19 Robert J. Higgins,1 Andrew W. Bollen,2 Peter J. Dickinson,1 and Sílvia Sisó-Llonch3 1University of California, Davis, USA 2University of California, San Francisco, USA 3BioMarin Pharmaceutical Inc, USA Primary neoplasms of the nervous system are relatively rare in most domestic animal species with the exception of the dog and, to a lesser extent, the cat. Dogs and cats live mostly in close proximity to the families of their owners and are generally permitted to live their whole life span, reaching an age when both central (CNS) and peripheral nervous system (PNS) tumors become most prevalent. Based on the few published collections and our own experience, about 75% of all nervous system neoplasms have been recognized in dogs and 15% in cats, with the remainder in all other animal species combined. Neoplasia of the nervous system in domestic animals is primarily a problem of aging animals; over about 95% of primary tumors of the canine CNS occur in dogs over 5 years of age. The incidence of CNS tumors in aged dogs is similar to that in the adult human population (incidence is defined as the percentage of all new cases in a specific time compared with the total population at risk). Overall all human CNS tumors have an incidence of 20.59 cases/100,000, whereas neuroepithelial, meningeal, and pituitary tumors account for 17/100,000.1 Limited dog data based on the Alameda and Contra Costa neoplasm registry (1963–1973) indicates an overall incidence of 14.5 cases/100,000.2 In a recent study of 9574 dogs there was an incidence of 4.5% at necropsy, comprising both primary and secondary intracranial tumors.2–4 Based on necropsy data, the incidence of adult canine brain tumors is 2–4.5% of all deaths where a necropsy was performed, compared with a human incidence of 2.1%.1,3–5 However, there is a significant difference in the incidence of human pediatric CNS tumors (children up to 15 years of age) compared with that in dogs. Brain and other CNS tumors constitute 21% of all childhood cancers, second only to that of leukemias, whereas only 10% of all canine CNS tumors occur in dogs under 3 years of age.1,3,5 Neuroblastoma comprises about 7% of all human pediatric tumors, which is again a striking difference in incidence from canine tumors.1,4,6 The majority of all canine primary and secondary intracranial tumors occur in dogs more than 5 years of age.7 There are some differences in the incidence of various animal tumor types both between and within species, and also among breeds. The frequency of intracranial neoplasia in the cat is about 2%, with a relatively high incidence (40%) of meningiomas.7 In the canine species, over 50% of all gliomas occur surprisingly in certain specific brachycephalic breeds (e.g., boxers, Boston terriers, English and French bulldogs, and English toy spaniels), although these breeds represent only about 6% of an institute’s referral population.2,4,6,7 This predilection of gliomas has been associated with a susceptibility locus on canine chromosome 26.7,8 In our institute, within this breed‐restricted population, 50% of all oligodendrogliomas occur in boxers, bulldogs, and Boston terriers, whereas only the boxer and Boston terrier have an increased risk of astrocytomas. Meningiomas have a statistically higher incidence in both golden retrievers and boxers, whereas choroid plexus tumors and lymphomas are overrepresented in the golden retriever and rottweiler breeds, respectively.7 An accurate classification scheme for the animal nervous system tumors provides an essential link between pathologists and clinicians as well as between veterinary medical and human medical specialists in the various branches of clinical and investigative neuroscience. The current classification and grading system is based on histological and cytological criteria capable of determining the origin of the tumor cell type, the cell tumor growth biology, and the degree of differentiation.9 Such systems are designed to provide predictive data critical for both subsequent prognostic outcome and informed therapeutic decisions. Attempts to establish classification schemes of human nervous system neoplasms started in the early part of the twentieth century. These systems first established the histogenic cell of origin as the basic concept for the current classification system. However, diverse opinions by leading neuropathologists from different schools and countries led to many controversies and created a need to establish a common language for defining and accepting a common classification system. In response to this need, the World Health Organization (WHO) initiated the formation of international committees of neuropathologists to create an internationally acceptable classification of tumors based on the most advanced methods available.9,10 Since that time a rapid development in cytogenetic analysis, electron microscopy, immunohistochemistry (IHC), molecular biology, and genetics has resulted in a most accurate and prognostically reliable classification of human brain tumors. Although there remain a number of different schemes, the most accepted one is the 2007 WHO Classification of Tumours of the Central Nervous System.9 Because of the demonstrated close similarities between human and canine brain tumors, and because the veterinary WHO classification is hopelessly outdated, we have elected to apply the 2007 WHO human tumor classification and grading system for all tumors in this chapter. Subsequent to 2007, however, there have been many advances in the molecular genetics and biology which will be integrated in the next edition of the WHO classification. Nevertheless, we do recognize the need for urgent prospective studies with complete follow‐up data from necropsies to confirm such prognostic accuracy, particularly for canine tumors and for integration with molecular studies. The histological grades vary from I to IV across tumor entities, with grade I having low proliferative potential and a possibility of successful surgical resection, whereas at the other end of the spectrum are grade IV tumors, assigned as cytologically malignant, mitotically active, with necrosis, and with rapid progression towards a fatal outcome without any attempts at treatment. Different tumor variants are graded based on specific histological criteria. For example, glioblastomas (changed from glioblastoma multiforme) are grade IV astrocytomas that have exclusively either necrosis with peripheral pseudo‐palisading and/or microvascular proliferation.9,10 It is important to understand that a provisional tumor diagnosis depends on many factors, including the age, breed, and neuroanatomical location, together with neuroimaging findings (e.g., magnetic resonance imaging).11–13 Despite algorithms that are now available as guides towards establishing a provisional diagnosis based on these various criteria, an accurate diagnosis currently depends on immunochemical confirmation, often complemented with ultrastructural and molecular genetic studies.9,10 Primary tumors of the CNS or PNS are distinguished from those secondary (metastatic) tumors that originate from extraneural organs and access the nervous system either hematogenously or by local extension. Approximately equal numbers of canine primary and secondary brain tumors occur in most large institutional series. However, in contrast in the cat, primary CNS tumors represent over 70% of the intracranial tumors and in general these tumors share fewer biologic similarities with their human counterparts than those in the dog. CNS neoplasms result in space‐occupying mass lesions that affect the surrounding brain parenchyma by their expansion. The most common clinical signs in dogs include changes in mentation, unilateral localization, and seizures. More aggressive neoplasms lead to infiltration and parenchymal destruction, with resultant edema, hemorrhage, necrosis, midline shifts, and reactive inflammation that result in exacerbation of clinical signs. The clinical consequences of CNS tumors depend upon their location and size as well as peri‐ and intratumoral changes. These neurological signs worsen with tumor growth and may become more diverse as new neuroanatomic sites become involved. Moreover, secondary hydrocephalus can occur if the flow of cerebrospinal fluid (CSF) is partially or completely obstructed by the tumor. Therefore, the clinical neurological examination is inadequate to determine the precise location of confined mass lesions. Their location and size is best visualized by neuroimaging with computerized tomography (CT) or by magnetic resonance imaging (MRI), both of which have become accessible to larger private veterinary clinical centers and university‐based veterinary hospitals. Follow‐up CT‐ or MRI‐guided stereotactic biopsy techniques can lead to a precise and rapid neuropathological diagnosis of such visualized mass lesions. Primary tumors of the CNS tend to be solitary masses, which result in lateralizing and progressive neurological signs. Changes in mentation and epileptiform seizures are also commonly associated with neoplasms in dogs. These signs broadly parallel those in human patients, where almost 50% of people with brain tumors develop epileptic seizures. Currently there is minimal scientifically based information available for canine CNS tumors with regard to their biological behavior, incidence, therapeutic response, or clinical prognosis compared with the rigorous and dependable data for comparable human tumors. In the future, the application of a standardized classification system, utilizing reproducible histological and grading criteria, combined with better identification of genetic and molecular signaling pathways will lead to improved prognostic information for canine CNS tumors. Conventional analysis of the CSF, which includes total and differential nucleated cell counts, and total protein concentration, has not been demonstrated to be of reliable diagnostic specificity for most nervous system tumors. Recently retrospective studies have demonstrated that cytospin CSF cell preparations can be useful indicators of neoplastic lesions. For example, oligodendroglioma cells can be readily identified and also at least 50% of all choroid plexus carcinomas have cytological evidence of malignancy compared with none in papillomas. Another emerging use of cytospin preparations is in combination with appropriate cell‐specific antigenic markers in order to immunocytochemically confirm the neoplastic cell‐type populations such as lymphomas. Most primary CNS tumors in dogs exfoliate a few neoplastic cells into the CSF but nondegenerate neutrophils are the most common nucleated cell. Some exceptions are lymphomas, histiocytic sarcomas, and granular cell tumors, in which sometimes nearly all of the cells in the CSF are neoplastic. Most tumors rarely produce any abnormalities in a complete blood cell count or serum chemistry, although nanoparticle technology is becoming an important tool for early detection of some primary and secondary tumors. Since brain tumors form space‐occupying masses within the cranial cavity, where expansion is limited by the cranial vault, a major objective of therapy is the elimination of the tumor mass. If this can be only partially accomplished then just short‐term clinical improvement can be expected. Long‐term survival may depend on significant tumor reduction by surgical debulking, radiation, or chemotherapy or combinations of these. Glucocorticoids can temporarily diminish the mass effect by reducing the peritumoral vasogenic edema. Evidence‐based data relating to outcome and optimal radio‐ or chemotherapy is either very limited or still lacking for most tumors of the nervous system in animals. However, advances in human diagnostic imaging, and application of advanced neurosurgical techniques and equipment (e.g., neuroendoscopy, surgical microscopy, stereotactic neuro‐navigation, and ultrasonic aspiration of tumors) are beginning to enter the veterinary field. Standard therapy beyond palliative care for the majority of canine brain tumors includes combinations of surgical resection, radiation, and systemic chemotherapy, but these are not based on scientifically defined criteria. Systemic chemotherapy alone has limited efficacy in most primary brain tumors, but there is minimal supportive data available. For meningiomas, surgical resection is considered the standard of care in most cases, with survival times of several years. For incompletely resected high‐grade meningiomas, adjunctive radiation therapy is attempted. Multiple novel therapeutic approaches as part of translational studies have shown promise and cover a spectrum of therapies.7,10 These include intratumoral convection‐enhanced delivery of chemotherapeutics such as CPT‐11, or antibodies bound to toxins targeting tumor cell–specific markers such as the IL‐13R receptor14 α2 and EGFR, and suicide‐based gene therapies with different methods and types of viral delivery, targeting of defined aberrant signaling pathways blocking specific tyrosine kinase receptors, gene therapy delivery of immunostimulatory genes (IL‐2, IL‐4, IL‐12, TNF alpha, and dendritic cell growth factors, such as Flt3L), as well as vaccine‐based approaches with primed dendritic cells.7,10,14,15 Genetic engineering with tumor‐specific chimeric antigen receptors (CARs) directs autologous patient T cells to the tumor site. The efficacy of these various conceptual approaches is encouraging enough to allow for the feasibility of these new modalities of treatment. The recent application in dogs and cats of techniques used in human neurosurgery for MRI or CT‐guided stereobiopsy has enhanced the capability for rapid and accurate diagnosis of mass lesions detected by neuroimaging techniques.10,13 Small core tissue samples can be obtained with remarkable anatomical precision. Cytological evaluation of intraoperative smear preparations from part of the biopsy needle core, immediately fixed in 95% alcohol and rapidly stained with haematoxylin and eosin (H&E) can provide a provisional histological diagnosis within 10 minutes with a high degree of diagnostic accuracy. This provisional diagnosis can be subsequently confirmed with either frozen or formalin‐fixed, paraffin‐embedded (FFPE) sections originating from the same core. Degenerative or inflammatory lesions pose more of a challenge than tumors due to the heterogeneous nature of their histological changes and the limited sample size, which can under‐ or misrepresent the underlying process.16–18 Neuroimaging has become an indispensable part of diagnostic neuropathology and although limited in providing specific diagnoses in individual tumors, in many cases a high degree of probability can be reached when comparing groups of tumors.10,13 Canine tumors, such as oligodendrogliomas and astrocytomas, or meningiomas and histiocytic sarcomas, can have very similar and overlapping imaging characteristics.10,12,13 Advanced imaging techniques, such as MRI, diffusion‐weighted imaging, and NMR spectroscopy, can help to further define intracranial masses and distinguish neoplastic from non‐neoplastic lesions. Advances in vascular imaging to define both tumor vascular supply and perfusion characteristics of masses may aid in future therapeutic strategies, such as tumor vascular embolization, as well as monitoring of antiangiogenic therapies in the dog.7,10 There are recent excellent and readily available texts for becoming acquainted with the basic physics and interpretation of various sequences now available for conventional MRI.7,9–12 The correlation of imaging findings with gross pathology is found in the cited references. IHC using a wide range of CNS/PNS cell‐specific antibody markers effective on FFPE tumors has become another indispensable technique for the confirmation of the diagnosis of nervous system tumors (Table 19.1). The prognostic utility of proliferation indices (e.g., Ki67, clone MIB‐1) and accurate mitotic indices as determined immunocytochemically (e.g., phosphohistones (PPH3)) for accurate counts is being prospectively evaluated in animal and human tumors. Similar to human tumors, analysis of canine tumors for genomic, epigenomic, transcriptome, and proteomic changes is likely to provide both insight into key tumor signaling pathways as well as a more prognostically and biologically relevant subclassification of tumors than is currently possible based on current histological criteria.9,10,12,15 Table 19.1 Immunophenotyping findings in primary and metastatic tumors in the CNS in FFPE tissues using routine diagnostic antibodies to cell‐specific markers FFPE, Formalin‐fixed, paraffin‐embedded; AFP, alpha fetoprotein; CK, cytokeratin; CRB, chromogranin B; DCX, double corticotrophin; PR, progesterone receptor; EGFR, epidermal growth factor receptor; GFAP, glial fibrillary acidic protein; MAP‐2, microtubule‐associated protein; PDGFRA, platelet‐derived growth factor receptor alpha; PLAP, placental alkaline phosphatase; SMA, smooth muscle actin; SMI 31, phosphorylated neurofilaments; SYN, synaptophysin; TNF, triple neurofilament; VIM, vimentin. Recent studies on the common signaling pathways in gliomas has dramatically altered the classification of some human tumors with respect to therapeutic outcome as well as providing a more focused assessment of newer therapeutic strategies. Imteractions and expression of the RAS/MAPK, P13/AKT, TP53, and RB1 pathways in canine gliomas suggest that there are specific pathway abnormalities in glioma subtypes that are shared with some human tumors although, for example, the higher expression of hyperphosphorylation of RB1, and the much lower incidence of mutant TP53 in canine gliomas indicates that caution should be exercised in direct extrapolation to the driver mutations in critical pathways of human tumorigenesis.7,8 We have listed a number of general references below which we hope will be useful for more detailed information and application beyond the necessarily limited data of this chapter (e.g., MRI interpretation, algorithms for differential diagnosis of gross, microscopic and immunocytochemical features of tumors, molecular genetics, key signaling pathways, etc.) and for more in‐depth information on specific tumors.9–12,15 Astrocytomas form a diverse subgroup of gliomas which, in the dog, are further subdivided into two distinct morphological subgroups based on their typical infiltrative versus well‐circumscribed growth patterns. The diffusely infiltrating tumors are classified as: (1) diffuse astrocytoma, (2) anaplastic astrocytoma, (3) glioblastoma, (4) gliomatosis cerebri, whereas the well‐circumscribed tumors are: (1) subependymal giant cell astrocytoma (SEGA), (2) pilocytic astrocytoma, (3) gemistocytic astrocytoma, (4) pleomorphic xanthoastrocytoma. Nevertheless these two subgroups of canine astrocytomas share many common features, for example MRI findings are not exclusively diagnostic, their incidence increases with age, they arise mainly within white matter of the cerebral hemispheres, and they can invade the cortex but generally not into the ventricular system.1–4 Their overall incidence is about 15% of all primary nervous system tumors, which is only exceeded by meningiomas, the most common tumor in both dogs and cats. Certain brachycephalic breeds (e.g., boxer, bulldog, and Boston terrier) have a relatively much higher incidence of astrocytomas (about 23 times) than in any other breeds.4 Astrocytomas have been sporadically reported in cats, horses, cattle, and pigs, but most of the following information is based on the canine tumors.4,5 The main criteria used for histological classification and grading (grades II–IV) of astrocytomas are increasing cell density, nuclear atypia, and mitotic count.3,4 The most malignant astrocytoma, grade IV, commonly called a glioblastoma, additionally must exhibit at least one of two other essential diagnostic features: (a) necrosis with marked glial pseudo‐palisading and/or (b) microvascular proliferation.2–4,6,7 Grade I tumors have a very low cell density and have not yet been recognized in animals, presumably due to their minimal clinical effect. The secondary structures of Scherer, consisting of three main morphological features of perineuronal and perivascular satellitosis, subpial spread, and invasion along the white matter tracts by neoplastic cells, are considered to reflect the upgraded migratory and invasive capability of a highly malignant clonal population of a glial tumor, of either astroglial or oligodendroglial origin (see Figure 19.10C).6 Astrocytomas will always express glial fibrillary acidic protein (GFAP) immunoreactivity to some degree, which decreases with increasing grades (see Figure 19.3B) and have a proliferation index (PI) up to 5, 10, and 35% in grades II, III, and IV, respectively (see Figure 19.4B).1,7 The diffuse astrocytoma has an infiltrating pattern of growth. Fibrillary astrocytoma is the descriptive classification used for this subtype of diffuse astrocytoma grade II. These tumors represent about 30% of all astrocytomas and occur with increasing frequency with advancing age. There is no gender predilection and they occur much more frequently in some brachycephalic breeds. Although they can be located anywhere in the brain or spinal cord, their preferential site is supratentorial, in the temporal and piriform lobes and less often in the frontal lobes (Figure 19.1A–C). The anaplastic astrocytoma grade III also has about a 30% incidence within the glioma group and can only be confidently distinguished from a grade II diffuse astrocytoma by histological criteria (Figure 19.1E,F).4,7 Figure 19.1 Astrocytoma diffuse, grade II and anaplastic grade III, canine. (A) T1‐weighted post‐contrast transverse MRI illustrating the iso‐ to hypointense noncontrast enhancing mass lesion of a grade II astrocytoma in the right piriform lobe. (B) A FLAIR MRI illustrating the typical diffuse hyperintensity with minimal apparent edema beyond the tumor margin. (C) Transverse section corresponding to the same slice level as in (B), illustrating the poorly defined whitish mass of the grade II astrocytoma obliterating normal gray and white matter structures in the right piriform lobe. (D) Smear preparation from a CT‐guided stereotactic biopsy of a grade II astrocytoma with intact capillaries (arrows), increased cell density due to elongate nuclei of neoplastic astrocytes with their prominent refractile processes. H&E stain. (E) Grade II astrocytoma with diffuse infiltration of parenchyma with a low density of neoplastic cells with elongate nuclei. (F) Anaplastic grade III astrocytoma with high cell density, some nuclear atypia and mitotic figures. Glioblastomas (astrocytoma grade IV) (Figures 19.2–19.4) are the most malignant primary CNS tumor of astrocytic derivation, most likely arising from a stem cell population in a subependymal niche around the lateral ventricles. Their incidence in the dog increases with age, tumors arise mainly supratentorially in subcortical white matter in parietal and temporal lobes, and glioblastomas represent about 30% of all canine astrocytomas (Figure 19.2B).4,7,8 It is an extremely rare tumor outside of these sites but is found in the spinal cord. They have been reported rarely in cats, horses, and cattle. No molecular genetic studies have yet been made in canine glioblastomas to distinguish, as in people, between the rapid onset of primary de novo and the much slower progress towards the secondary glioblastomas. The incidence of the latter is much lower (up to 10%) and they have a slower clinical evolution driven by progressively consistent genetic mutations from a diffuse grade II glioma. The primary and secondary glioblastomas have only been recognized to date in people: they are considered to be distinct entities that evolve through different genetic pathways, with characteristic genetic profiles, but they are similar histologically.7 Figure 19.2 Glioblastoma (astrocytoma grade IV), canine. (A) T1‐weighted post‐contrast transverse MRI illustrating the strong peripheral ring‐enhancement and heterogeneous enhancement within the center of the tumor. Marked mass effect is demonstrated by effacement of the lateral ventricle and deviation of the falx cerebri. (B) Transverse sections illustrating the variegated, well‐circumscribed glioblastoma with a marked right‐sided shift and left lateral ventricular compression. (C) Transverse section through a glioblastoma with the exclusive diagnostic hallmark of intratumoral serpentine necrosis and glial cell pseudo‐palisading. H&E stain. Figure 19.3 Glioblastoma (astrocytoma grade IV), canine. (A) Microscopically there is dense cellularity, prominent nuclear atypia, and numerous mitotic figures. H&E stain. (B) Strongly immunoreactive fibrillary processes of the astrocytoma with GFAP immunostaining. Figure 19.4 Glioblastoma (astrocytoma, grade IV), canine. (A) Microscopically there is prominent microvascular proliferation, and also necrosis with peripheral glial cell pseudo‐palisading, each being a characteristic hallmark of a glioblastoma. Note the marked nuclear atypia, increased cell density and high mitotic count compared with lower grade astrocytomas. (B) The PI is up to 35%. MIB‐1 immunostaining. (C) Many (up to 70%) of the cells are immunoreactive to Olig2. (D) Robust overexpression of EGFR as detected immunohistochemically is a common feature of glioblastomas. Gliomatosis cerebri is a diffusely infiltrating glioma in at least three separate brain lobes or in the spinal cord (Figure 19.6A,B). In dogs, the original diagnosis was termed microgliosis but the human tumor is of a morphologic and molecularly and genetically defined astrocytic phenotype.7 In addition, human oligodendrogliomas and mixed astro‐oligodendrogliomas may have a similar morphological pattern. Gliomatosis cerebri has characteristic long, thin, slender, almost helical hyperchromatic nuclei with minimal bipolar cytoplasm. Although very rare, it occurs in middle‐aged dogs.9 Recently a cluster suggestive of a familial inheritance pattern has been found in young siblings of several families of bearded collie dogs (A. Oevermann, personal communication, 2013). Infiltrative astrocytomas account for approximately 90% of all canine astrocytomas while the circumscribed astrocytic tumors form a much smaller subgroup. A subependymal giant cell astrocytoma (SEGA) has been reported once in a cat in a subependymal site of the lateral ventricle. Two pilocytic astrocytomas have been described in a larger case series of canine astroglial tumors as supratentorial tumors affecting the temporal lobe and thalamus.4 A third possible canine pilocytic astrocytoma has been described in the optic nerve, resembling the adult type of human pilocytic astrocytoma, termed optic chiasmatic‐hypothalamic glioma.10 Gemistocytic astrocytomas are a rare variant of well‐circumscribed astrocytomas, which in the canine are found mainly in the cerebellum and less often in the spinal cord (Figure 19.5A). They are characterized by a dominant fraction (>70%) of neoplastic cells resembling reactive gemistocytes.4,7 A pleomorphic xanthoastrocytoma has been described as an intra‐axial well‐demarcated mass in the medulla oblongata of a 13‐week‐old mixed‐breed poodle.11 Figure 19.5 Gemistocytic astrocytoma, canine. (A) Well‐circumscribed tumor within the cerebellum with intratumoral necrosis and hemorrhage. (Image courtesy of A. Oevermann, Vetsuisse Faculty, University of Berne.) (B) Smear from a CT‐guided stereobiopsy with marked nuclear atypia of gemistocytic‐like cells with abundant refractile astrocytic processes. H&E stain. (C) Most cells have abundant homogeneous eosinophilic cytoplasm with an eccentric nucleus and processes resembling reactive gemistocytes. Mitotic figures are rare. H&E stain. (D) Tumor cells are strongly immunoreactive to GFAP. Figure 19.6 Gliomatosis cerebri, canine. (A) FLAIR transverse MRI with diffuse, asymmetrical hyperintensity predominantly restricted to the corona radiata bilaterally. (B) Transverse section of brain corresponding to the same slice level as the MRI with focal areas of whitish edema most obvious on the right side of the white matter and which correspond to the areas defined in the FLAIR image. (C) There is a diffuse infiltration of neoplastic astrocytes with slender elongate nuclei longitudinally aligned within white matter tracts. H&E stain, (D) Tumor cell processes are strongly immunoreactive to GFAP, indicating their astrocytic derivation. (Images B–D courtesy of A. Oevermann, Vetsuisse Faculty, University of Berne.) (Image courtesy of P. Kircher, Vetsuisse Faculty, University of Zurich.) With MRI, most diffuse astrocytomas are hypointense on both T1‐ and T2‐weighted images (Figure 19.1A), and tend to have minimal post‐contrast enhancement.1,2 Anaplastic astrocytomas tend to have some T2‐weighted hyperintensity and also more pronounced post‐contrast enhancement (Figure 19.1B). Glioblastomas commonly have a post‐contrast peripheral ring or ring‐enhancing pattern with central T1‐weighted hypointense regions of necrosis on post‐contrast images (Figure 19.2A). FLAIR sequences illustrate moderate peritumoral edema, while T2*‐GRE images can detect the frequent intratumoral multifocal hemorrhages. However these findings only provide a provisional diagnosis with the histological confirmation of a specific subtype obtained from surgical or stereotactic biopsy samples.1,3,7,11 In gliomatosis cerebri, MRI findings mimic those of low‐grade diffuse astrocytomas in the brain and spinal cord, but lesions on T1‐ and T2‐weighted images are restricted to white matter, and are widespread, uniform, and distinctly multifocal (Figure 19.6A).7,8 Contrast enhancement is minimal to absent. The pleomorphic xanthoastrocytoma was variably hyperintense on T2‐weighted images,with some contrast enhancement on T1‐weighted, while the FLAIR sequences illustrated central hypointensity but was hyperintense peripherally. Diffuse astrocytomas on transverse sections of the CNS tend to have marked effacement of gray and white matter boundaries with solid, homogeneously white tissue with blurred margins (Figure 19.1C). Anaplastic astrocytomas can have a similar appearance. Glioblastomas tend to be relatively large, poorly demarcated, are variegated with areas of yellowish to white necrosis, acute and chronic hemorrhage and sometimes multiple cysts with a turbid content (Figure 19.2B). Spread by local extension across the corpus callosum into the contralateral hemisphere, a characteristic feature of human glioblastomas, is rare in canine glioblastomas.3 In gliomatosis cerebri there are areas of whitish uniform enlargement of the infiltrated cerebral hemispheric white matter, in the brainstem, or in the spinal cord (Figure 19.6B).9 SEGA in the cat is a well‐circumscribed mass that protrudes into the lateral ventricle from a primary subependymal site as a firm white mass. Pilocytic astrocytoma occurs as a well‐circumscribed mass in the thalamus, temporal lobe, and optic nerve.4 Gemistocytic astrocytomas are most common in the cerebellum and form well‐demarcated discrete, rather large, tan‐colored tumors with areas of yellowish necrosis and small clear cysts (Figure 19.5A). Grossly, the xanthoastrocytoma was a well‐demarcated central tan mass with an outer hemorrhagic border.11 The overall characteristic histologic features for the diagnosis of astrocytoma are of neoplastic cells resembling astrocytes, most of which have elongate plump spindloid nuclei, often with distinct bipolar processes but no distinct borders. The various subtypes of diffuse or well‐circumscribed astrocytomas are then individually graded based on their cell density, mitotic count, severity of nuclear atypism, and presence of necrosis with pseudo‐palisading and/or microvascular proliferation.1,2,4,7,8 The gemistocyic astrocytoma is an exception, with the distinctive appearance of sheets of plump cells with eosinophilic cytoplasm closely resembling reactive gemistocytic astrocytes.4,7 Diffuse astrocytoma grade II is usually composed of a fairly uniform population of loosely arranged neoplastic cells, which infiltrate imperceptibly within normal neuropil (Figure 19.1E). The most common cell type is the fibrillary astrocytoma composed of diffusely infiltrating elongate, spindloid, and occasionally polygonal cells that lead to a moderate increase in overall cellularity. A reliable hallmark of neoplastic astrocytes is their nuclear atypia, with enlarged or irregular hyperchromatic nuclei with scant cytoplasm and their fibrillary processes (Figure 19.1E). There may be cytological differences within the tumor, depending on regional anatomical involvement. Importantly, mitotic figures are not found.7 Entrapped, often vacuolated neurons are admixed with tumor cells infiltrating gray matter. Anaplastic astrocytoma grade III contains a pleomorphic, more dense cell population consisting of fusiform, polygonal, or round cells of variable sizes, often with distinct nuclear atypia; single and multinucleate giant cells may also be present (Figure 19.1F). Nuclei are larger and more hyperchromatic than in diffuse astrocytomas. There are also moderate mitoses. Anaplastic astrocytomas are highly cellular, have a high PI, and are infiltrative; their nuclei tend to align longitudinally along white matter tracts.1,2,4,5,7 Glioblastoma is characterized by marked cellular pleomorphism which may include a range from small undifferentiated cells to rare giant cells, high cell density, marked nuclear atypia, a high mitotic count, and an infiltrative growth pattern (Figure 19.3A). The two hallmarks essential for this classification, however, are areas of serpentine necrosis (Figure 19.2C) and/or microvascular proliferation (Figure 19.4A).1,2,4,7,8 Within areas of necrosis, intraluminal fibrin thrombi are found in areas of acute coagulation necrosis and areas of acute hemorrhage, presumably associated with rupture of large, thin‐walled ectatic blood vessels. As in all highly infiltrating gliomas, secondary structures of Scherer are common at the periphery of the tumor.6 Regional cellular heterogeneity of the astrocytic population, including gemistocytic populations and small round glioblastoma cells, or areas of oligodendroglial or neuronal differentiation, can complicate a diagnosis dependent on small tissue samples from stereotactically derived biopsy tissue. A canine giant cell glioblastoma subtype has been seen rarely. In gliomatosis cerebri there are diffusely infiltrating glioma cells with elongated, enlarged, uniformly hyperchromatic twisted nuclei and minimal detectable cytoplasm and typically with an increased cell density (Figure 19.6C). The mitotic count is low. Like their astrocytoma counterparts, the cells tend to align longitudinally within white matter tracts (Figure 19.6C).7,8 Secondary structures of Scherer are common.6 A single case of gliomatosis cerebri has been reported in the spinal cord of a cat.12 Feline SEGA is composed of a biphasic pattern of several pleomorphic cell types within a fibrillar matrix arranged in interlacing streams and perivascular rosette patterns. The cells vary from spindloid, arranged in sheets, to large and polygonal gemistocytic‐like cells (with abundant eosinophilic cytoplasm and eccentric nucleus) and/or ganglion‐like with eccentrically placed nuclei, abundant cytoplasm, large vesicular nuclei with prominent nucleoli (Figure 19.7A). Figure 19.7 (A) Subependymal giant cell astrocytoma (SEGA). A discrete mass composed of spindle, epithelioid and gemistocytic‐like cells arranged in nests or fascicles separated by fibrovascular stroma. There is a compact arrangement of large astrocyte‐like cells with abundant cytoplasm and large vesicular nuclei. Mitotic figures are rare. H&E stain. (B) Pilocytic astrocytoma, grade I. Biphasic appearance of alternating dense fibrillary and loose microcystic areas. The thin, elongate pilocytic‐like cells in the dense areas have occasional Rosenthal bodies but rare mitotic figures. H&E stain. (C) Astroblastoma. There is a dominant astroblastic pseudovascular rosette pattern of thick nonfibrillary processes radiating from a central blood vessel with outer placement of the nucleus. H&E stain. (Images courtesy of G. Stoica, Texas A&M University, College Station.) (Image courtesy of A. Oevermann, Vetsuisse Faculty, University of Berne.) Canine pilocytic astrocytomas have a histological biphasic pattern of varying proportions of compacted bipolar cells with a predominant hair‐like (piloid) pattern forming loose cellular bundles or areas of microcyst formation (Figure 19.7B). Rosenthal fibers, granular hyaline droplets, eosinophilic intracytoplasmic bodies, microcysts, and glomeruloid capillaries are common.4,7 The optic chiasmatic‐hypothalamic glioma type is further classified as an anaplastic astrocytoma as it has malignant cytological features such as necrosis and mitotic figures.10 In gemistocytic astrocytomas the predominant gemistocytic cell population has plump, glassy, eosinophilic cytoplasm with randomly oriented processes forming a dense fibrillary network. Their nuclei are eccentrically positioned with distinct nucleoli. Mitotic figures are extremely rare and the cells are overall cytologically bland (Figure 19.5C,D). Due to their characteristically abundant eosinophilic cytoplasm and short processes this may be the easiest astrocytoma subtype to diagnose on H&E‐stained sections.4,7 Astroblastomas are dominated by the astroblastic rosette comprising elongated tumor cells with eosinophilic cytoplasmic processes radiating around a central blood vessel (Figure 19.7C). In the pleomorphic xanthoastrocytoma, histologically, there were characteristic giant lipid‐bearing cells which were often GFAP immunoreactive, as were the predominant small elongate cells and their processes. Granular eosinophilic bodies were seen with reticulin fibers surrounding individual or clusters of tumor cells. Mitotic figures were rare.12 In both diffuse and circumscribed astrocytomas the cytology of intraoperative smear preparations varies with the histological grade. Distinguishing criteria between these grades are the hypercellularity, nuclear atypia, and cytoplasmic processes with a prominent fibrillarity. There is often a dense intertwining network of these processes. The nuclei tend to be larger, more irregular, more basophilic, and more pleomorphic than those of normal or reactive astrocytes (Figure 19.1D).7 These cells must be distinguished from reactive gemistocytic astrocytes, which are common in degenerative or inflammatory disease, and which have much more dense but focally restricted fibrillary processes. Anaplastic astrocytomas cannot always be distinguished from grade II diffuse astrocytomas although increased mitotic figures, increased cell density and pleomorphism are their cytological features. However, the histologic criteria for grading and classification schemes used on tissue sections cannot be applied from smears with as much accuracy without much experience. Glioblastomas have a more pleomorphic and denser cell population, many fibrillary processes, many normal and atypical mitotic figures, microvascular proliferation and sometimes giant cells. Gemistocytic astrocytomas have a distinctive profile with large, eosinophilic, neoplastic gemistocytic‐like cells admixed with a more conventional astrocytoma population and, together with their dense network of fibrillary processes, can be confidently identified (Figure 19.5B). A reliable marker for the identification of normal, reactive, or neoplastic astrocytes is GFAP, the main component of astrocytic intermediate filaments. In astrocytomas, vimentin immunoreactivity parallels that of GFAP while expression of both is less intense with increasing tumor grades (Figure 19.3B).7 Olig2 is immunoreactive in up to 70% of tumor cells in most grade II and III astrocytomas, while their respective PIs are up to 5% and 15%, respectively.1,2,4,7 In glioblastomas, immunoreactivity to GFAP is most reliable for confirmation of this diagnosis (Figure 19.3B). However, overexpression of epidermal growth factor reeptor (EGFR), platelet‐derived growth factor receptor alpha (PDGFRα) and insulin growth factor binding protein (IGFBP2) can also be detected immunocytochemically in a majority of these canine tumors.13 This closely parallels that expression in human glioblastomas (Figure 19.4D).3,4,7,11,14 The PI can range up to 35% (Figure 19.4B), while the Olig2 immunostaining can range up to 70% of all cells (Figure 19.4C).3,7,11 In canine gliomatosis cerebri in our experience, many neoplastic cells are immunoreactive for GFAP and vimentin despite being reported as nonreactive by some observers (Figure 19.6D). This difference in GFAP immunoreactivity has led to some confusion as to the histogenesis of this tumor cell type. The first description of this tumor as canine microgliosis was based on the assumption that morphologically these were microglial cells. However, consistent immunonegativity to both CD18 and Iba1, the most current specific markers for canine microglial cells, confidently excludes this diagnosis. In two recent canine cases of gliomatosis cerebri, vimentin and nestin immunoreactivity was also demonstrated, although GFAP was reported as immunonegative.8 In gliomatosis cerebri in a cat, GFAP reactivity was immunonegative.12 The feline SEGA had uniform immunoreactivity to S100 and to a lesser degree immunoreactivity with GFAP, neuron‐specific enolase (NSE), and neurofilament protein (NFP). Pilocytic astrocytoma has strong immunoreactivity to GFAP in the pilocytic cells and very weakly in the cells forming the microcystic foci. Gemistocytic astrocytomas can be easily confirmed by their variable to robust GFAP immunoreactivity combined with a low PI (<2%), the latter corresponding with their grade II status (Figure 19.5D). Astroblastoma is an extremely rare tumor with only one reported description in a 12‐year‐old, neutered, male, Belgian malinois/great Dane, while another astroblastoma is cited in a 7‐year‐old boxer dog in a case series of canine gliomas.4,14 However, this diagnosis does not yet fit into any defined categories within any current human classification systems. The first tumor was a multilobulated, firm, white to tan expansile mass in the cerebellum. Histologically, plump astrocytoma‐like cells have homogeneous eosinophilic cytoplasm and peripherally located nuclei. Their broad, coarse, cytoplasmic nonfibrillary processes were radially arranged as rosettes around well‐hyalinized blood vessel walls in a papillary form (Figure 19.7C). Cells were immunoreactive for GFAP and vimentin.14 The differential diagnoses are an ependymoma and a papillary meningioma. The criteria for exclusion of this former diagnosis depends, rather unconvincingly, on the absence of fibrillarity in the perivascular processes and prominent vascular hyalinization.10 Papillary meningiomas will be immunonegative for GFAP. Differences in the molecular genetic characterization between these tumors in people are more definitive.7 Oligodendrogliomas are diffusely infiltrating, well‐demarcated tumors derived from glial cells histologically resembling oligodendrocytes (Figures 19.9–19.11). Their overall incidence in dogs ranges up to 15% of all primary nervous system tumors with the well‐known higher incidence that oligodendrogliomas and other glial tumors have (at least 23 times greater) in certain brachycephalic breeds (e.g., boxers, Boston terriers, bulldogs, etc.) than in all other breeds.1 In cats, horses, sheep, and cattle they are very rare with only sporadic reports.2–4 The onset of neurological signs in canine oligodendrogliomas usually occurs between 5 and 11 years, without any gender bias, but their natural history is not well defined. Oligodendrogliomas arise in the white or gray matter of the cerebral hemispheres, with decreasing frequency caudally from the olfactory bulbs and frontal, temporal, and piriform lobes to the parietal and occipital areas with at least half exhibiting secondary intraventricular growth and invasion into the cortex (Figure 19.8B,C).1,5,6 They may extensively infiltrate gray matter with effacement of the normally well‐defined gray–white junctions, both on MRI and grossly.1,5,6 Oligodendrogliomas with predominant intraventricular growth have been described with focal neurocytic differentation and which therefore need to be excluded by their morphology and broadly immunonegative IHC (synaptophysin) from a differential diagnosis of a neurocytoma.7 Oligodendrogliomas rarely are found in the brainstem or spinal cord as primary sites, sometimes with metastases diffusely into the meninges and focally within the spinal canal.8 Diffuse canine spinal meningeal oligodendrogliomatosis without identification of a primary site has been rarely seen.4 Rarely oligodendrogliomas have been described as multifocal tumors,and can occur in conjunction with meningiomas and choroid plexus tumors.1,5,8 Figure 19.8 Oligodendroglioma, grade III, canine. (A) T1‐weighted post‐contrast transverse MRI with strong, but heterogeneous contrast enhancement. (B) Lateral view of the brain with swollen discolored gyri due to the oligodendroglioma infiltration. (C) Section of the same tumor with a sharply demarcated border, areas of hemorrhage and necrosis, cortical infiltration and secondary spread within the right lateral ventricle and a left midline shift. Figure 19.9 Oligodendroglioma, grade III, canine. (A) T2‐weighted transverse MRI. The tumor has a well‐defined border with heterogeneous hyperintensity, minimal hyperintensity beyond the tumor margins, and moderate mass effect. (B) Smear from a CT‐guided biopsy of this tumor. Most tumor cells are dispersed from capillaries, with round nuclei with some atypia and eosinophilic cytoplasm. H&E stain. (C) Biopsy core sample with oligodendroglioma cells and a prominent microvascular proliferation. H&E stain. (D) Nuclear immunoreactivity of almost all tumor cells with Olig2. (E) GFAP immunoreactivity is confined largely to astrocytes outlining the microvascular proliferation. (F) This high‐grade tumor had a surprisingly low PI of up to 3%. MIB‐1 immunostaining. Figure 19.10 Oligodendroglioma, grade III, canine. (A) There are sheets of cells whose nuclei are round with minimal eosinophilic or vacuolated cytoplasm, a sharp and well‐defined cytoplasmic border and an interstitial accumulation of a basophilic myxoid matrix, sometimes forming small cysts or lakes. H&E stain. (B) Areas of necrosis with glial cell pseudo‐palisading and/or microvascular proliferation are characteristic of grade III tumors. (C) Secondary structures of Scherer, indicative of a highly invasive tumor, include both perivascular infiltration (arrow) and neuronal satellitosis (arrowheads) by tumor cells. Figure 19.11 Oligodendroglioma, grade III, spinal cord, canine. There is overexpression of PDGFRα in most oligodendrogliomas (93%) as detected immunocytochemically. Unstained tissue at bottom left is a spinal peripheral nerve root. Punch biopsy (0.6 mm diameter) on a tissue microarray (TMA) section. Canine oligodendrogliomas are remarkably similar in their morphology and biological behavior to their human counterparts and thus, from a comparative neuro‐oncology viewpoint, are now recognized as the best spontaneous tumor of all species for translational molecular and innovative therapeutic studies, as are most other canine primary CNS tumors. Oligodendrogliomas are very rare in the cat in the spinal cord and brain.3,4 Oligodendrogliomas on MRI may be globular or irregularly shaped, mildly hypointense on unenhanced T1‐weighted images but can be heterogeneously hyperintense on T2‐weighted images (Figure 19.8A).5,6 Post‐contrast they can be variably enhancing, ranging from none to non‐uniform or with an intense peripheral ring‐enhancement (Figure 19.9A).5,6 Focal or regional enhancement is often distributed centrally or eccentrically and may have a serpentine outline. There is no correlation of any of their imaging features with tumor grade except that enhancement has been more common in grade III than grade II tumors.5,6 Intratumoral hemorrhages (detected with T2*‐GRE) and cyst formation may occur. Compared with almost identical MRI features of diffuse astrocytomas, infiltration of the cortex and growth into the adjacent ventricular system are much more likely with oligodendrogliomas (Figure 19.8). Oligodendrogliomas are often large, generally with a characteristic grayish‐blue, often gelatinous or mucoid, translucent matrix (Figure 19.8B). On section, they are usually discrete, well demarcated, soft, and gray to pink, may involve the cortex and expand to the gyral surface (Figure 19.8C). Intraventricular growth from local extension is common in the dog and cat. In the cat there also can be extensive local and metastatic invasion of the meninges.3 A primary canine leptomeningeal oligodendroglioma with spinal leptomeningeal oligodendrogliomatosis has been reported.4,8 Grade III oligodendrogliomas usually have multifocal variegated areas of hemorrhage, yellowish to white areas of necrosis, and gelatinous cystic foci (Figure 19.8C). A dog with both an oligodendroglioma and a separate meningioma has been reported (see Figure 19.12). Figure 19.12 Coexistence of an oligodendroglioma in the left caudate nucleus and septal area with intraventricular spread (arrows) and of a meningioma (arrowhead) in the cerebellum, canine. Oligodendrogliomas usually form dense, patternless sheets of monomorphic cells but can be arranged in long straight or curving cords or in cell clusters (Figures 19.9C and 19.10A). With a short interval (minutes) before fixation, the cells have an irregular hyperchromatic nuclei set in a lightly staining cytoplasm, each with a prominent cytoplasmic border. A perinuclear halo effect is common with delayed fixation and results in the essentially artifactual but classically described gourmet “honeycomb” cell pattern. There is a prominent distinctive capillary bed, often forming an almost lobular pattern. Marked nuclear atypia and occasional mitotic figures are compatible with a grade II oligodendroglioma. However in the human WHO grading system, foci of necrosis and/or microvascular proliferation or >6 mitotic figures/10 HPF are univariate markers necessary for a grade III (anaplastic) oligodendroglioma classification and which occurs in over 90% of all canine tumors (Figures 19.9C and 19.10B). Basophilic staining, mucinous‐like material can be commonly found interstitially and accumulating in large multifocal microcystic lakes (Figure 19.10A). Most grade III tumors have larger, more irregular, and less basophilic nuclei, a denser eosinophilic cytoplasm with scant vacuolization and prominent mitotic figures. The PI can be 2–5% and up to 20% in low‐ and high‐grade tumors, respectively (Figure 19.9F).1,5,6 The microvascular proliferations consisting of foci of tumor‐derived paracrine secretions of VEGF and PDGF, which induce proliferation of endothelial and smooth muscle cells, respectively, are arranged in long lines or clusters, at both brain–tumor margins and intratumorally (Figure 19.10B).10 In grade III tumors, there can be dilated thin‐walled vessels containing intraluminal fibrin thrombi associated with areas of necrosis and hemorrhage. The necrotic areas may have a mild peripheral glial cell pseudo‐palisading, but this never approaches the cell density seen with glioblastomas (Figure 19.10B). There is minimal parenchymal reaction, including edema at the tumor margins, but the secondary structures of Scherer (neuronal satellitosis, perivascular and subpial accumulation of individual migrating tumor cells) can be a feature of grade III tumors (Figure 19.10C). Histologically, the edges of the tumor are generally very sharp and discrete. Foci of calcification, which are so common in human tumors, tend to be rare in both grade II and III tumors. Oligodendrogliomas may also grow by extension along or under the leptomeninges and ependymal surfaces respectively. Associated with intraventricular growth there can be widespread intraventricular metastases, with tumor foci impinging upon the ependymal surface or invading the underlying neuropil away from the primary site. Two dogs have been described without a defined focal primary site, with a pattern of diffuse oligodendrogliomatosis in the spinal cord or in the brainstem meninges, and one with bilateral involvement of the piriform lobes.9 Multifocal oligodendrogliomas have been described.8 In the cat, there is extensive robust secondary spread within the ventricles and meninges.3 One has been described within the spinal cord.4 With suitable education and experience a provisional diagnosis of an oligodendroglioma or a mixed oligoastrocytoma can be made from intraoperative smears. Most characteristically the cells disperse readily and evenly, they are uniformly round with some minimal eccentrically located eosinophilic cytoplasm (Figure 19.9B). Compared with low‐grade tumors, smears from grade III tumors have more overt mitotic activity, marked nuclear atypia with very coarse chromatin and small multiple nucleoli and individual cell necrosis (Figure 19.9B). Foci of microvascular proliferation are classical determinants of a grade III tumor but are most reliably visualized in histologic sections. Careful analysis of cytospin preparations from CSF also has proved to be useful in clinical studies, with the recognition of exfoliated oligodendroglioma cells in both dog and cat tumors.3 There are still no reliable or available antibodies for cell‐specific markers for the routine confirmation of a diagnosis in FFPE oligodendrogliomas. The microvascular proliferations diagnostic of grade III tumors are composed of layers of human von Willebrand factor VIII–related antigen and CD31‐immunoreactive endothelial cells. Most of the remaining cells have cytoplasmic immunoreactivity for smooth muscle actin (SMA). Most oligodendrogliomas immunocytochemically overexpress PDGFRα (Figure 19.11).10 A transcription factor Olig2 can be immunoreactive in nuclei of most tumor cells (80–90%), but this factor is also a consistent marker for astrocytomas or mixed glial tumors (Figure 19.9D). In the spinal cord, confirmation of the diagnosis of oligodendrogliomatosis is largely based on immunoreactivity to Olig2. Canine oligodendrogliomas do not express myelin basic protein or myelin‐associated glycoprotein by immunostaining, although fresh‐frozen tumor tissue will be immunoreactive for galactocerebroside and sometimes for Sox‐10, another transcription factor. A huge number of monoclonal antibodies (mAbs) are available for labeling but most of these are unsuitable for routine diagnostic purposes as they are dependent on more sophisticated fixation methods. Intratumorally, reactive gemistocytic astrocytes, presumably present as a result of entrapment, can be demonstrated in small numbers immunocytochemically with GFAP. Mini‐gemistocytes which have an eccentric eosinophilic cytoplasm are GFAP‐immunoreactive round cells which have no cytoplasmic processes, and are interpreted as part of the tumor cell phenotypic profile, although they are not included in any grading criteria. GFAP immunoreactivity is common around perivascular areas within the tumor (Figure 19.9E). Occasional synaptophysin (SYN) immunoreactive clusters of cells identifying neuronal differentiation, but of uncertain significance, can be demonstrated. The PI is up to 5% and 20% in well‐differentiated (grade II) and anaplastic (grade III) subtypes, respectively (Figure 19.9F). The main differential diagnosis that needs to be considered, particularly of a tumor with intraventricular growth and especially within the lateral or third ventricles, is the central neurocytoma (see Figures 19.8C and 19.24A,B). Neurocytomas share surprising close phenotypic patterns and cytology with those of oligodendrogliomas (vide infra), with which it has been confused in people. However, neurocytomas are strongly immunoreactive for SYN, NeuN, NSE, and other neuronal markers, but imunonegative for Olig2, and thus can be reliably differentiated from oligodendrogliomas (see Figure 19.24C,D). In the dog, a clear cell ependymoma also needs to be considered with any provisional oligodendroglial diagnosis. Clear cell ependymomas can be best separated on the basis of their strong uniform cytoplasmic GFAP immunoreactivity and confirmed ultrastructurally by their desmosomal junctions and intermediate filament content (see Figure 19.16A–C). In the dog, oligoastrocytomas have prominent areas of oligodendroglial differentiation (see Figure 19.13C). Diffuse astrocytomas can be challenging, particularly from stereotactic‐derived biopsy tissue where there is minimal tissue. However, the nuclei of astrocytomas are more elongated and angular compared with those of oligodendroglioma (Figures 19.1F and 19.3A). Some glioblastomas may have small areas of oligodendroglioma‐like differentiation. Oligoastrocytomas are diffusely infiltrating gliomas composed of a pleomorphic mixture of tumor cells resembling both diffuse astrocytoma and oligodendroglioma phenotypes. These forms are classified as either intermingled (diffuse) or compact (collision) tumors (Figure 19.13B). In people and dogs, up to 90% present as the diffuse type. Their higher incidence in some brachycephalic breeds parallels that of pure gliomas, and mixed gliomas likewise tend to exhibit an increasing incidence with age. Their overall incidence in dogs is up to 5% of all primary nervous system tumors. These tumors arise in the same predilection sites in the cerebral hemispheres as their individual glial cell tumor components, most commonly in the temporal and piriform lobes (Figure 19.13B). One tumor in a young dog has been described with discrete, multifocal distribution involving both cerebral hemispheres. Figure 19.13 Mixed glioma, canine. (A) T1‐weighted post‐contrast MRI with a well‐defined, heterogeneously hypointense mass with minimal contrast enhancement and moderate mass effect with deviation of the falx cerebri. (B) Transverse brain section through the same slice with both a translucent (arrow) (oligodendroglioma) and more solid tissue (arrowhead) (astrocytoma) in a mixed collision type of an oligoastrocytoma with a slight left‐sided shift. (C) Microscopically at the collision boundary of the oligodendroglioma with vacuolated cells with round nuclei (bottom right) and a diffuse astrocytoma with elongate nuclei and fibrillary processes (top left). H&E stain. There is no specific grading scheme for mixed gliomas. The histological criteria used in the individual glioma components also applies (e.g., necrosis, cell density, nuclear atypia, mitotic activity, and microvascular proliferation). Transgenic mouse and stem cell studies confirm the current hypothesis that these tumors arise from one stem cell population able to differentiate into these biphasic cell lines. Very rarely in the dog we have found ependymoma‐like cells replacing the astrocytoma population; such tumors are then classified as oligo‐ependymomas. Two feline spinal cord oligoastrocytomas have been reported.1 Oligoastrocytomas have very similar MRI characteristics to those of grade II oligodendrogliomas and diffuse astrocyomas (Figure 19.13A). Cystic cavitations as interpreted on MRI have been shown to correlate histologically with areas of low cell density of the fibrillary astrocytoma component. Grossly, mixed gliomas may present as a mass demarcated regionally into the gross features of both an astrocytoma and an oligodendroglioma (Figure 19.13B). Microscopically, these two cell types may appear most commonly as either equally admixed and intermingled (diffuse) or rarely (5–10%), but more easily recognized, juxtaposed into two or more (compact), separate cell clusters of each component – the so‐called collision tumors (Figure 19.13C). The diffuse type is the most diagnostically challenging type because of the ambiguous morphology of both populations. In canine oligoastrocytomas, areas of oligodendroglioma dominate, with up to 30% composed of astrocytomas.2 The astrocytic component can be strongly GFAP immunoreactive. Neoplastic astrocytes also have a distinctive phenotype with a reduced amount of cytoplasm, very thin and wavy processes, and the cells tend to be more dense compared with entrapped but scattered gemistocytic astrocytes (Figure 19.13C). Recognition of GFAP‐immunoreactive mini‐gemistocytes will increase the level of suspicion of an oligodendroglioma. However, it can be very difficult to make this diagnosis or to confirm with immunocytochemistry when confronted with less well‐differentiated cells without typical neoplastic phenotypes. Most canine grade II oligoastrocytomas have a minimal to absent mitotic rate with the PI up to 3%, compared with up to 15% in the much less common grade III tumors. The astrocytoma population can be visualized with GFAP immunoreactivity, often displaying small wavy cell processes, while Olig2 expression can only confirm that both populations are of glial cell origin. Immunocytochemically there is strong expression of PDGFRα in the oligodendroglial cell population. The main challenge with this tumor is establishing it as a mixed tumor and not as a relatively pure population of either an oligodendroglioma or astrocytoma. Otherwise the same differential considerations apply for each of these tumors as considered previously. As with other gliomas, too few canine and feline cases have been followed to know how they behave clinically.1,2 Ependymomas are relatively rare tumors that originate from ependymal cells (possibly of radial glial stem cell derivation) which line the ventricular system of the brain and spinal cord (Figures 19.14–19.17).1 Ependymomas have been sporadically reported in dogs, cats, cattle, and the horse.2–6 Canine ependymomas occur as a slow‐growing, supra‐ or subtentorial expansile mass, mainly within either a lateral ventricle or less often in the third and fourth ventricles, and rarely within the central canal of the spinal cord.1,2 They constitute about 3% of all primary canine CNS tumors. Their incidence increases with age and without any gender bias. In cats, a recent series (n = 18) of ependymomas has been described with a mean age of 8.5 years.6 Fifteen of these feline ependymomas were located intraventricularly and classified as the same histological subvariants in the human WHO scheme, while the three extraventricular tumors were designated as subependymomas or primary ependymomas.1,6 Figure 19.14 Ependymoma, papillary subtype, canine. (A) T2‐weighted transverse MRI showing a well demarcated intraventricular mass. The mass is diffusely and moderately hyperintense with numerous punctate, more strongly hyperintense areas superimposed throughout the tumor. (B) Transverse section through the ependymoma that has a rough granular cut surface. (C) Microscopically the papillary subtype has a papilliform structure with centrally placed blood vessels from which fibrillary processes of cells radiate centripetally, while the nucleus is peripheral, creating a characteristic nuclei‐free zone around blood vessels. (D) The central processes, attached to the central vascular core, are strongly immunoreactive to GFAP, thus excluding a choroid plexus tumor. Figure 19.15 Clear cell ependymoma, canine. (A) T1‐weighted post‐contrast transverse MRI showing a homogeneously hypointense well‐demarcated intra‐axial frontal lobe mass. (B) Corresponding gross section of the frontal lobe displaying an elongate cystic mass within the ventricular system. Figure 19.16 Clear cell ependymoma, canine. (A) Microscopically the cells have round nuclei with a globular eosinophilic cytoplasmic mass, a sometimes vacuolated cytoplasm and a sharply defined cytoplasmic border. H&E stain. (B) The globular cytoplasmic inclusions are characteristic and strongly uniformly immunoreactive to GFAP. (C) TEM illustrating desmosomal junctions between cells (arrowheads) and intermediate filaments (arrow) forming the globular inclusions. (D) A photomicrograph of a section of examined with TEM of an ependymal cell rosette with intraluminal cilia (arrow) and their anchoring blepharoplasts (arrowheads). Ependymomas are generally large, intraventricular, well‐demarcated masses that with MRI are hypointense on T1‐weighted, hyperintense on T2‐weighted, are discreetly circumscribed and with varying degrees of post‐contrast enhancement display a granular heterogeneous profile (Figure 19.15A). Obstructive hydrocephalus may be demonstrated rostral to the tumor site. Peritumoral edema is minimal.5,7,8 Grossly, they are mostly well demarcated, soft, tan to red, with a smooth texture on cut surface in the dog, more granular in the cat and even more so in the papillary variant in all species (Figures 19.15B and 19.17A).2,3,5,7 Intratumorally there can be cystic areas, necrosis, and focal hemorrhage. Although growth is usually confined within the ventricular system, ependymomas may infiltrate the adjacent neuropil or meninges. A secondary obstructive hydrocephalus may develop, depending on their location.2 The dichotomous epithelial and glial features of ependymomas are characteristic (Figure 19.17C). Both cellular and papillary ependymomas have a distinctive appearance due to the formation of a highly branching vasculature with firmly attached palisading tumor cells. These cells have an outer polar location of the nucleus and elongated cytoplasmic attachments radially arranged around blood vessels (Figure 19.17C).1 Cellular variants display numerous cells packed closed around vessels (Figure 19.17C). Individual cells have long tapered to oblong unipolar cytoplasm with distinctive processes. In the dog, cellular and papillary ependymomas comprise the two major histological variants, while clear cell ependymomas occur rarely.2–5,7 Cellular ependymomas are moderately to densely cellular and well vascularized, with a characteristic key feature of nuclear‐free perivascular zones formed by tumor cell processes. These zones can appear fibrillated, are GFAP immunoreactive, and lead to the formation of characteristic perivascular pseudorosettes around a centrally placed blood vessel. These are to be distinguished from true ependymal rosettes that have an empty lumen (Figures 19.17D and 19.18C,D).8 Neuroblastomas can have Homer–Wright rosettes.8 The tumor cells are also clustered individually without any obvious orientation between these blood vessels. Cell nuclei are round to ovoid with poorly defined cytoplasmic borders between cells. Mitotic figures are rare. Figure 19.17 Ependymoma, feline. (A) Sequential sections illustrating the large, well‐demarcated, granular tumor mass with marked left shift. (B) There are many true ependymal rosettes (arrows) which have sharply defined lumens throughout the section as well as pseudorosette formation around a central blood vessel core. H&E stain. (C) Intraoperative smear with pseudovascular rosetting of neoplastic cells oriented around blood vessels and which are also disseminated individually. The dispersed neoplastic cells have sharp cell borders, abundant eosinophilic cytoplasm and an easily recognizable columnar shape. H&E stain. (D) An ependymal rosette with intraluminal cilia and anchoring blepharoplasts. Toluidine blue‐stained plastic‐embedded thick section. Figure 19.18 Diagrams of different rosette types. (A) A Homer–Wright rosette where a halo of neuroblastic cells surrounds a lumen filled with their processes. (B) A Flexner–Wintersteiner rosette, characteristic of a retinoblastoma or pinealoma, where the halo of neuroblastic cells form a largely empty lumen into which small cytoplasmic extensions protrude. (C) An ependymal rosette with the cell halo surrounding an empty lumen which may contain microvilli and cilia. (D) A perivascular rosette with a halo of cells surrounding a blood vessel core. (Originally published in Neuropathology: A Reference Text of CNS Pathology, 2nd edn. (eds. D. Ellison et al.). Elsevier Mosby, Philadelphia, PA, pp. 677–684, 2004; with copyright permission from Elsevier Ltd.) In the other common variant, the papillary ependymoma has papilliform‐like fronds covered on their luminal surface by neoplastic ependymal cells, whose long refractile GFAP‐immunoreactive processes terminate on the underlying vascular stroma.2 The third variant is a clear cell ependymoma which occurs mainly in the lateral ventricles of dogs. The tumor cells have round nuclei and empty perinuclear halos and well‐defined cytoplasmic borders resembling oligodendrocytes, although these cells have diagnostically characteristic globular eosinophilic intracytoplasmic inclusions which are strongly and uniformly immunoreactive for GFAP (Figure 19.16A,B).7 In contrast, in the cat other histological variants not yet reported in the dog are: subependymoma, tanycytic ependymoma, and primary extraventricular ependymoma.1,2,6 All these variants exhibit characteristic perivascular pseudorosettes, although true ependymal rosettes are not common (Figure 19.17B). Feline cellular ependymomas are densely cellular, the cells have minimal cytoplasm and darkly staining basophilic nuclei, and have higher numbers of both perivascular pseudorosettes and ependymal rosettes than the canine ependymoma (Figure 19.17B,D). The ependymal lumens sometimes contain cilia whose anchoring blepharoplasts stain as dark blue dots with the phosphotungstic acid hematoxylin (PTAH) stain (Figure 19.17D). Between the ependymal rosettes and the pseudorosette formations there are solid sheets or clusters of cells without any defined pattern. No grading system has been applied to these feline tumors.6 An equine myxopapillary ependymoma has been described in the caudal fossa, an unusual site compared with the most common location in the conus medullaris in people.1,3 In a calf, an ependymoblastoma was diagnosed on the basis of age, the small round cells, and numerous mitotic figures. However, this diagnosis is claimed to be neither precise nor specific and some authors want it abandoned.9 As a group, ependymomas are relatively benign cytologically and in dogs are generally classified as grade II. Tumors classified as anaplastic ependymoma grade III exhibit pleomorphism with nuclear atypia, frequent mitoses (>4/10 HPF at 400×), pseudo‐palisading necrosis, and microvascular proliferation. Well‐differentiated ependymomas have a uniform and consistent cytoplasmic GFAP immunoreactivity. Vimentin and S100 are also expressed but to a much lesser intensity and extent, while pancytokeratin expression (Lu5, AE1AE3)is variably immunonegative and almost always Olig2 immunonegative. The PI is very low at <1%, which is consistent with their low grade and well‐differentiated morphology. Some authors have claimed that canine ependymomas are mainly GFAP immunonegative, but this has not been our experience, and may just reflect differences in methodology or an incorrect diagnosis. Positive immunoreactivity with GFAP may reflect their radial glial stem cell derivation.1 Ependymomas of all variants contain large numbers of intermediate filaments, sometimes arranged in a whorling pattern. Adjacent cells are connected by prominent desmosomal junctional complexes of varying lengths and patterns (Figure 19.16C).1,7 These junctions are most dense near the lumens of true rosettes. Microvilli and normally configured cilia protrude into the lumen of ependymal rosettes, particularly in cats (Figure 19.18). Cilia extend from and are anchored by blepharoplasts. The basal poles of the cells rest on a basal lamina of vascular origin and are anchored there by hemidesmosomes.1 Differentiation between the papillary variant of ependymoma and an anaplastic choroid plexus tumor may be difficult on H&E sections. True ependymal rosettes with empty central lumens are present only in ependymomas (Figures 19.17B,D and 19.18C).8 Together, the positive GFAP and negative pancytokeratin immunostaining in ependymomas are the most reliable criteria for diagnosis.1,7 Choroid plexus epithelium consistently expresses cytokeratins. The choroid plexus tumor also has a GFAP‐immunonegative fibrovascular stroma, while GFAP‐immunoreactive glial processes abut the core vasculature in ependymomas. Clear cell ependymomas can be differentiated from oligodendrogliomas by their positive cytoplasmic GFAP immunoreactivity (Figure 19.16B), negative Olig2 immunoreactivity, and ultrastructurally by their desmosomal junctions and cytoplasmic intermediate filament content (Figure 19.16C).1,7 The spinal thoracolumbar tumor of young dogs, currently designated as an ectopic nephroblastoma, has been commonly misdiagnosed historically as an ependymoma or even as a medulloepithelioma.2,4 Increased diagnostic vigilance is suggested when evaluating spinal cord tumors in young dogs between T9 and L5 segments. WT‐1 immunoreactivity in spinal nephroblastomas is considered pathognomonic (see Figure 19.27D). Ependymomas can also be confused with neuroblastomas, but the latter have neuroblastic (Homer–Wright) rosettes not pseudorosettes, and neuroblastomas may also express neuronal‐specific cell markers (e.g., SYN, NeuN, and TNF).1,6
Tumors of the Nervous System
INTRODUCTION
General considerations
Classification and grading systems
Clinical characteristics
Clinical pathology
Current advances in brain tumor therapy
New emerging techniques in diagnostic neuro‐oncology
Tumor type
Immunoreactive
Immuno‐negative
Variable immunoreactivity
Astrocytoma
VIM, GFAP, Olig2, EGFR, nestin
CK
SYN, P53, SMA, factor VIII RAg, nestin
Oligodendroglioma
Olig2, GFAP, PDGFRA
CK
SYN, NeuN, SMA, factor VIII RAg, nestin
Mixed glioma (oligo/astrocytoma)
Olig2, GFAP
CK
CK (AE1,3)
Mixed glioma (oligo/ependymoma)
Olig2, GFAP
CK
Gliomatosis cerebri
GFAP, VIM
Iba1, CD18
SEGA
GFAP, VIM
CK
Choroid plexus tumors
VIM, GFAP, CK (AE1,3), laminin
E‐Cadherin, SYN, B‐catenin, transthyretin, GFAP
Ependymoma
CK (AE1,3), GFAP
SYN
Ganglioglioma
Gangliocytoma
SYN, GFAP, NeuN, TNF, SMI 31, CGB, MAP‐2
Central neurocytoma
SYN, NeuN
LCA, CK, OLIG2, TNF, CGB
GFAP
Paraganglioma
SYN, NeuN, CGB,
CK
GFAP, HMB‐45
PNET
Medulloblastoma
SYN, GFAP, NeuN, MAP‐2, SMI 31, TNF, SYN, TNF, SMA 31, NeuN
CK (AE1,3), CLA
PAX5, NESTIN, WT‐1
Neuroblastoma
Ectopic nephroblastoma
WT‐1, VIM, CK (Leu5, AE1,3)
GFAP, SYN, NeuN
Laminin
Meningioma
VIM, CK, E‐cadherin,
GFAP
Claudin‐1, PR, CD34, N‐cadherin, DCX
Meningioangiomatosis
VIM,
Laminin, CK
Granular cell tumor
Ubiquitin
CD18, Iba1, canine leukocyte markers
Lymphoma/neurolymphomatosis
CD18, Iba1, CD45R, CD3, CD20, CD79a, granzyme B, PAX5
CK, GFAP, SYN
Intravascular lymphoma
CD3, CD79a, PAX5, CD45R
CK, GFAP, SYN, HMB‐45
Histiocytic sarcoma
CD1a, CD11c, MHC class II, CD18, Iba1
CK
Germinoma
AFP, c‐KIT, OCT4
CK
Teratoma
VIM, CK, PLAP
Desmin, SMA
Cranial and spinal nerve tumors
See Table 19.3
Metastatic (secondary) tumors
See algorithms in literature
References
PRIMARY TUMORS OF THE CENTRAL NERVOUS SYSTEM
Astrocytoma
General features, classification, and subtypes
Definition, incidence, and sites
Neuroimaging
Gross morphology
Histological features
Intraoperative cytology
Immunohistochemistry
Other nonclassified glial neoplasms
References
Oligodendroglioma
Definition, incidence, and sites
Neuroimaging
Gross morphology
Histological features
Intraoperative cytology
Immunohistochemistry
Differential diagnosis
References
Oligoastrocytoma (mixed glioma)
Definition, incidence, and sites
Neuroimaging and gross pathology
Histopathology and immunocytochemistry
Differential diagnosis
References
Ependymal tumors
Definition, sites, and incidence
Neuroimaging and gross morphology
Intraoperative cytology
Histopathology
Immunohistochemistry
Electron microscopy
Differential diagnosis
References
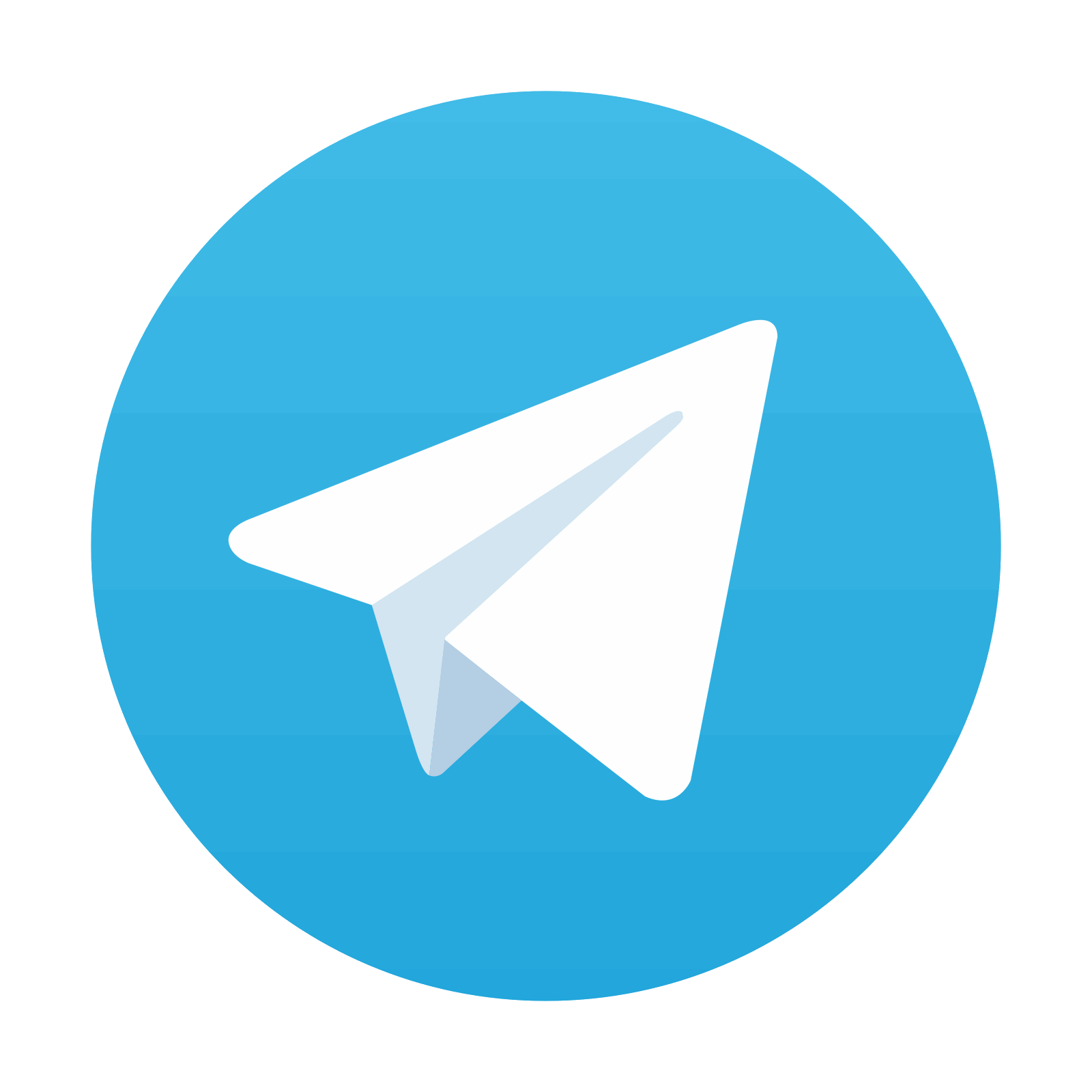
Stay updated, free articles. Join our Telegram channel

Full access? Get Clinical Tree
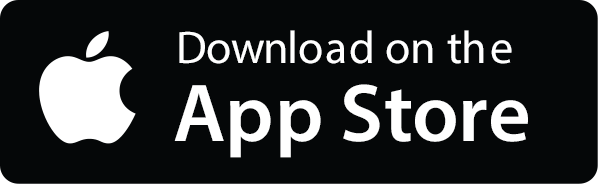
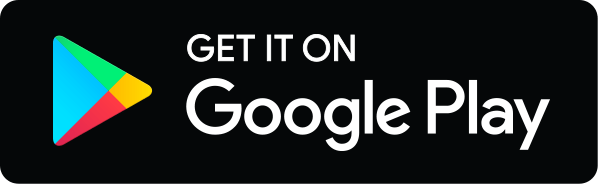