Fig. 1.
Clinical aspects of canine spinal cord injury. (a) T1-weighted transverse MRI scan of the spinal cord close to the thoracolumbar junction in a normal dog; (b) comparable level transverse T1W MRI scan in a dog with right sided intervertebral disk extrusion causing compression of the spinal cord (white arrow ); (c) surgical appearance of the spinal cord during surgical decompression, hematoma of the spinal cord parenchyma is visible through the dura mater (black arrow ) (c: Hidalgo A, with permission).
In contrast to the relatively high degree of homogeneity of lesion appearance between individuals in the acute stages after contusive injury, pathological heterogeneity, especially with regard to the extent of demyelination–remyelination and formation of cystic structures or syringomyelia (Fig. 2), is readily apparent in more chronic stages of clinical SCI in both dogs and humans. In terms of potential for limiting functional recovery, intraspinal cavitation is important because it may have profound effects on both axonal sprouting and the ability to remyelinate (32). The chronic phase of the injury also highlights a key problem with modeling SCI in the laboratory, because the post-injury scarring process in the mouse spinal cord differs greatly from that in the rat, dog and human. In mice progressive necrosis and cavity formation does not occur as it does in other species. Instead there is formation of a continuous connective tissue matrix, much as occurs in non-neural tissue (33). This phenomenon is likely to be specific to mice because it does not occur in rats or hamsters (34). Thus, genetically modified mice, while useful to answer specific research questions, may not be appropriate for examination of all aspects of pathogenesis or gene activation patterns following SCI. In contrast, canine clinical cases model accurately the patterns of chronic sequelae that occur in humans and which may represent obstacles to successful translation of strategies from rodent to human.
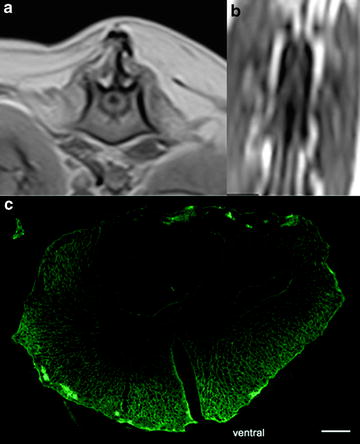
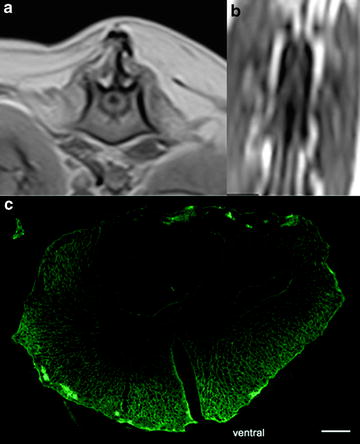
Fig. 2.
Post-injury spinal cord cavitation. (a) Transverse T1W MRI scan of spinal cord immediately caudal to the injury level showing a cavity (which appears black) within the parenchyma; (b) same lesion viewed in dorsal plane T1W MRI scan—note that the volume of the lesion can be derived from images (a) and (b); (c) transverse section of a post-injury cavitation following immunohistochemical reaction for glial fibrillary acidic protein (green) (12 μm section). Scale bar: 500 μm.
3 Concepts Underlying Use of Veterinary Patients in SCI Research
The study of SCI in the laboratory has identified numerous interventions that are successful in improving functional outcome in experimental rodents, but there is now a pressing need to determine how best to translate successful strategies into clinically applicable treatments for human patients. Because of the factors outlined above, spontaneous SCI in domestic dogs constitutes an excellent intermediate model for this purpose.
To illustrate this specific point, use of dogs as experimental models for proof-of-principle experiments is rarely of great value, since there is little additional information that can be obtained over that available from rodent experiments, which are cheaper, more tightly controllable and ethically less complicated. Instead, domestic dogs that sustain naturally occurring SCI in the same way as humans provide excellent models for translational research, because they mirror so well the clinical presentation in human patients.
Proof-of-principle laboratory studies on experimental animals rely on establishing homogenous injuries within a homogenous population in which the effect of an intervention can be most readily observed. For instance, typically, the nature of the injury will be stereotypical (e.g., computer controlled impact device) in a tightly controlled sample of rats of the same weight, gender and genetic background. This implies a poor degree of “generalizability” of the investigations to other situations including, most importantly, human patient populations. In contrast, but in common with human patients, domestic dogs with SCI have natural injuries, in which the precise nature of the injury varies between individuals (e.g., varying components of contusion and compression, resulting from fractures, luxations, disk extrusions), there is a variable genetic background and there will be varying degrees of functional loss even within defined patient sub-groups. Further, rodent models of SCI also tend to minimize anatomical disruption of the spinal cord and surrounding structures, which favors axonal regrowth following an intervention (35), whereas structural integrity is frequently compromised in clinical injuries to dogs or humans. Similarly, dogs that sustain SCI because of a road traffic accident will, like human patients, present as poly-traumatized cases—a complicating factor that is never reproduced in experimental animals. It is likely that poor perfusion and oxygenation of the spinal cord as a result of multiple organ damage will exacerbate histologic injury and be detrimental to recovery.
Veterinarians are increasingly aware of the possibilities for translating laboratory research into the veterinary clinic and dog owners not only demand state-of-the-art treatment for their pets but also are well informed (via the internet) about possible novel treatments. These factors now have conspired to produce a large pool of domestic dogs that have severe SCI, in which the lesion can be well characterized using state-of-the-art imaging technology, and could form part of multicenter trials.
In summary, the central concept is that pet dogs form a “surrogate human” population of clinical patients in which to test the efficacy of potential treatments for SCI. Specific advantages of using naturally occurring SCI in domestic dogs as a model for investigating interventions are: (1) patterns of SCI are very similar to those in human patients; (2) diagnosis and patient categorization are made using the same tools; (3) similar methods can be used for functional assessment; (4) there is a large pool of chronically injured patients in both species; and, (5) domestic dogs and humans share a similar environment and can receive similar methods of rehabilitation.
4 Advantages of this Model for Exploring Specific Aspects of Spinal Cord Injury
4.1 Study of Treatment “Dose” in Dogs
The translation of therapies from laboratory animals to human patients necessitates defining the dose of a given treatment required to obtain the expected effect in a different, much larger, species. Defining the dose may be a problem in itself although may be done by simply scaling up the rat dose for the body weight, or body surface area, of a human patient (which may be an adequate approach for drug administration). Another method, which may be a better approach for cell therapies, would be to scale up the dose for the size of the spinal cord or by correcting the dose for the volume of the lesion measured by magnetic resonance imaging (MRI) (Fig. 2) (36). For example, the normal spinal cord diameter of Dachshund dogs in the caudal thoracic region (T9) is 4.0 ± 0.5 mm and 3.0 ± 0.2 mm at a lesion site (T12, T13 or L1) 3 months after an injury (n = 10 dogs with a mean body weight of 6.2 ± 1.8 kg; unpublished data; measurement from MR images). In comparison, the rat normal spinal cord diameter measured on MRI varies between 1 and 3 mm (36), and the gross human spinal cord diameter is around 7 mm in the caudal thoracic region (37).
However, because human or dog spinal cord lesions are longer and wider than lesions obtained in laboratory animals, not only the number of cells but the migratory properties of the cells tested, as well as the optimal method of delivery of the cells to populate the entire lesion must also be considered. The key difference between cell and drug therapies in this regard is that, although the calculated number of cells may be appropriate, it will also be critical that the cells are able to arrive at a lesion site within a specified amount of time (i.e., when the environment is responsive to their effects). The size of a lesion may present an obstacle in this respect because the time that is required for a cell to migrate through a large lesion may delay it sufficiently to make it arrive too late to be of benefit. Therefore, the dog forms a good model in which treatment doses and timing could be tested for their flexibility in development toward human use, since spinal cord lesions are larger than those in rats.
4.2 Histopathological Study of the Spinal Cord after Intervention
The availability of human spinal cord pathological specimens is very limited and when available, poor tissue preservation due to delayed fixation can limit acquisition of high quality data. Dogs have a shorter lifespan that humans, and may live only between a few months to several years following SCI and so are more commonly available for post mortem examination including, in some cases, tissue prepared by perfusion-fixation. Owners of dogs that have taken part in exploratory studies on novel therapeutic interventions are highly motivated by the research and commonly allow post mortem examination.
4.3 Environmental Factors
Recovery following a SCI is highly influenced by the activities of the patient, either performed autonomously (if still possible) or via a rehabilitation program. For example, treadmill training can have a major impact on plasticity of the intrinsic spinal cord circuitry in humans, cats and rats (38). It has also recently been found that behaviors can compete. For example, environmental enrichment in rats (which favors non-specific “self-training”) can cancel the benefits obtained by combining an intervention with specific task training (39). Following SCI, domestic dogs are treated in directly comparable environments as human patients and may also benefit from rehabilitation, such as physiotherapy and hydrotherapy, in which dogs engage very readily. Thus, domestic dogs could also be used to investigate how best to integrate medical interventions and rehabilitation programs.
5 Practicalities of Studying SCI Interventions in Dogs
5.1 Lesion Types
All types of lesion found in humans, including pure contusion, mixed contusion-compression, chronic compression, dynamic compression and vascular lesions have been recognized in dogs, and are readily identifiable on advanced imaging studies. However, penetrating trauma, such as that resulting from knife or gunshot wounds, is uncommon.
Domestic dogs are therefore available for modeling many different aspects of SCI although, as in humans, the most common clinical lesion results from acute mixed compressive and contusive events, such as that associated with fracture-luxation or intervertebral disk nuclear extrusion. This sub-population of dog patients is large, because the incidence rate of nuclear IVD extrusion is very high, especially within specific sub-populations.
5.2 Lesion Sites
SCI can occur at any site in dogs, but is most common adjacent to the thoracolumbar junction (TLJ), probably because of the changing nature of the mechanics and stabilizing influences within this region (19). In dogs, the TLJ contains the spinal cord (which extends into L5 vertebra) and therefore injuries at this level model well the effect of spinal cord lesions in the thoracic part of the human vertebral column. Effects of the lesion on brain control of the distal extremities, including bladder and limbs, can therefore be examined, with the exception of the control of sexual function (which would be difficult to study in dogs).
5.3 Survival Periods and Complications
The shorter general lifespan of dogs implies that canine veterinary patients will become available for post mortem studies much sooner after an intervention than their human counterparts. Nevertheless, dog SCI patients will usually survive many years after their initial injury, providing an opportunity to study the effects of an intervention over a longer follow-up period than is possible in rodents. On the other hand, dogs that have become paraplegic following SCI are susceptible to the same secondary problems as human paraplegics, such as ascending urinary tract infections, providing another opportunity for assessment of the impact of interventions in prehuman clinical trials.
5.4 Monitoring Function After Injury
Routine methods of clinical analysis and classification of function in canine SCI patients are very similar to those available for human patients, relying on simple, long-established, scoring systems. However, domestic dogs are highly amenable to sophisticated analysis of movement and the quadrupedal gait can be exploited to provide detailed quantifiable data on the coordination of limb movements between thoracic and pelvic limb girdles. It is particularly helpful in this respect that the most common site of injury in dogs is between T3 and L3 spinal cord segments (and by far most commonly between T12 and L2 (dog spinal cord has 13 thoracic and 7 lumbar segments)) and so lesions at that site disrupt communication between thoracic and pelvic girdles. The quadrupedal gait permits assessment of this coordination in a way that would not be feasible in human subjects and allows precise quantification of deficits and the response to therapeutic interventions (see below).
5.5 Routes for Delivery of Therapeutic Agents
Any of the feasible approaches for drug delivery in human patients can be used in dogs, including intravenous, intrathecal or even intraparenchymal routes of delivery of both cells and drugs. There are regulations in most countries regarding the ethics of interventions in domestic dogs, but these are not so restrictive as to prevent clinical trials of novel therapies, similar to those that would be permitted in human patients, so long as there is adequately documented scientific justification for the intervention. Regulation of interventions in veterinary patients varies throughout the world and frequently differs from the regulation of experimental procedures carried out in laboratory animals. One obstacle in the UK is the specific prohibition of placebos in clinical trials. This can make it difficult to properly evaluate in a double-blinded study (i.e., when both veterinary investigator and owner are blinded) the implications and treatment efficacy of a novel intervention versus sham therapy. However, for severely injured animals there is (of course) no currently effective therapy, meaning that it is feasible to choose to compare two different plausible interventions based on data obtained in laboratory rodents. Furthermore, there are frequently patient groups that can be identified and act as reasonable, albeit imperfect, controls (for instance paraplegic dogs whose owners choose not to take part in a clinical treatment trial). The specific prohibition of placebos places obstacles on surgical interventions in particular, since it would be very difficult for an Ethical Committee to accept sham surgery for a paralyzed dog.
6 Outcome Measures
Until recently, a potential drawback to the use of domestic dogs in SCI research was the lack of well-defined outcome measures of the effect of an intervention. Such a limitation also applies to some degree to human patients, since routine clinical outcome scores are coarse estimates of function and subjective (40) and therefore may differ from 1 day to another despite a stable neurologic deficit. Therefore the recent development of outcome measure tools for canine patients (41, 42) suggests that it might well be possible to detect changes in function with greater subtlety than in human patients.
In general, the clinical scoring instruments that are used to measure the neurological deficits and the function in dogs with SCI are identical to those used for human patients, allowing direct translation of this type of outcome data from dogs to human patients.
6.1 Clinical Score
The severity of functional loss following SCI in dogs has been subject to clinical scoring since the 1950s, using Tarlov scales, or that implemented by Hoerlein (43). Recognizing the need for more precise tools, Olby and others (44) developed a scoring scheme similar to the BBB score used in rodents (7) for use in dogs. Although providing considerably more subtlety, the scoring scheme is not linear, which limits accurate and flexible statistical analysis over the range of possible scores. Furthermore, subjective scores remain susceptible to bias and are often incomplete because they do not take into account urinary and fecal incontinence; also, assessment of fine sensation, which is a component of similar schemes in human patients, is difficult in dogs.
6.2 Spinal Cord Imaging
Advanced imaging techniques of the spinal cord such as high field MRI and 16-slice computed tomography (CT) are increasingly available for veterinary patients (45), although dogs must be anesthetized during such examinations. These techniques allow detailed analysis of spinal cord integrity similar to that available in routine clinical practice in humans and can be used to recognize post-traumatic complications of SCI such as syringomyelia (46). Extended regions of hyperintensity on T2-weighted MRI scans are now known to be correlated with poor recovery (45, 47). Diffusion tensor imaging and other less routine MRI techniques, although not commonly used in dogs currently, could also be applied with access to the appropriate software (23).
6.3 Electrophysiology
Electrodiagnostic equipment used for dogs is identical to that used for human patients and similar recordings as can be made in human patients can be obtained from dog patients, providing information on integrity of impulse transmission through the damaged region of spinal cord (48–50). This includes:
–Transcranial magnetic motor evoked potentials: compound muscle action potentials recorded in limb muscles following magnetic stimulation of the head or spine. Motor evoked potentials are used to study long-distance descending motor pathways;
–Somatosensory evoked potentials: field or ascending potentials recorded over the vertebral column or skull following stimulation of a peripheral nerve, spinal cord (spinal-evoked potential) or skin (dermatomal somatosensory evoked potential). Somatosensory evoked potentials are used to study ascending sensory pathways;
–Late waves recorded from peripheral nerves, such as the Hoffman reflex (H-reflex), reflect motor neuron excitability following SCI (51) and can assess plasticity of the spinal cord. The H-reflex can be recorded in paraplegic dogs (Fig. 3).
Fig. 3.
H-wave recording in a dog following thoracolumbar spinal cord injury. The distal tibial nerve is stimulated and the recording obtained from the interosseous muscles; the H-wave amplitude is higher during sub-maximal stimulation of the tibial nerve (1, dashed line with double arrowheads) compared to supra-maximal stimulation (2, plain line double arrowheads); the presence of the H-wave demonstrates the integrity of the lumbar spinal cord below the lesion site; CMAP: compound muscle action potential corresponding to the direct interosseous muscle response; H-wave: late potential.
There is extensive veterinary experience with these techniques and somatosensory evoked potentials and spinal-evoked potentials have been shown to be clinically relevant to grade SCI in dogs (49, 52). Transcranial magnetic motor evoked potentials (TMMEPs) can be difficult to record in the acute phase of SCI in non-ambulatory dogs (53), but recent investigations have shown that the severity of conduction deficits following TMMEP in dogs with cervical spondylomyelopathy (a chronic compressive lesion of the cervical spinal cord) correlate with the severity of the clinical signs (54). Somatosensory evoked potentials have not been recorded in dogs with thoracolumbar disk herniation and loss of pain sensation caudal to the lesion, but measurements have been made in the acute phase of SCI (49). Recently, we have recorded SSEPs in the chronic phase of SCI in patients that have absent or equivocal pain perception in the hindquarters (41).
6.4 Urodynamic Testing
Similar to human patients with SCI (55), dogs with SCI will present neurogenic bladder dysfunction such as reflex voiding and detrusor over-reactivity complicated by vesico-sphincterial dysynergia, or detrusor atony (41). Urodynamic equipment used for analysis of human continence can be used in dogs (56) to obtain cystometric, urethral profilometric, and sphincter electromyogram data. This information is directly relevant to understanding possible effects of novel interventions in human patients—for instance, routine urodynamic assessments of function in human SCI patients (57, 58), such as bladder compliance, number of involuntary detrusor contractions, sphincter activity during bladder filling can readily be obtained to define the same aspects of continence in dogs with SCI.
6.5 Gait Analysis
Disruption of quadrupedal coordination after SCI provides an enticing opportunity for functional analysis in dogs. Following severe thoracolumbar SCI, there is complete loss of pelvic limb movement, but often “spinal walking”—defined as stepping by the pelvic limbs in the absence of clinically detectable spinal cord transmission across the lesion—can appear within a few months in dogs (59). A characteristic feature of “spinal walking” is the lack of coordination between thoracic and pelvic limb movements, and there is also a lack of pelvic limb lateral stability. Computerized gait analysis provides methods to quantify both these aspects of spinal walking, meaning that it is possible to attribute a numerical score to the changes in coordination that occur during either spontaneous or intervention-induced recovery (Fig. 4) (for a review see (41)). Using these methods we have shown that normal dogs have extremely rigid coordination between thoracic and pelvic limb motion, and also that this pattern is recovered following mild SCI (41, 60, 61). We are currently using these techniques to evaluate the outcome after intraspinal transplantation of olfactory ensheathing cells (OECs).
< div class='tao-gold-member'>
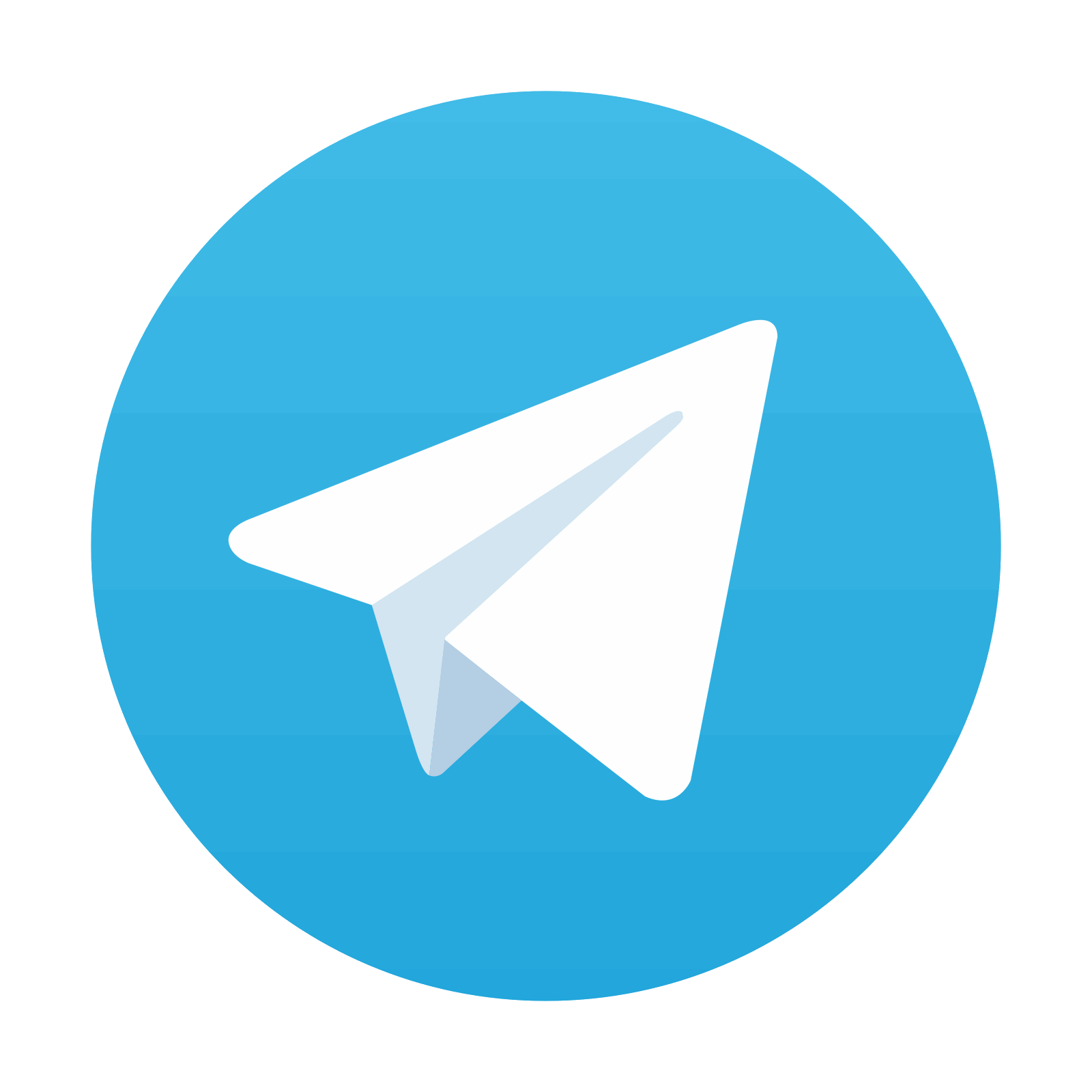
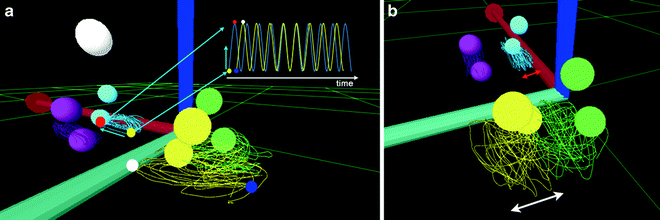
Only gold members can continue reading. Log In or Register a > to continue
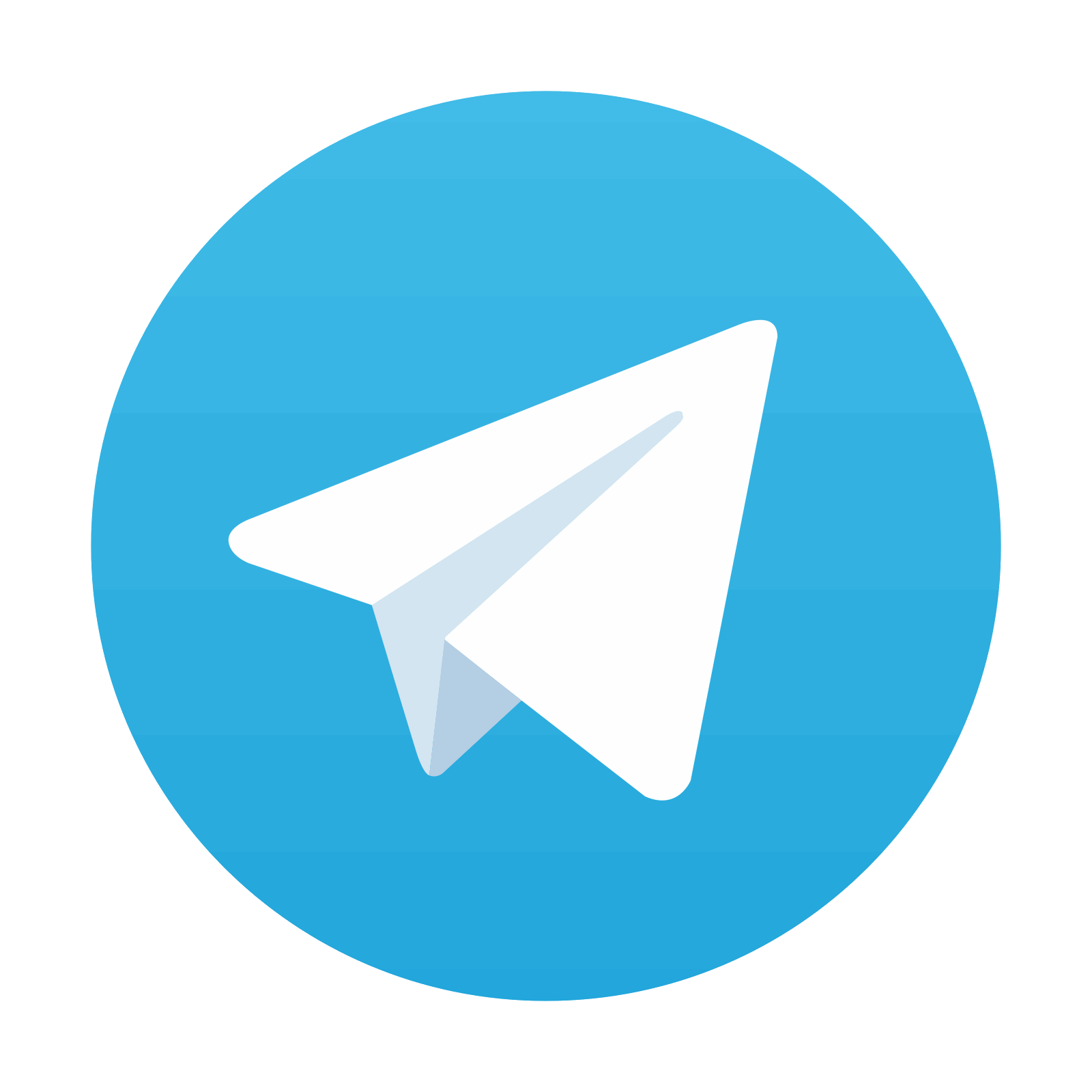
Stay updated, free articles. Join our Telegram channel

Full access? Get Clinical Tree
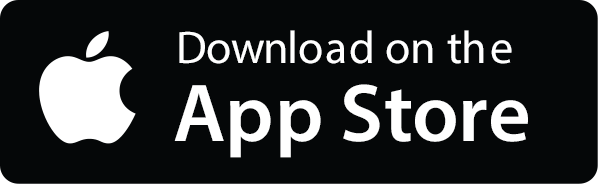
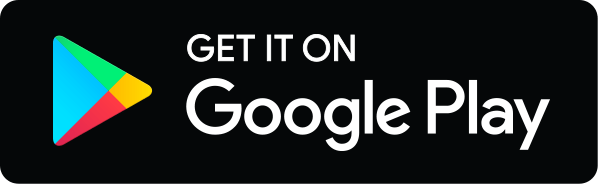