Signe Beebe Ozone (O3) is a blue, gaseous molecule, composed of three atoms of oxygen with a cyclical structure; ozone is O3 oxygen is O2. O3 occurs naturally in the stratosphere and acts as a filter to protect the earth from harmful ultraviolet radiation. It has a distinctive pungent acrid odor and is explosive in its liquid or solid form. O3 can also be formed from electrical discharges that catalyze the formation of ozone from atmospheric oxygen during lightning strikes and can often be smelled after a storm. O3 is a highly reactive, unstable form of oxygen that cannot be stored; and due to its instability, the half-life of ozone is temperature-dependent and quite short (at 20°C, O3 concentration will be reduced by half within 40 min) upon which it reverts back to the more stable form of oxygen [1–3]. The word “ozone” comes from the Greek word “ozein,” which means “smell” and was first isolated by the German-Swiss chemist Christian Friedrich Schönbein in the mid-nineteenth century [4]. The effects of O3 have been studied and recorded for over 150 years, and the medical use of O3 for water treatment, disinfection, and its antimicrobial properties have been well documented [1–3]. However, the use and application of O3 in veterinary medicine is still in the developmental stage. O3 was initially used as a microbicidal molecule in 1856 and subsequently used as an operating room disinfectant and in water treatment. The first medical use of O3 is said to have been during World War I for the treatment of gangrene in German soldiers, and to follow, a Swiss dentist began to use O3 in endodontic treatments [1]. Since this time, the medical use of O3 has been expanded and its use today is based on its anti-oxidant, immunostimulant, anti-microbial, and anti-protozoal properties. In addition, current research shows the potential use of O3 to treat HIV and cancer [5–7]. Although O3 is well known as a toxic gas, its medical use in low concentrations has been well-established as a promising medical modality for many different conditions because of its broad mechanism of actions [8–11]. Medical O3 (also known as oxygen-ozone therapy) is produced by a medical generator that uses high voltage electrical discharge as the source of energy to produce a standardized medical oxygen-ozone gas. Medical O3 concentration should be composed of no less than 95% O2 and no more than 5% O3 [2, 8]. In this process, oxygen molecules are pulled apart and reorganized in the form of O3. The first ozone generator was developed in 1857 by the French chemist Marius Paul Otto and the first O3 generator in the US was patented by Nikola Tesla in 1896, later forming the “Tesla Ozone Company,” in the late nineteenth century [12]. Chronic inflammatory processes are characterized by high oxidative stress, reactive oxygen species, suppression of antioxidant capacity, and immunologic dysregulation, each of which in turn creates a perpetual cycle of promotion and maintenance of the inflammatory process. In these cases, medical O3 therapy is thought to act as a bioregulator to help control and stop these chronic processes. O3 is one of the most powerful known oxidizing agents, surpassed only by flourine and persulphate [1, 12–14]. O3 as an oxidant, shows a paradoxical activity when it encounters organic molecules, causing a powerful antioxidant response. Antioxidants are critical for the maintenance of cellular integrity and cytoprotection. Once O3 contacts body fluids and tissues it rapidly reacts with all macromolecules (lipids, proteins, carbohydrates, and DNA) of the cellular membranes [15] to create hydrogen peroxide (ROS-reactive oxygen species) and a mixture of lipid ozonation products (LOP), mainly composed of 4-hydroxynonenal (4-HNE), one of the major end products of lipid peroxidation and an inducer of oxidative stress, and trans-4 hydroxy-2-hexenal (4-HHE) from polyunsaturated fatty acids (PUFA). These two important groups of by-products, ROSs that rapidly stimulate important biochemical processes in the early phase and LOPs, in the later phase, act as secondary messengers to promote and modulate the nuclear factor-erythroid-derived 2-related factor 2 (Nrf2) pathway. Nrf2 is a key transcription factor that coordinates expression of genes encoding antioxidant and detoxifying enzymes and stimulates the synthesis of several substances such as: γ-glutamyl transferase, γ-glutamyl transpeptidase, HSP-70, HO-1, and antioxidant enzymes such as such as super-oxide dismutase (SOD), glutathione peroxidase (GSH-Px), catalase, and glucose-6-phosphate dehydrogenase (G6PDH). This process represents the basis of the paradoxical phenomenon, for which an oxidizing molecule, such as O3, triggers a potent anti-oxidant reaction [9, 15] (Figure 18.1). At a therapeutic dose, O3 acts as a modulator or pro-drug and by inducing secondary messengers, facilitates the ensuing adaptive responses by the body. The main goal behind the use of repeated O3 treatments is to create resistance against oxidative stress (oxidative preconditioning) through the stimulation of the antioxidant system to produce cytoprotective effects in order to help treat and control different pathological conditions [16–19]. Figure 18.1 Ozone therapy: Paradoxical mechanism of action. The antimicrobial effects are one of the most important properties of O3. The oxidant activity of O3 has long been used to disinfect and kill bacteria, viruses, fungi, yeast, and protozoa. In bacterial, infections O3 therapy disrupts the bacterial cell wall through oxidation of the phospholipids and lipoproteins. This action is non-specific and selective to microbial cells; it does not damage human body cells because of their major antioxidative ability [20, 21]. After the membrane is damaged by oxidation, its permeability increases, and O3 molecules can enter the cells [19–21]. In viral infections, O3 damages the viral capsid and disrupts the reproductive cycle via peroxidation [19, 22–25], and destroys fungi via the same oxidative mechanism. In its gaseous and oiled forms, O3 has been successfully used in the treatment of epidermophyton, microsporum, and trichophyton dermatophyte infections, showing inhibitory effects on sporulation via inhibition of enzymes required for multiplication [25, 26]. A study on yeasts evaluated the fungicidal effects of O3 on different forms of Candida albicans that showed O3 was highly effective in killing C. albicans in yeast form and inhibited germ tube formation [27]. O3 has demonstrated activity to destroy protozoa (leishmania giardia, cryptosporidia, and microsporidia) in vitro, using ozonated oil and ozonated water [19, 28, 29]. O3 can stimulate cells of the immune system due to its reaction with polyunsaturated fatty acids (PUFA) in cellular membranes. When in contact with biological fluids, it forms reactive oxygen species as well as lipid oxidation products. After the peroxidation compounds are formed, H2O2 can then diffuse into immune cells and regulate signal transduction, stimulate cellular/humoral immunity/phagocytic function and immune responses such as reduction of cytokines (interleukin-1, interleukin-6, tumor necrosis factor-α, and interleukin-10 etc.), induction of interferon-γ, and others. O3 also has a promoting effect on vascular endothelial growth factor (VEGF), platelet-derived growth factor (PDGF), and TGF-β [30–34]. Many studies have suggested O3 therapy as an effective therapeutic option in the management of musculoskeletal disorders [11, 35–38]. The activation of Nrf2-antioxidant signaling pathway is thought to attenuate NF-κβ, a key regulator of the inflammatory response and prevent muscle atrophy, as discussed in several in vivo studies [39–41]. Other studies have assessed the effects of O3 on musculoskeletal pain. It is well known that pain is a common clinical sign related to the inflammatory process, and O3 therapy might play a key role in the modulation of nociceptive pain perception [11, 42]. O3 has a broad range of action, making it an effective therapy for many types, conditions, and diseases in veterinary medicine (Table 18.1). O3 generators today can generate a precise volume and oxygen-ozone concentration where ozone constitutes 3–5% of the total. The therapeutic effects of O3 are dose-dependent, and safe when given by the recommended route. The same type of precautions for systemic administration of any medication should be taken when using medical O3. The use of the appropriate product or concentration of O3 for the condition is essential to avoid toxicity [1, 2, 12]. Rapid administration is to be avoided and breathing in O3 directly can be toxic to the lungs. Adverse effects occur when ozonation products overwhelm the body’s anti-oxidant system, and can lead to toxicity that includes the formation of free radicals and reactive intermediates, induction of lipid peroxidation chain reaction, destruction of biomolecules and enzymes, and alteration of cellular membranes with tissue destruction [19, 43]. O3 can be administered via the systemic [44] and local routes and the volume of ozone will be different depending on the route of administration. Table 18.1 Medical ozone therapy by species. There are few research studies available on medical O3 therapy in veterinary medicine, and most of the current literature is specific for its use in humans. The author recommends reading the reviews that have been recently published [10, 19] in the last three years, which indicate an increasing interest in O3 as a treatment modality. Two excellent systematic reviews of the current literature on the use of O3 therapy in veterinary medicine were published in 2020 and 2021 [45, 46]. Both of these reviews discussed the application of O3 therapy in multiple species. The results of one review [46] showed that two-thirds of the articles selected on the use of O3 in veterinary medicine were published in the past decade, and the remainder from 2000–2010. The majority of the articles on the use of O3 as a healing agent referred to experimental studies. Fifty of the 117 papers documented clinical research on laboratory animals or in vitro. The best results were seen on the use of O3 in cattle and small ruminants where the therapeutic effects of O3 were confirmed by a large number of articles [10
18
Veterinary Ozone and Prolotherapy
Veterinary Ozone
Introduction
Ozone Paradoxical Mechanism of Action
O3 Antimicrobial Effects
O3 Immunomodulation Effects
O3 Anti-inflammatory and Analgesic Effects
Ozone Method of Administration and Veterinary Applications
Species
Application
Canine
Stifle/hip/elbow arthritis
Intervertebral disk disease
Back and neck pain
Conjunctivitis
Dentistry/tooth infection
Wound healing
Bacterial infection
IBD
Anal fistula
Ulcerative colitis/proctitis
Adjunct for cancer
Dermatitis
Otitis
Feline
Dentistry/tooth infection
Dermatitis
Adjunct for cancer
Equine
Laminitis
Back pain
Osteoarthritis
Dentistry
Wound healing
Bovine
Clinical and subclinical mastitis
Metritis
Retained of fetal membranes
Urovagina
Wound healing
Podermatitis
Lagomorph
Dermatitis
Tooth infection
Ovine/Caprine
Acute foot infection
Retained fetal membranes
Wound healing
Porcine
Herniated disk
Wound healing
Ozone Studies/Research in Veterinary Medicine
Stay updated, free articles. Join our Telegram channel

Full access? Get Clinical Tree
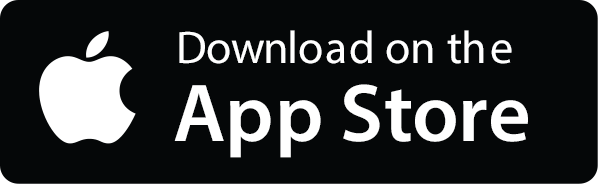
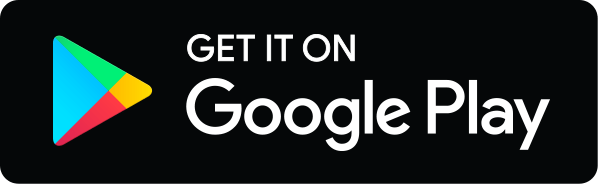