Advantages
Atherosclerosis develops over a period of months
Relative ease of genetic manipulation to allow for investigation of multiple cell types or proteins, including temporal or cell specific expression, on atherogenic process
Relatively short gestation time
Lower amounts of drugs/reagents needed due to small size
Limitations
Does not develop unstable plaques characteristic of human lesions
Does not readily develop coronary artery atherosclerosis
Develops significant atherosclerosis in the aortic sinus, which is not a common site of lesion formation in humans
Lipoprotein profile is not identical to humans: need to significantly increase apoB-containing lipoproteins and plasma cholesterol levels to develop atherosclerosis; does not express CETP; HDL subclass profiles are different
Amount of tissue obtainable for analysis is limited by small size
Immune system is not identical to humans
It is reassuring that mechanisms of atherogenesis appear to have much in common between humans and mice, including those driven by hemodynamic stresses. This said, the murine models and their analysis have important limitations (Table 1). First, the currently intrinsic limitation is that murine lesions seldom develop the instability characteristic of the advanced human lesions that account for much of clinically significant atherosclerotic cardiovascular disease. Also the precise distribution of lesions is not identical for murine and human lesions. Mice seldom develop atherosclerosis in the coronary artery, a major site of lesion development in humans. In addition, the aortic root is one of the earliest sites for the development of lesions in the mouse and is seldom involved in humans. This is likely due to the more rapid heartbeat in the mouse. The aortic root has been the most frequently analyzed site of lesion development, but fortunately investigators are examining now more than one arterial site and often at multiple experimental time points. This allows for a better understanding of the impact of the genetic manipulation or therapeutic treatment at different stages of plaque evolution and if there are site-specific effects. Mice also differ in lipoprotein metabolism, with wild-type mice predominantly accumulating cholesterol in HDL particles, not expressing cholesteryl ester transfer protein (CETP), and producing apoB48, the edited version of apoB100, in the liver as well as the intestine, unlike humans where only the intestine is involved in the editing of the apoB transcript.
The initial study of vascular lesions in rodents began more than 60 years ago with the feeding of cholesterol and cholate containing diets to rats [11]. However, the use of mice for such investigations really took off after Jan Breslow’s laboratory [12] and Nabuyo Maeda’s laboratory [13] independently described the apolipoprotein E (apoE) deficient mouse (Apoe−/−), which develops atherosclerosis even while being fed standard rodent chow diets. With maturation these lesions can be quite complex [14]. This model has been very widely used for the preclinical study of atherosclerosis. The other widely employed murine model is the LDL receptor deficient (Ldlr−/−) mouse, which is a murine model of human familial hypercholesterolemia [15]. Both of these models are characterized by hypercholesterolemia which is prerequisite for the development of atherosclerosis in murine models. However, these two models differ in significant ways from one another as will be apparent from what follows.
2.1 The ApoE Deficient Model
ApoE is carried on plasma lipoproteins, mostly chylomicron remnants, VLDL and large HDL. It serves as a ligand for the hepatic uptake of non-HDL lipoproteins by members of the LDL receptor superfamily of receptors. The lipoprotein phenotype of Apoe−/− mice consists of a predominance of remnant lipoproteins that are enriched in cholesteryl ester and apoB48. Though atherosclerosis develops when Apoe−/− mice are fed a standard low fat chow diet, the development can be accelerated by feeding the fat and cholesterol enriched Western type diet (WTD). Lesions develop at the aortic root, the aortic arch, the innominate artery, the carotid arteries and other arterial sites depending on the diet and the duration of feeding [14]. Note that in this model there is little or no coronary artery lesion. However, the combination of apoE and scavenger receptor BI deficiencies leads to a model having profound coronary lesions with lumenal obstruction [16].
Although the presence of hyperlipidemia is a prerequisite for atherogenesis in mice, the modern concept of atherogenesis focuses on inflammation as the other major component of its pathogenesis. Bone marrow transplantation is a technique often used to assess the involvement of specific cells or cell products of the immune system in atherogenesis. Fixed tissue macrophages e.g., Kupffer cells are not completely eliminated by irradiation that destroys the cells of the bone marrow of the recipient mouse, but this can be effected by treatment with clodronate liposomes following reconstitution of the bone marrow in the recipient mice [17]. However, there is a limitation to using bone marrow transplantation studies in Apoe−/− mice. The clearance of chylomicron remnants is quite sensitive to the concentration of apoE in the plasma, with plasma levels ~10 % of normal being sufficient to effect virtually normal lipoprotein clearance [18]. While the hepatocyte is the major site of synthesis of apoE, it is also produced by macrophages, as well as steroidogenic cells, astrocytes and adipocytes [19]. The complementation of hyperlipidemic Apoe−/− mice with bone marrow from wild type mice largely rescues the hyperlipidemic and atherosclerosis phenotype, even though the plasma concentration of apoE barely reaches 10 % of normal levels [20]. The reduction in plasma lipids by macrophage-derived apoE likely contributes to the reduced atherosclerosis. However, apoE has a number of non-lipoprotein metabolism related functions as well that likely influence atherosclerosis, including promoting lipid efflux from macrophages and anti-inflammatory actions [19]. Studies involving the transplantation of bone marrow from Apoe−/− mice [21, 22] or from apoE expressing mice under conditions that did not alter plasma lipid levels [23, 24] demonstrate a plasma lipid-independent effect of apoE expressed by bone marrow-derived cells, primarily macrophages, on atherosclerosis. Thus, its local presence within the lesion in the artery wall also contributes to atherosclerosis phenotype. As a result, the bone marrow transplantation approach to the study of cells and genes of the immune system on atherosclerosis is somewhat limited if using Apoe−/− recipient mice by the need to use bone marrow donors that are themselves apoE deficient. However, not all sources of apoE are equally effective in rescuing the hyperlipidemic atherosclerosis phenotype of Apoe−/− mice. Though apoE expressed by adipose tissue transplants results in a plasma apoE concentration similar to that induced by bone marrow transplantation, it does not improve either the hyperlipidemia or the atherosclerosis of the Apoe−/− host [25]. The basis for this distinction is not clear.
2.2 The LDL Receptor Deficient Mouse
The LDL receptor is a widely expressed plasma membrane protein that is responsible for the clearance of plasma lipoproteins containing either apoB100 or apoE. From the pioneering and continuing work of Brown, Goldstein and their colleagues in Dallas, we know that the major function of this receptor is to mediate the hepatic clearance of these lipoproteins and modulation of receptor biogenesis is a major indirect target of the statin group of drugs used to control hyperlipidemia in patients at risk of developing atherosclerotic heart disease. Since the hepatic LDL receptor is primarily responsible for the uptake and clearance of apoB/apoE -containing lipoproteins, the absence of the hepatic LDL receptor is mostly responsible for the accumulation of apoB100 containing LDL particles in the Ldlr−/− mice fed standard chow diet. But the liver has other uptake pathways for VLDL and remnants, which accounts for the modest hypercholesterolemia and small lesions that develop only in aged Ldlr−/− mouse upon feeding chow diet [26]. Therefore, this model is most often employed with the feeding of a high-fat, high-cholesterol diet, leading to an accumulation of VLDL remnants as well as LDL. In contrast to the Apoe−/− mouse, the VLDL of the Ldlr−/− mouse is richer in triglyceride and apoB100. Lesions in Ldlr−/− mice develop in each of the vascular sites where lesions are seen in the Apoe−/− model, within a time frame exceeding at least 6 weeks of high-fat diet feeding.
Although the WTD is most widely employed atherogenic diet, a variety of high-fat diets have been used [27]. This includes diets containing quite high cholesterol content [28] or others that induce obesity and diabetes, such as the high-fat, high-sucrose, cholesterol-supplemented diet [29], which may induce even larger lesions than does the WTD [30]. There are also diets in which the composition of the dietary fat is varied. The WTD is usually associated with the presence of insulin resistance, which can be avoided by feeding a diet enriched in cholesterol with low fat [31].
The Ldlr−/− mouse is much more useful for bone marrow transplantation studies, as the LDL receptor expressed on bone marrow derived cells is not critical for the clearance of apoB containing lipoproteins [32]. Thus, in contrast to the situation with the Apoe−/− model, the donor bone marrow can express the LDL receptor without significantly influencing atherosclerosis burden in the chimeric mouse.
2.3 Other Murine Models of Atherosclerosis
In addition to Apoe−/− mice, several models in which variants of apoE are expressed have also been used in atherosclerosis research. In humans there are three major apoE isoforms, designated apoE2, apoE3, and apoE4. These different isoforms can profoundly modify the metabolic phenotype of the individual. A series of targeted gene replacement mice in which the mouse apoE gene was replaced with one of the human apoE isoforms have been engineered [33], allowing for the exploration of the mechanisms by which these isoforms may modify arterial vessel functions that impact on atherogenesis. While mice expressing APOE3 and APOE4 genes are relatively protected from development of atherosclerosis, APOE2 knock-in mice spontaneously develop atherosclerosis. Likewise, transgenic mice expressing the APOE*3–Leiden variant, which has low affinity for the LDL receptor, develop atherosclerosis when fed a high-fat diet and have been used by several groups [34].
There are a number of variants of the Ldlr−/− model that will develop atherosclerosis while being fed standard chow. These include apoB100 transgenic/Ldlr−/− mice [35] and Apobec−/−Ldlr−/− mice [36]. This latter model lacks the enzyme responsible for the editing of the apoB100 mRNA transcript to yield apoB48 and hence the apoB expressed in these mutant mice is exclusively the apoB100 variant. Young and colleagues have also developed apoB100 only mice by mutating the codon in the mouse apoB gene that is edited to generate apoB48. These mice were crossed with Ldlr−/− or Apoe−/− mice to explore the role of plasma lipoprotein size on atherogenesis [37]. These studies were partly informed by the finding that cholesterol fed rabbits had more atherosclerosis than similar fed rabbits that were diabetic [38]. The diabetic rabbits had more chylomicron cholesterol, i.e., larger particles, which were thought to have more limited access to the arterial intima [39]. When Young and colleagues compared the two apoB100 only models fed standard chow at similar plasma cholesterol levels, they found that the Apob100 only, Ldlr−/− mice, whose plasma cholesterol was carried primarily in LDL particles, had much more lesion than the Apob100 only, Apoe−/− mice which had most of their plasma cholesterol in larger remnant particles. Thus, a large number of small apoB-containing particles (i.e., LDL) were more atherogenic than a smaller number of large particles (i.e., VLDL/chylomicron remnants). However, a model in which chylomicrons are very poorly lipolyzed and accumulate in the plasma (due to deficiency of glycosyl phosphatidyl inositol anchoring HDL binding protein required for binding of lipoprotein lipase to the capillary wall) indicates that large chylomicrons may be atherogenic albeit at a much slower rate and extent than for elevated LDL [40]. As mentioned above, mice lack CETP, which facilitates the exchange of lipids between HDL and apoB-containing lipoproteins leading to a reduction in HDL levels. Mice transgenic for human CETP expressed in the Ldlr−/− mouse [41] and Apob100 transgenic mouse [42] as well as in Apoe−/− [41] and APOE*3–Leiden [43] mice have been used to examine the impact of CETP expression on atherogenesis.
3 Immune Cells in Atherogenesis
The macrophage is the most abundant cell type of the atherosclerotic plaque, especially in the early plaque which is predominantly a foam cell lesion. The importance of macrophages was highlighted by the profound reduction in lesion growth in the absence of M-CSF [44]. Monocytes in the blood are the source of lesional macrophages and we now know that monocytes and macrophages are heterogeneous [4, 45]. In murine blood, the monocytes are divided into two major subpopulations, the inflammatory Ly6Chi cells and patrolling Ly6Clo monocytes. Hypercholesterolemia in Apoe−/− mice increased the level of Ly6Chi monocytes [46]. This subpopulation is also the major monocyte population that infiltrates the atherosclerotic lesion [46, 47]. Within the atherosclerotic lesions, signals within the microenvironment promote the polarization of macrophages to M1-like inflammatory macrophages and alternatively activated M2-like less inflammatory macrophages, although macrophages with other phenotypes are also observed [4, 48]. The M1-like macrophages are the predominant cells in developing atherosclerotic plaques and are likely derived from infiltrating Ly6Chi monocytes and conversion of M2-like macrophages in response to signals within the lesion. Activated inflammatory macrophages in the plaques are one source of pro-inflammatory cytokines, such as IFNγ, and chemokines such as MCP-1. The M2-like cells probably participate in healing that occurs within chronic plaques and are enriched in plaques undergoing regression [49].
Recently there has been much evidence showing that mouse models of atherosclerosis, particularly Apoe−/− mice fed a WTD, have monocytosis [50]. Indeed in humans monocytosis constitutes a risk factor for atherosclerotic heart disease [51]. Among the regulators of monocytosis in the setting of atherosclerosis is the stimulation of growth factor-mediated proliferation in the hematopoietic stem and multiple progenitor cells (HSPC) in the bone marrow in response to dysregulated cholesterol homeostasis [52]. The mechanism appears to involve an increase in plasma membrane cholesterol leading to increased lipid rafts and the concentration of growth factor receptors, particularly IL-3/GM-CSF receptors which share a common subunit. This occurs, for example, in HSPC of cholesterol efflux deficient Abca1−/− Abcg1−/− mice. Thus, the cells are more responsive to cell proliferation in the presence of IL-3 and GM-CSF. The enhanced proliferation of these cells is attenuated by HDL [53]. In addition, monocytes may be mobilized from the spleen where extramedullary hematopoiesis may add to the numbers of these cells in circulation, i.e., the spleen may function as a reservoir from which cells are mobilized into the plaques [54].
Neutrophils have been recently implicated in the progression of human and murine atherosclerosis as well as contributing to plaque instability in humans [55]. Apoe−/− mice fed WTD also have a neutrophilia [50]. This effect on neutrophil levels involves extramedullary hematopoiesis and the IL-23/IL-17/G-CSF signaling axis [52].
Many cells of the adaptive immune system are also found in the plaque and influence its evolution [9, 56]. In the complete absence of mature T and B cells, as for example in the recombination activation gene deficient animals (Rag−/−), atherogenesis in the aortic root is reduced, but this is not observed when animals are fed a WTD for an extended period [57–59]. As a first approximation this argues that cells of the adaptive immune system are not required for the development of robust atherosclerosis. The immune cells may be either pro-inflammatory or anti-inflammatory. The fact that immune deficient mouse models generally exhibit either smaller or similar sized lesions suggests that their predominant effect is pro-inflammatory, although other effects may occur under certain circumstances. For example, in the chow fed Apoe−/−Rag−/− model the innominate artery atherosclerosis is actually increased (unpublished data), arguing that in this context the predominant cell removed is anti-inflammatory.
Among B cells there are several subsets; B-2 cells, B-1 cells, and B regulatory cells. B-2 cells are the primary antibody producing cells and they also are capable of presenting antigen. Their role in atherosclerosis is primarily proatherogenic as evidenced by the effect of their removal or interruption of their signaling in murine models of atherosclerosis [60, 61]. On the other hand, the B-1 cells of the innate immune system produce atheroprotective natural IgM antibodies, such as those targeting oxidized phospholipids [5, 62]. A number of investigators have immunized atherosclerosis susceptible murine models with relevant antigens (e.g., apoB peptides) with resulting attenuation of atherosclerosis [63].
T cells also have the potential to contribute various influences on atherogenesis. There are many subsets of T cells, among which are Th1 cells, Th2 cells, Th17 cells, T regulatory cells and natural killer T (NKT) cells. The Th1 cells generally are proatherogenic, by functioning as helper cells or producing proinflammatory cytokines, such as IFNγ. Their adoptive transfer into immune deficient atherosclerosis susceptible hosts promotes lesion development [64]. The role of Th2 cells is less clear, although they appear to be antiatherogenic. Similarly, Th17 cells have been shown to be pro-atherogenic and antiatherogenic in different studies in mouse models [65]. T regulatory cells are notably antiatherogenic, predominantly by secreting anti-inflammatory cytokines, of which IL-10 and TGFβ are most prominent and most studied [66]. NKT cells are of particular interest with respect to atherosclerosis in that they recognize lipid antigens presented by CD1 molecules on antigen presenting cells. Although they come in at least two varieties, invariant or group I NKT cells that express relatively invariant T cell receptors and group II NKT cells with more diverse T cell receptors, both groups of cells are CD1d restricted in the mouse [67]. The group I NKT cells are most prominent, particularly in the liver, and they have been most studied. They are proatherogenic as demonstrated in several ways, including their adoptive transfer into immune deficient Ldlr−/− recipients [68]. The type II NKT cells are much less abundant and their role in atherogenesis is much less clear. Their direct study is not easy for lack of specific targetable markers.
The stimulation of the adaptive immune system requires two signals; an antigen signal and a second signal involving a variety of interacting co-stimulatory molecules on T cells and antigen presenting cells. The role of many of these costimulatory molecules have been studied in murine atherogenesis models [69]. For the most part, these molecules are pro-atherogenic, consistent with the pro-atherogenic role of T and B cells.
4 Genetics of Murine Atherogenesis
Much of the work reviewed above has been carried out in a single strain of mice: the C57BL/6 strain which is the most atherosusceptible strain. The focus has been primarily on the examination of the effect of overexpression or deletion of a large number of genes on atherogenesis. In a recent review, the effect of the deletion of about 100 genes on atherosclerosis in C57BL/6 mice, mostly in the Apoe or Ldlr deficient backgrounds, has been surveyed [70]. About a third of these genes affect plasma lipids, which may be mediating the role of that gene product in atherosclerosis. A less biased approach to the identification of genes that impact on atherosclerosis has involved genetic crosses between the atherosusceptible C57BL/6 mice and one or other resistant strains, e.g., FVB, again frequently in the Apoe or Ldlr deficient background. This has resulted in the identification of a number of loci (>40) having an impact on lesion development that are mapped to a variety of chromosomes. This emphasizes the complexity of genetic influences on atherogenesis. It should be noted that in most cases, each putative locus contains multiple neighboring genes. In very few cases has a particular gene been identified by this approach. However, recently the subcongenic analysis of the Ath11 locus in a cross between C57BL/6 Apoe−/− and FVB Apoe−/− mice has identified a single gene, Raet1e encoding a major histocompatibility class 1-like molecule, whose expression level regulates aortic root atherosclerosis [71]. This conclusion was confirmed by the targeted overexpression of one of the alleles of this gene, resulting in the predicted atherosclerosis phenotype. A particularly interesting outcome of the comparison of mouse strains involved the study of 129S6/SvEvTac Apoe−/− strain which exhibited a surprising increase in aortic arch atherosclerosis relative to C57BL/6 Apoe−/− mice. The aortic root in the 129S6/SvEvTac strain is more atheroresistant, but this strain has a sharper angle of curvature for the arch and this appears to induce a difference in shear stress at this vascular site resulting in a hemodynamic environment that promotes lesion initiation and growth [72]. The development of large panels of recombinant inbred strains offers the potential for the dissection of high-resolution genetic control of various lipid and atherosclerosis phenotypes [73].
5 Regression of Atherosclerosis
It is important to appreciate that development of the atherosclerotic plaque is a highly dynamic chronic inflammatory process. The susceptibility of plaques to regression is a very good indication of this. There are several examples of the regression of established murine lesions [74]. The clearest indication of plaque regression is seen in the transplantation of an atherosclerotic aortic arch into a naïve non-atherosclerotic, normolipidemic host. In this model, both early and advanced lesions regress [75, 76] and this regression is enhanced by the overexpression of apoA-I, suggesting that apoA-I/HDL mediated cholesterol efflux may be an important mechanism promoting regression [77]. Indeed, the induction of regression following agonism of miR-33 which leads to increased expression of the ABCA1 transporter involved in macrophage cholesterol efflux and hepatic biogenesis of HDL [78] or treatment of mice with LXR agonists [79] that also promote expression of ABCA1 along with ABCG1 and apoE support the concept that enhancement of macrophage cholesterol efflux promotes atherosclerosis regression. Given that hypercholesterolemia plays such a critical role in lesion development, the substantial reduction of plasma cholesterol level likely contributed to the lesion reduction in the transplant model. Plasma cholesterol reduction can be accomplished by switching from a high-fat to low-fat diet, thus reducing apoB-containing lipoproteins. Another mechanism to reduce the secretion of apoB-containing lipoproteins is achieved by targeting microsomal triglyceride transfer protein (MTP), a protein involved in the lipidation of apoB. The conditional attenuation of the Mtp gene expression in the Apob100 only/Ldlr−/− model or the inhibition of MTP activity in Ldlr−/− mice along with a return to a standard chow diet represents an effective strategy to achieve regression [80, 81]. Similarly, the conditional induction of apoE expression in the hypomorphic Apoe/Mx1–Cre model also promotes regression of lesions in high-fat diet fed mice [82]. During lesion regression associated with lowering the cholesterol burden, macrophage cells alter their phenotype and are thought to emigrate from the lesion to the draining lymph nodes [75]. This process is enhanced by the increased expression of the chemokine receptor CCR7 [83], now recognized as a cholesterol responsive gene [84]. Murine models of atherosclerosis are also being used to demonstrate that immune cells other that macrophages, such as T cells and T regulatory cells [85, 86], can also participate in lesion regression. Thus, cells of the immune system participate in many aspects of the atherogenic process.
6 Conclusions
The study of murine models of atherogenesis over the last two decades has been extremely valuable in revealing the mechanisms that are operative in initiating lesion development as well as their progression and regression. As lesion development occurs over an extended time, it is likely that the dominance of pathways may vary over the evolution of the plaque, which adds to the requirement for examination of the effect of genetic manipulation at different stages of lesion development. Murine atherosclerotic models are also valuable for the dissection of mechanisms operative in response to potential therapeutic interventions. It is clear from the information so far acquired that there are many genes and processes implicated in the pathogenesis of atherosclerosis, involving a great deal of cross talk between cells and their gene products, and this in turn may change with lesion maturation. With the rapid advance in gene sequencing and analysis technology as well as associated data processing one can expect even more appreciation of these mechanisms, involving not only single genetic targets but system analysis [87]. The more frequent employment of conditional gene deletions such as the Cre-Lox system and inducible expression systems to temporally turn on or off gene expression, will add greatly to our understanding of the mechanisms involved. While studies in mice have and will continue to provide insight into the mechanisms of the pathogenesis of atherosclerosis, it needs to be kept in mind that there are limitations to the use of mouse models, particularly that the arterial sites where lesions develop are not identical to humans, that mice do not develop unstable plaques characteristic of human lesions, and that significant hypercholesterolemia is needed to induce atherosclerosis in these models.
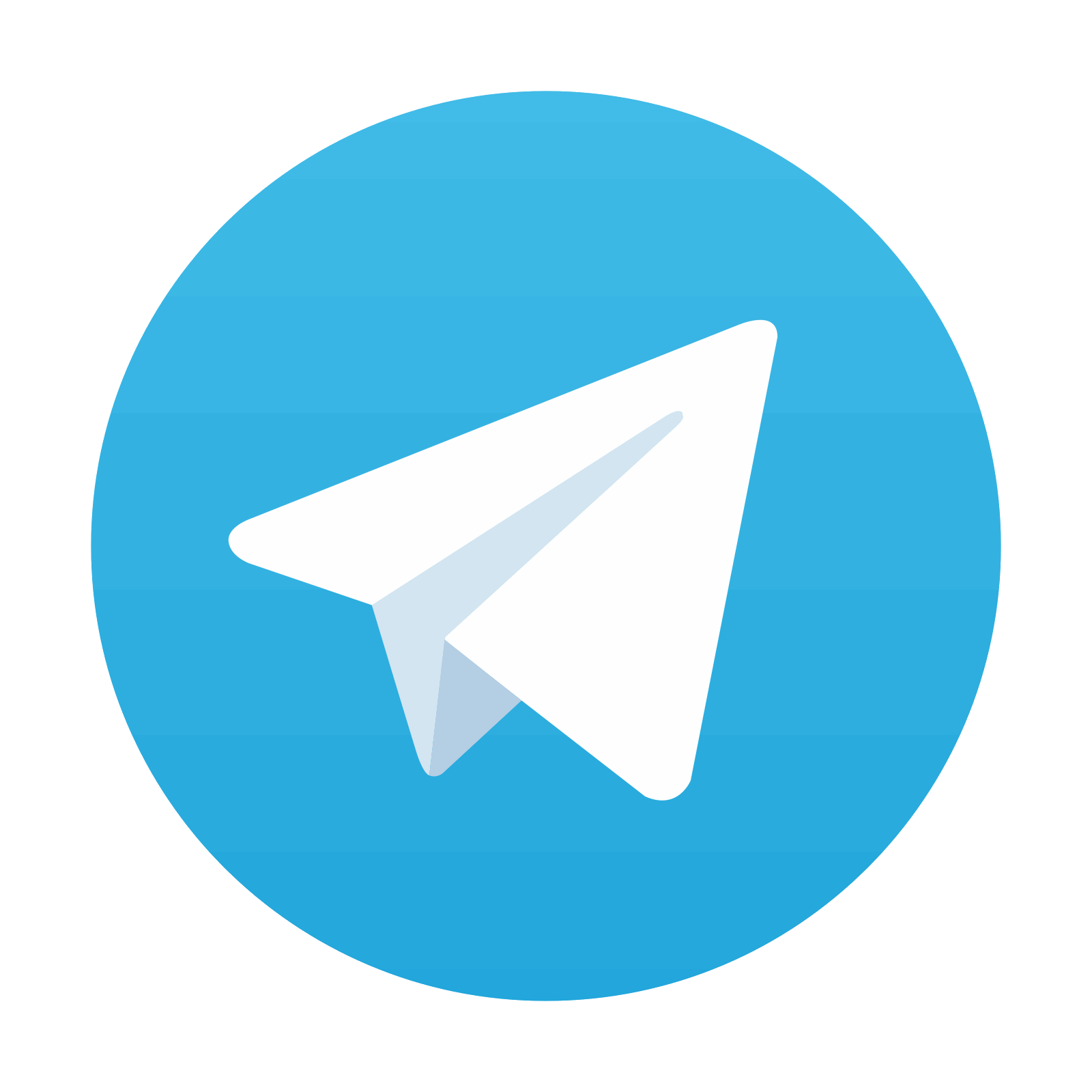
Stay updated, free articles. Join our Telegram channel

Full access? Get Clinical Tree
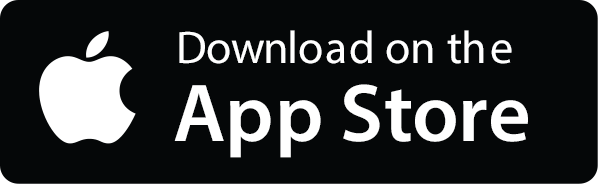
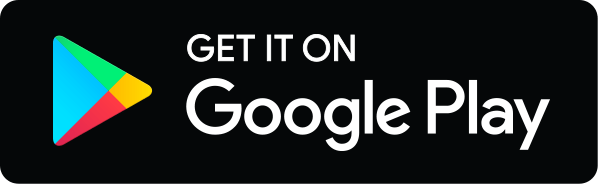