjects were first evaluated at 1 year of age [2]. Prior to the wide availability of echocardiography, the incidence of VSDs was reported between 30 and 240 per 100,000 births [3–5]. More recently, a higher incidence of VSDs has been reported, particularly in premature infants [6–10]. Utilizing screening echocardiography in neonates, a muscular VSD was diagnosed in 5320 and 5660 per 100,000 term and preterm babies, respectively [8,9]. Groups reporting a high incidence have also reported high rates of spontaneous closure of these defects. The perceived increase in the incidence of VSDs may be due to increased sensitivity of perinatal and neonatal surveillance rather than to a true increase in incidence. Between 10,000 and 11,000 infants with an isolated VSD are diagnosed annually in the United States [5,11,12]. Between 15% and 20% of these patients require surgery [13]. If VSDs in association with other congenital heart anomalies were included, the incidence of VSDs would be much higher. Similar to other congenital heart defects, the etiology of VSDs is multifactorial. Moreover, different types of VSDs likely arise from different developmental abnormalities. VSDs are quite prevalent in association with genetic syndromes, especially in trisomy 13, 18, or 21 as well as with other less common syndromes [14–16]. In the autosomal dominant Holt–Oram syndrome, both atrial and ventricular septal defects are inherited in association with limb deformities as a result of mutations in the gene encoding the transcription factor TBX5 [17]. The Baltimore–Washington Infant Study has reported an association between paternal use of marijuana and cocaine and paternal occupation such as jewelry making and paint stripping with the occurrence of VSDs in their offspring [18,19]. Detailed description of the development of the human heart is provided elsewhere [20–31]. Septation of the ventricles involves a complex series of events. The transitional zone between the embryonic left ventricle (LV) and right ventricle (RV) is characterized by the expression of “neuromuscular” markers. These markers form a ring around the primary interventricular foramen, termed the “primary ring” [26,32–34]. This ring separates the upstream portion (the atria and LV) from the downstream portion (the RV and outflow tract) of the heart. Myocardialization and fusion of the endocardial cushions in the outflow tract (outflow tract ridges) leads to the formation of a muscular outlet that includes the conal (outlet) septum [35]. Although controversy exists regarding the septal components, the muscular interventricular septum is formed from three components: (i) the primary, or trabecular, component that arises from the septum separating the trabeculated cavities of the developing RV and LV and becomes the muscular septum; (ii) the AV canal or inlet component that develops from the AV cushions (controversy exists about this component); and (iii) the outlet septum that develops from the outflow tract ridges. The muscular septum is identifiable between the trabecular portions of both ventricles at 5 weeks’ gestation. The fibrous membranous septum is formed by the fused major (superior and inferior) AV cushions and the mesenchymal cap of the primary atrial septum. The bifurcation of the ventricular conduction system is the landmark that separates the contribution of the AV cushions and the outflow tract ridges to septation [21]. A thorough understanding of the anatomy of the ventricular septum is critical to fully describe and categorize VSDs and optimize treatment strategies. The natural history and prognosis of VSDs vary according to the location and borders of the defect(s). Treatment choices are expanding for some types of VSDs to include transcatheter and innovative surgical options. Several schemes for nomenclature and classification have been proposed and debated. Further discussion about this nomenclature is beyond the scope of this chapter. For the purposes of echocardiography, we choose to define VSDs primarily by their geographic location rather than by their surrounding structures. However, both components are important for complete characterization of the VSD. Thus, for each VSD type, several of the names that are in common usage will be discussed and are listed in Table 13.1. Recently, the International Society for Nomenclature of Paediatric and Congenital Heart Disease (ISNPCHD) has come to a consensus about the categorization of VSDs using geographic location as the initial point in the hierarchy but also emphasizing whether there are fibrous or muscular borders to the defects [13,36]. The four geographic regions include central perimembranous (e.g., perimembranous or conoventricular VSD), inlet (AV canal type with or without malalignment of the ventricular septum or posterior muscular VSD), outlet (conal septal or doubly committed subarterial with or without malalignment), and trabecular (exclusively in the muscular septum). Viewed from the RV, the ventricular septum may be divided into four zones: (i) inlet septum; (ii) muscular (trabecular) or ventricular sinus septum; (iii) outlet septum; and (iv) membranous septum (Figure 13.1) [37,38]. The smooth‐walled inlet septum extends inferiorly and posteriorly adjacent to the septal leaflet of the tricuspid valve to the distal septal attachments of the tricuspid valve papillary muscles. Extending inferiorly and anteriorly is the muscular (trabecular) septum, which is characterized by coarse trabeculations on the RV aspect and includes the moderator band. The muscular (trabecular) septum is separated from the smooth‐walled outlet (infundibular, conal) septum by the septal band (septomarginal trabeculation), which courses superiorly and posteriorly from the mid‐portion of the ventricular septum. The septal band divides into an anterosuperior limb, which courses superiorly toward the pulmonary valve and blends into the subpulmonary conus (infundibulum), and a posteroinferior limb, which courses towards the RV free wall, where it merges with the parietal band (septoparietal trabeculation). The conal (infundibular) septum sits within the “Y” of the septal band and in the normal heart is difficult to distinguish from the conal free wall (it becomes more clearly recognized when it is displaced from the “Y” of the septal band). The membranous septum, the fibrous component of the ventricular septum, sits at the inferior edge of the conal septum just superior to the inlet septum. The membranous septum is divided by the septal leaflet of the tricuspid valve such that a portion sits on the right atrial side (a defect in this area results in the rare left ventricular to right atrial defect – the Gerbode defect [39]) while the other portion is interventricular and is in continuity with all other components of the ventricular septum. On the LV side, the membranous septum sits adjacent to the right coronary–noncoronary commissure of the aortic valve. The conduction tissue, consisting of the AV conduction bundle, the branching bundle, and left bundle branch, courses in the posterior–inferior border of the membranous septum. Table 13.1 Glossary of terms used to describe ventricular septal defects * This terminology excludes the ventricular septal defect seen in the atrioventricular canal defect, which is considered a separate entity. ISNPCHD, International Society for Nomenclature of Paediatric and Congenital Heart Disease [36]. Figure 13.1 Normal right ventricular (RV) septal wall demonstrating normal landmarks of the ventricular septum. The “Y” indicates the “Y” configuration of the septal band to help define ventricular septal defect location. A central perimembranous defect is the most common type of VSD that requires surgical closure. It is centrally located in the ventricular septum. This defect has various other names including conoventricular, perimembranous, membranous, and paramembranous because of its close proximity to or inclusion of the membranous septum. However, many other defects are also located adjacent to the membranous septum. Thus, the terms “perimembranous” or “paramembranous” are nonspecific with regard to location but are better used to describe borders. Central perimembranous defects are located between the conal (infundibular) septum and the muscular (trabecular) septum near the septal–anterior commissure of the tricuspid valve (Figure 13.2). These defects typically result in fibrous continuity between the tricuspid valve and aortic valve but, in some cases, there is a small muscular rim between the aortic valve and the defect. The tricuspid valve frequently has attachments to the margins of the defect, partially or completely restricting the ventricular level shunt. In some cases, adherence of the tricuspid valve leaflets to the edges of the VSD may cause shunting from the LV directly into the right atrium (not to be confused with a true Gerbode defect). On the LV side, central perimembranous VSDs lie adjacent to the commissure between the right and noncoronary cusps of the aortic valve. Thus, in some cases, a portion of an aortic valve leaflet (most commonly from the right coronary cusp) may prolapse into the VSD and cause aortic regurgitation. These defects may also be associated with the late development of muscle bundles in the right ventricular outflow tract, so‐called double‐chambered RV as well as subaortic membranes. Figure 13.2 Central perimembranous ventricular septal defect (VSD). (a) Pictorial view of the right ventricular (RV) septal wall showing a central perimembranous type VSD (in black). The defect is seen in proximity to the septal leaflet of the tricuspid valve and encompasses the membranous septum as well. (b) A pathologic specimen in the same view of the RV septal wall showing a central perimembranous‐type VSD sitting under tricuspid valve septal attachments. The “Y” of septal band is represented by the black Y to show its relationship to the defect. An inlet‐type VSD is seen just under the septal leaflet of the tricuspid valve and spans the entire length of the valve (Figure 13.3). The distinction between an inlet‐type VSD and a posterior muscular defect is that the inlet‐type VSD has no superior muscular rim between the AV valve and the defect. Both, however, are inlet defects. Importantly, in an inlet‐type VSD that is perimembranous, the conduction tissue may course posterior to the defect resulting in a typical counterclockwise loop on electrocardiogram [40]. For the purposes of this chapter the discussion is limited to inlet defects that are not in association with an AV canal defect. In inlet VSD without AV canal defect, there are distinct tricuspid and mitral valves with separate annuli. Inlet‐type VSDs are often associated with a straddling tricuspid valve. This straddling is typically associated with malalignment between the atrial and ventricular septum with variable degrees of RV hypoplasia [41]. Figure 13.3 Inlet ventricular septal defect (VSD). (a) Pictorial view of the right ventricular (RV) septal wall showing an inlet‐type VSD. Notice that the defect sits along the entire length of the tricuspid valve and that the perimembranous portion of the ventricular septum is also included in the defect. (b) A pathologic specimen in the same view of the RV septal wall showing an inlet‐type VSD. The tricuspid valve is straddling the defect. An outlet‐type VSD without malalignment occurs in the outlet portion of the ventricular septum and is defined as complete or partial absence of muscle in the conal septum (Figure 13.4). Many other names have been used to describe this type of VSD including conal septal defect, doubly committed subarterial defect, subpulmonary defect, juxta‐arterial defect, and supracristal defect. This type of defect occurs more frequently in the Asian population. It is important to distinguish outlet defects from conoventricular defects though they are relatively close in location. Outlet defects do not close spontaneously and often require a surgical approach through the pulmonary valve for closure. With complete absence of the conal septum, there is fibrous continuity between the adjacent cusps of the aortic and pulmonary valves. Due to the close proximity of these defects to the right coronary cusp of the aortic valve and the lack of support to the aortic valve from below, there is a strong tendency for the right coronary cusp of the aortic valve to prolapse into the VSD, resulting in partial or complete closure. Figure 13.4 Outlet ventricular septal defect (VSD). (a) Pictorial view of the right ventricular (RV) septal wall showing an outlet‐type VSD. A portion of the conal septum is absent. The defect is adjacent to the pulmonary valve. Notice that the membranous septum is intact. (b) A pathologic specimen in the same view of the RV septal wall showing an outlet‐type VSD. Notice its close proximity to the pulmonary valve and that the defect is just above the “Y” of septal band. Malalignment defects are defined by the orientation of the conal (infundibular) septum. As such, they are defects in the outlet portion of the ventricular septum. In malalignment defects, the conal septum which normally sits in the “Y” of the septal band, is positioned out of that plane either toward the right ventricular outflow tract (i.e., tetralogy of Fallot) or toward the left ventricular outflow tract (associated with subaortic narrowing) (Figure 13.5). Some refer to malalignment defects as conoventricular or perimembranous defects with malalignment. Importantly, malalignment defects almost never close spontaneously and generally do not have tricuspid valve tissue attached to the margins of the defect. In rare circumstances, AV valve tissue may be “pulled” into the defect, obstructing ventricular level flow and potentially obstructing an outflow tract. Malalignment defects are commonly associated with other conotruncal malformations such as double‐outlet ventricle or transposition of the great arteries. In the setting of normally related great arteries, anterior malalignment makes the conal septum an exclusively RV structure that impinges on the subpulmonary region resulting in: (i) subpulmonary obstruction; (ii) the aorta positioned over the ventricular septum (overriding aorta); and (iii) right ventricular hypertrophy. These components in addition to the VSD itself make up the diagnosis of tetralogy of Fallot. When anterior malalignment is severe, the conal septum may fuse with the free wall of the right ventricular outflow tract resulting in pulmonary atresia. In cases of mild anterior malalignment with a normal‐sized pulmonary valve, there may be no significant pulmonary outflow tract obstruction; these defects are called “Eisenmenger‐type” VSDs because the pulmonary vascular bed is not protected from increased flow [42]. When the conal septum is malaligned in the posterior direction, it becomes an exclusively LV structure; as a result, the aorta sits entirely above the LV (Figure 13.5). It is almost always associated with subaortic narrowing and usually associated with coarctation of the aorta or interruption of the aortic arch. When posterior malalignment is severe, aortic atresia may be present. Muscular VSDs are the most common defects seen in the fetus and newborn. Muscular defects are present in the trabecular portion of the ventricular septum and are entirely surrounded by muscle. When small, many muscular VSDs will close spontaneously without intervention. Typical designations of more specific locations include anterior, posterior, mid‐muscular, inferior, and apical (Figure 13.6). In autopsy specimens, muscular VSDs are readily seen on the LV septal surface because of the fine trabeculations but they may be covered by dense and coarse trabeculations on the RV side, giving the appearance of multiple defects – the so‐called “Swiss cheese” septum [43]. Multiple defects in the same patient are also common. Accurate echocardiographic location of these defects is important if surgical or catheter‐directed closure is considered. The Gerbode defect is defined as a communication between the left ventricle and the right atrium in the membranous portion of the ventricular septum that sits above the tricuspid valve [38]. True Gerbode defects (in contrast to defects that result from adherence of the tricuspid valve commissure to the VSD rim) are extremely rare. A VSD may have two closely related adverse consequences: altered hemodynamics due to a left‐to‐right shunt, and alteration of the pulmonary vascular resistance. Figure 13.5 Malalignment ventricular septal defect (VSD). (a) Pictorial view of the right ventricular (RV) septal wall showing a malalignment‐type VSD. Note that the defect is anterior and superior to where a central perimembranous defect sits. In this example, anterior malalignment of the conal septum is depicted. The aortic valve is shown in this figure because it is overriding the ventricular septum (tetralogy of Fallot). (b) A pathologic specimen in the same view of the RV septal wall showing an anterior malalignment‐type VSD. The conal septum is seen deviated toward the pulmonary valve which is small (i.e., tetralogy of Fallot). The “Y” of the septal band is represented by the black Y to show the relationship to the defect. (c) A pathologic specimen of the left ventricular septal wall showing a posterior malalignment‐type VSD. The conal septum is seen projecting into the left ventricular outflow tract with a small aortic valve. Figure 13.6 Muscular ventricular septal defect (VSD). (a) Pictorial view of the right ventricular (RV) septal wall showing multiple locations of muscular‐type VSDs (in black). All possible locations of muscular defects are labeled including the posterior muscular defect that sits behind the tricuspid valve. (b) A pathologic specimen in the same view of the RV septal wall showing a large mid‐muscular‐type VSD. Left‐to‐right shunting across a VSD leads to increased pulmonary blood flow. The magnitude of the left‐to‐right shunt depends on VSD size and the resistance in the pulmonary vascular bed. Based on both these factors, VSDs are broadly divided into three categories: small, moderate, and large [44]. Small VSDs are variably pressure‐restrictive, preventing transmission of systemic pressure and usually maintaining a normal RV pressure. Of note, a substantial left‐to‐right shunt can occur through smaller, pressure‐restrictive defects as the pulmonary vascular resistance drops over the first weeks of life. Large VSDs offer little restriction to pressure or flow such that the RV pressure is usually at systemic levels and the left‐to‐right shunt is large. A moderate VSD usually provides some resistance to pressure but little resistance to flow. As a result, this defect leads to variable elevation of RV pressure, and hemodynamically significant left‐to‐right ventricular shunting. Left‐to‐right shunting across a VSD may lead to three adverse hemodynamic consequences: (i) LV volume overload; (ii) pulmonary overcirculation; and (iii) potentially compromised systemic cardiac output [13]. The LV becomes volume overloaded because the blood flow essentially is ejected into the pulmonary artery, bypassing the RV. The increased volume load on the LV leads to dilation, which produces elevation of end‐diastolic pressure via the length–tension relationship [45]. LV hypertrophy develops as a compensatory mechanism to minimize the increase in wall tension, but it also decreases ventricular compliance, thus further aggravating the increase in end‐diastolic pressure [46]. This elevation in end‐diastolic pressure is reflected in increased left atrial and pulmonary venous pressure. The subsequent increase in hydrostatic pressure of the pulmonary capillary bed leads to the development of pulmonary interstitial fluid and even pulmonary edema [47]. Pulmonary compliance and gas exchange may be altered as a result. A hemodynamically significant VSD leads to a “common ejectile force,” where RV and pulmonary arterial systolic pressure are systemic. Increased pulmonary blood flow and increased pulmonary arterial pressure may alter the normal maturation of the pulmonary vascular bed [48]. As the pulmonary vascular resistance drops over the first few weeks after birth, left‐to‐right shunting of blood increases, leading to pulmonary overcirculation. As such, symptoms such as tachypnea, tachycardia, diaphoresis with feeds, and failure to thrive occurs in infancy. Without intervention, this pulmonary overcirculation leads to changes in the pulmonary vascular bed, limiting the left‐to‐right shunt and decreasing symptoms [49]. The pulmonary arteriole vessel transitions from having a reactive muscular wall to one with potentially irreversible structural changes consisting of medial hypertrophy and intimal proliferation, leading to elevated pulmonary vascular resistance. Eventually, pulmonary vascular resistance elevates to the point of exceeding systemic vascular resistance, leading to right‐to‐left shunting across the VSD and cyanosis, the so‐called Eisenmenger syndrome. Ventricular septal defects often occur in isolation. However, in surgical series, nearly 50% of all patients with a VSD have an additional cardiac anomaly [50]. Atrial septal defects (including patent foramen ovale), pulmonary stenosis, and a persistent left superior vena cava are seen in up to 13%, 15%, and 8% of VSD patients, respectively [51]. Patent ductus arteriosus in association with VSD is also seen frequently. Double‐chambered RV and subaortic stenosis, either separately or in combination, have been reported in up to 10% of patients with a central perimembranous VSD in surgical series [52,53]. Aortic regurgitation is an acquired and frequently progressive lesion in some patients with VSD [54]. It develops in association with central perimembranous or outlet‐type VSDs. The outlet VSD lies directly below the right coronary cusp of the aortic valve, accounting for the strong tendency of this cusp to prolapse into the VSD. Prolapse of the right coronary cusp of the aortic valve leads to defective apposition of the leaflets. In addition, absence of the conal septum may cause deficient support for the valve apparatus from below. In contrast, the central perimembranous VSD lies below both the right and noncoronary cusps of the aortic valve. Aortic regurgitation in this setting has been attributed to prolapse of either of these cusps but most frequently the right coronary cusp [55]. The left coronary cusp of the aortic valve is distant from the ventricular septum, and prolapse of this leaflet into a VSD is quite uncommon.
CHAPTER 13
Ventricular Septal Defects
Definition
Incidence
Etiology
Morphology and classification
Development
Anatomy
Ventricular septum
ISNPCHD
Van Praagh, Weinberg
Anderson
Other
Central perimembranous
Conoventricular, membranous
Perimembranous
Paramembranous, infracristal
Inlet*
Atrioventricular canal type
Perimembranous inlet
Outlet
Conal septal, conal septal hypoplasia
Doubly committed subarterial
Subpulmonary defect, juxta‐arterial defect, supracristal defect
Outlet with malalignment
Malalignment, conoventricular with malalignment
Perimembranous with malalignment
Trabecular, muscular
Muscular (with naming location: anterior, mid, posterior, apical)
Muscular (with naming location: inlet, outlet, trabecular, apical)
Central perimembranous VSD
Inlet VSD
Outlet VSD
Malalignment VSD
Muscular VSD
Gerbode‐type VSD
Pathophysiology
Left‐to‐right shunt
Alteration of pulmonary vascular resistance
Associated defects
Imaging
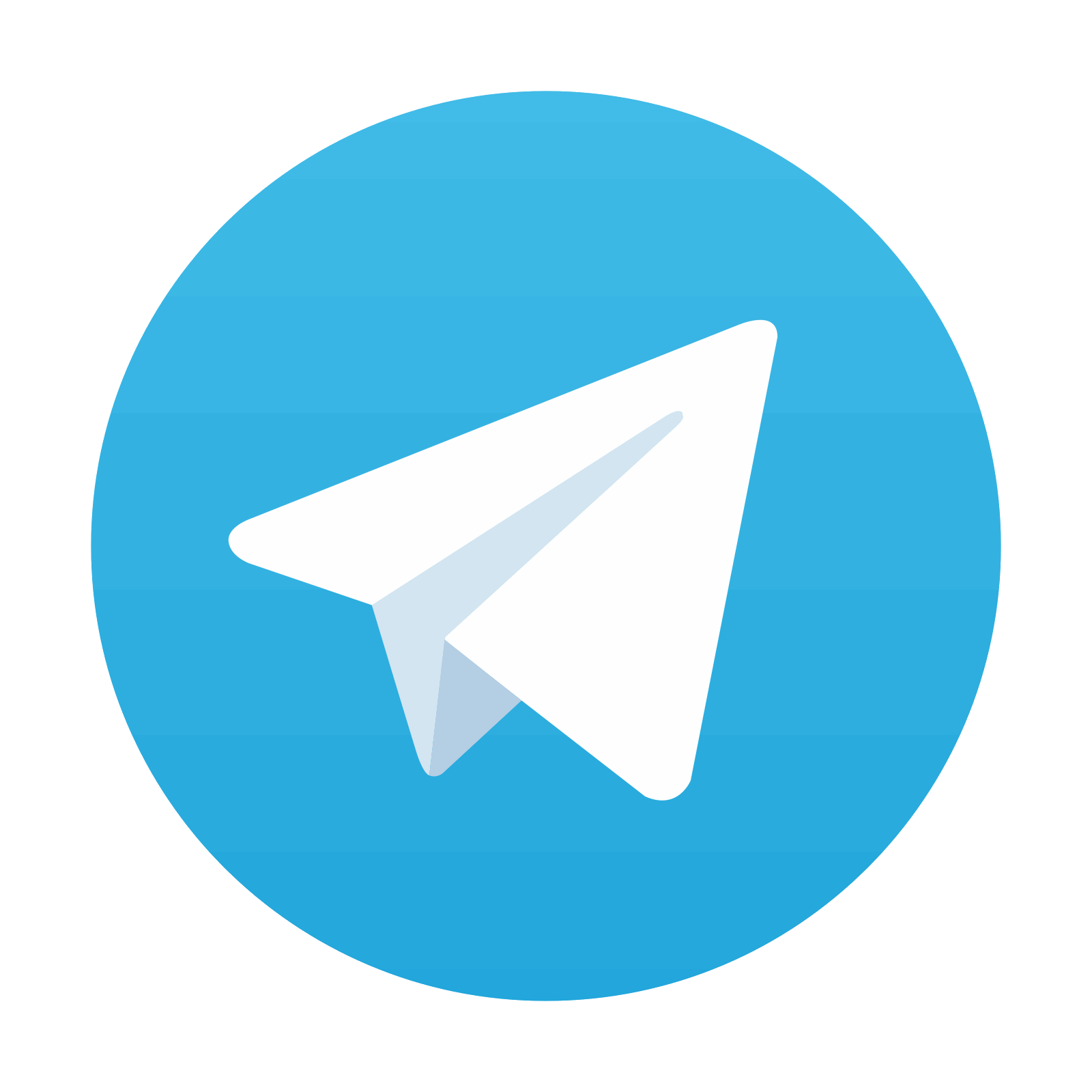
Stay updated, free articles. Join our Telegram channel

Full access? Get Clinical Tree
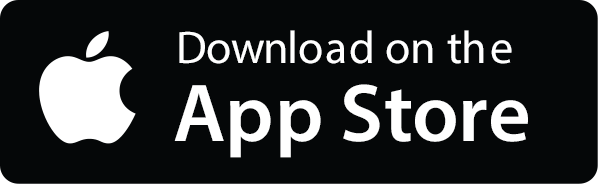
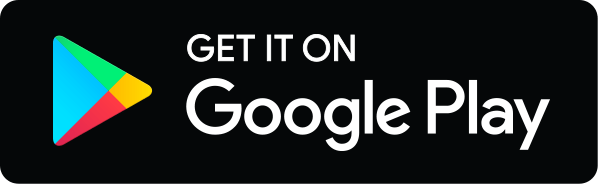