16 Sharon L. Crowell‐Davis University of Georgia, Athens, GA, USA The tricyclic antidepressants (TCAs) act as inhibitors of both serotonin and norepinephrine. They also have antihistaminic and anticholergic effects and are α‐1 adrenergic antagonists. The extent of these effects varies widely between the different TCAs (see Table 16.1). Some have strong serotonin reuptake inhibition and weak norepinephrine reuptake inhibition. Others have strong norepinephrine reuptake inhibition and weak serotonin reuptake inhibition. Other molecular activities vary widely as well. For example, amitriptyline has much stronger antihistaminic effects than clomipramine. Table 16.1 Acute in vitro biochemical activity of selected tricyclic antidepressants. Source: (Potter 1984; Potter et al. 1991; Richelson and Nelson 1984; Richelson and Pfenning 1984; Potter et al. 1995). Chronic administration of TCAs results in decreased numbers of β‐adrenoceptors and serotonin receptors and altered function of various serotonin receptors in the forebrain (Vetulani and Sulser 1975; Sulser et al. 1978; Heninger and Charney 1987; Potter et al. 1995). These long‐term changes in receptor number and function are believed to contribute to the significant changes in behavior that evolve over time when a pet is maintained on these medications. All of the TCAs are readily absorbed from the gastrointestinal tract. Peak plasma levels occur over a wide range of time, two to six hours being the mean peak time for various drugs. Half‐lives vary widely as well, but are long, somewhere in the range of 24 hours. In the liver, they undergo demethylation, aromatic hydroxylation, and glucuronide conjugation of the hydroxy metabolite (Potter et al. 1995). Tricyclic antidepressants are so named because of their central three‐ring structure. The tertiary amines have two methyl groups at the end of their side chain, while the secondary amines have only one. The type of side chain is substantially related to the molecular action. Tertiary amines have a proportionately greater effect on blocking serotonin transport, while the secondary amines have a proportionately greater effect on blocking norepinephrine transport (Bolden‐Watson and Richelson 1993; Tatsumi et al. 1997; Nelson 2004). Only the six TCAs that have been most commonly used in veterinary behavior will be discussed in this chapter: the tertiary amines amitriptyline, clomipramine, doxepin, and imipramine, and the secondary amines desipramine and nortriptyline. Like the selective serotonin reuptake inhibitors (SSRIs), the TCAs have anxiolytic, anticompulsive, and antiaggressive effects, in addition to antidepressant effects. In veterinary clinical behavioral medicine, they are used primarily for the first three effects. As with the SSRIs, the onset of effect takes several days to several weeks, and clients whose pets are placed on a TCA need to be cautioned of that so that they do not have unrealistic expectations. While occasional pets have a rapid response to a single dose, as a general rule, they should not be prescribed on an as‐needed basis. One of the TCAs, clomipramine, is the only psychoactive medication with anxiolytic properties to be FDA‐approved for an anxiety disorder. Specifically, Clomicalm is approved for the treatment of separation anxiety in dogs, when used in combination with behavior modification. Clomipramine is the most serotonin‐selective of the commercially available TCAs. Common uses in domestic animals include anxiety, affective aggression, compulsive disorder, and urine marking. Side effects vary widely, as there is a substantial range of effect on serotonin and norepinephrine between the various TCAs, as well as substantial variation between the TCAs in molecular effects other than those on serotonin and norepinephrine. In general, side effects may include sedation, constipation, diarrhea, urinary retention, appetite changes, ataxia, decreased tear production, mydriasis, cardiac arrhythmias, tachycardia, and changes in blood pressure. There is some evidence from the human literature that antidepressant drugs that have a sedative action and an antimuscarinic effect can interfere with memory. Amitriptyline is an example of one such medication that has both a high occurrence of antimuscarinic and sedative effects. Clomipramine, on the other hand, has some antimuscarinic and sedative effects, but not as much as amitriptyline, and has no measurable effect on learning and memory (Liljequist et al. 1974; Thompson 1991). However, mice injected with 10 mg kg−1 of either amitriptyline or clomipramine did not show any deficits in learning or memory when tested in a maze test (Nurten et al. 1996). TCAs should never be given in combination with MAOIs. This includes such various compounds as selegiline, which is used for the treatment of canine cognitive dysfunction, and amitraz, which is used for the treatment of demodicosis and is also a common compound in collars designed to prevent infestation with ticks. There is no antidote for overdose of any of the TCAs, although physostigmine given intravenously has been shown to be useful in alleviating cardiac and central nervous system (CNS) toxic effects in humans (Falletta et al. 1970; Slovis et al. 1971). Treatment must consist of decontamination and supportive therapy; however, emesis is contraindicated. While discontinuation of a TCA does not generally produce significant withdrawal symptoms per se, it is generally recommended that a patient that has been medicated with a TCA for several months be weaned off gradually. It may be that, while a behavior problem has resolved at a given dose, it recurs when the patient is off medication or even on a lower dose. Therefore, tapering of the dose while monitoring can allow for identification of dose levels at which the problem returns, and initiation of appropriate management and behavior modification protocols. As with the SSRIs, the TCAs should not be given on an as‐needed basis, because they act by producing a gradual shift in levels of serotonin and/or norepinephrine and by down‐regulation of the postsynaptic neurons. While some patients may begin to exhibit a response after just a few days of daily administration, others that ultimately have a good response may not respond for several weeks. Doses of selected TCAs in cats, dogs, horses, and parrots are given in Tables 16.2 and 16.3. Table 16.2 Doses of selected tricyclic antidepressants in dogs and cats. Note: Always start with a low dose and titrate up as necessary if the patient does not exhibit side effects. All doses are given orally. Table 16.3 Doses of selected tricyclic antidepressants in horses and parrots. Note: Always start with a low dose and titrate up as necessary if the patient does not exhibit side effects. All doses are given orally. Amitriptyline inhibits the reuptake of norepinephrine and serotonin. It is rapidly absorbed and metabolized. Plasma concentrations correlate with total intake of amitriptyline (Rudorfer and Robins 1982). In dogs, the primary metabolic pathways are hydroxylation, glucuronide hydroxylation, methyl hydroxylation and N‐demethylation, respectively (Lee et al. 2015), It is metabolized into nortriptyline and a variety of other metabolites (Diamond 1965). In dogs, the peak concentration occurs at two hours (Kukes et al. 2009). The half‐life in humans is 5–45 hours (Nelson 2004), and the half‐life in dogs given amitriptyline orally is 4.5–8 hours (Shanley and Overall 1992; Kukes et al. 2009; Norkus et al. 2015a). Being fed vs. fasted prior to administration of oral amitriptyline does not significantly affect the half‐life (Norkus et al. 2015a). However, giving amitriptyline IV extends the half‐life to 10–11 hours (Norkus et al. 2015b). Amitriptyline is excreted in the milk. In rabbits given carbon‐14‐labeled amitriptyline, the concentration of radioactivity in the milk is equivalent to concentrations in the serum. Concentrations of radioactivity in neonates consuming the milk are substantially lower than concentrations in the equivalent organs in the mother (Aaes‐Jørgensen and Jørgensen 1977). Amitriptyline is approved for the treatment of depression. Amitriptyline is contraindicated in patients with a history of sensitivity to this or other TCAs. It should not be given concurrently with MAOIs, because serious side effects, including convulsions and death, may result. If a patient is to be changed from an MAOI to amitriptyline, discontinue the MAOI for at least two weeks before beginning amitriptyline. Avoid or use it cautiously in patients with a history of seizures, urinary retention, or glaucoma. In humans, amitriptyline has been shown to cause cardiac arrhythmias, tachycardia, and prolonged conduction time. While dogs do not appear to be as susceptible to cardiotoxic side effects as humans (e.g. Reich et al. 2000), it should be avoided or used cautiously in veterinary patients with existing cardiac disease. Sufficiently large doses can induce cardiotoxicity. In a study in which anesthetized dogs were given a continuous intravenous infusion of amitriptyline until cardiotoxicity occurred, toxic effects were observed at an average of 25 mg kg−1 (range 15–80 mg kg−1) in one study and an average 36 mg kg−1 in another study (Lheureux et al. 1992a, 1992b). Rabbits given amitriptyline by intravenous injection exhibit decreased blood pressure and increased heart rate (Elonen et al. 1974). Amitriptyline may enhance the effects of barbiturates and other CNS depressants (Merck 1998). Amitriptyline is metabolized in the liver and excreted through the kidneys. It should therefore be used cautiously and at lowered doses in patients with mild to moderate liver disease and avoided entirely in patients with severe liver disease. Levels of amitriptyline may be elevated in patients concomitantly given drugs that are metabolized by cytochrome P450 2D6 (Merck 1998). Systemic absorption of amitriptyline is poor when administered transdermally as opposed to orally. Therefore, transdermal administration of amitriptyline is not recommended at this time (Mealey et al. 2004). The most common side effects in cats and dogs are sedation, miosis, and urinary retention. Weight gain, decreased grooming, and transient cystic calculi may also occur (Chew et al. 1998). A variety of cardiovascular, CNS, anticholinergic, hematologic, gastrointestinal, and endocrine side effects are reported in humans. Amitriptyline has teratogenic effects in mice and hamsters when pregnant females are given doses of 28–100 mg kg−1 day−1. In the rat, medicating pregnant females with 25 mg kg−1 day−1 results in delayed ossification in the fetal vertebrae. In rabbits, if pregnant females are medicated with 60 mg kg−1 day−1, ossification of the cranial bones is delayed. Amitriptyline crosses the placenta, and there have been some reports of adverse events in human babies when the mother was medicated with amitriptyline during pregnancy. However, there is insufficient documentation to determine if the amitriptyline was the cause of the adverse events. Amitriptyline is also excreted into breast milk. Because of the potential for adverse effects on fetuses or young, pregnant or lactating females should not be medicated with amitriptyline. The intravenous LD50 (the dose that kills 50% of the animals tested) is 18–22 mg kg−1 in mice and 6–11 mg kg−1 in rabbits. The oral LD50 is 286–359 mg kg−1 in rats and 100–216 mg kg−1 in mice (Ribbentrop and Schauman 1965). Toxic signs include respiratory depression, ataxia, tremors, convulsions, and prostration. There is no specific antidote for overdose of amitriptyline. Decontaminate and provide supportive therapy. Emesis is contraindicated. Lipid therapy may be beneficial in amitriptyline overdose (Kiberd and Minor 2012). Patients that have been on amitriptyline daily for several weeks should be withdrawn gradually. Amitriptyline has historically been commonly used in general practice for the treatment of anxiety disorders in dogs and cats, apparently initially for economic reasons and, later, because of familiarity with the medication. However, compared with other drugs such as fluoxetine and clomipramine, which have become much more economically feasible for the pet owner in recent years, amitriptyline has a relatively low clinical efficacy and high incidence of side effects. In a retrospective study, two of three cats with psychogenic alopecia that were treated with amitriptyline at doses of 2.5 mg q12h or 5.0 mg q24h (total dose) responded (Sawyer et al. 1999). Amitriptyline has been used successfully to treat hypervocalization in a cat (Houpt 1994). Amitriptyline (2 mg kg−1 orally [PO], daily) may decrease clinical signs of severe recurrent idiopathic cystitis in cats, possibly in part because of analgesic effects such as those that occur in human patients (Hanno et al. 1989; Chew et al. 1998). High doses of amitriptyline, 7–10 mg kg−1 intravenously (IV) result in loss of electroencephalogram changes in cats that are subjected to loud tones or pinching. Lower doses result in attenuation of the response (Vernier 1961). Dogs given amitriptyline at a dose range of 0.74–2.5 mg kg−1 q12h for ≥45 days do not exhibit any electrocardiogram (EKG) changes. P‐wave duration has a significant negative correlation with serum concentration of amitriptyline at clinically usual doses, but remains within normal parameters (Reich et al. 2000). In a retrospective study of 103 dogs with various presentations of compulsive disorder, amitriptyline was found to be significantly less effective than clomipramine (Overall and Dunham 2002). In a prospective, randomized, double‐blind, placebo‐controlled trial of treatment of canine aggression with amitriptyline plus behavior modification versus clomipramine plus behavior modification, amitriptyline was no more effective than placebo (Virga et al. 2001). One dog diagnosed with a combination of “dominance aggression” and food‐defense aggression responded positively to a combination of amitriptyline and behavior modification (Reich 1999). In an open trial, 15 of 27 dogs (56%) with separation anxiety that were treated with amitriptyline in addition to behavior modification improved (Takeuchi et al. 2000). Suspected neuropathic pain may be successfully treated with amitriptyline (Cashmore et al. 2009). Amitriptyline is rapidly metabolized in the horse. A single dose of 750 mg followed by collection of urine through a catheter during the zero‐ to three‐week period after administration revealed that almost all of the medication being excreted during this period was nortriptyline (Fenwick 1982). Clomipramine affects both the serotonergic and noradrenergic neural transmission in the CNS. The primary mechanism of action is probably prevention of reuptake of serotonin in the CNS, and it is the most serotonin‐specific of the commercially available TCAs (see Table 16.1). It is highly lipophilic and therefore passes easily through lipophilic membranes. The major route of biotransformation is demethylation, resulting in desmethylclomipramine. Subsequently, further metabolic processes produce various water‐soluble substances that are eliminated through the bile or the urine (Faigle and Dieterle 1973). In humans, the half‐life is 15–60 hours (Nelson 2004). In the dog, it is almost totally absorbed when given orally. In the dog and the rat, the main mode of excretion is through the bile, with the dog eliminating about 80% of an oral or intravenous dose of 5 mg kg−1 of clomipramine via the bile within four days, most of the remainder being excreted via the kidneys in the same amount of time. In humans, more clomipramine is excreted via the kidneys than the bile (Faigle and Dieterle 1973). Following intravenous injection, clomipramine is rapidly distributed throughout the body, penetrating various tissues and organs, as demonstrated by whole‐body autoradiography performed on mice given 10 mg kg−1. High concentrations initially occur in the lung, adrenal gland, thyroid, the kidney, the pancreas, the heart, and the brain, which would be predicted based on clomipramine’s lipophilic nature. The affinity of clomipramine for tissues containing fat results in rapid decreases in blood levels (Faigle and Dieterle 1973). In humans, there is no relation between dose and plasma level of clomipramine, but plasma concentrations of desmethylclomipramine, the primary active metabolite, are correlated with dose (Jones and Luscombe 1977). After both single‐dose and multiple‐dose oral treatment of dogs with clomipramine, peak concentrations of clomipramine occur in the plasma within three hours, while peak concentrations of the primary active metabolite, desmethylclomipramine, usually occur within four to six hours. Subsequently, plasma levels decline rapidly, with a plasma half‐life for clomipramine of about four hours. However, there is a substantial range in elimination half‐life, and it can be as great as 16 hours. The measured plasma half‐life for desmethylclomipramine is likewise about four hours, but since this is a combination of the interaction between generation of new desmethylclomipramine as clomipramine is metabolized, and elimination of desmethylclomipramine, the actual half‐life of desmethylclomipramine is shorter. With intravenous administration in dogs, the mean elimination half‐life is five hours (Hewson et al. 1998a; King et al. 2000a, 2000b). The half‐life in humans is longer, with a mean of about 20 hours when given orally (Nagy and Johansson 1977; Evans et al. 1980). In dogs, plasma concentrations of clomipramine are higher than concentrations of desmethylclomipramine (about 3 : 1), which is the opposite of humans, in which plasma concentrations of clomipramine are lower than those of desmethylclomipramine (about 1 : 2.5) (Broadhurst et al. 1977; Jones and Luscombe 1977; Kuss and Jungkunz 1986; Hewson et al. 1998a; King et al. 2000a; King et al. 2000b). This may be one of the reasons that adverse events appear to be less frequent in dogs than in humans, since clomipramine is the molecule that acts predominantly on serotonin whereas desmethylclomipramine has stronger anticholinergic activity (Benfield et al. 1980). When dogs are dosed daily with clomipramine, steady‐state plasma levels are achieved within four days (King et al. 2000a). In vitro, cat microsomes transform clomipramine more slowly than do rat or dog microsones. The cat also exhibits a gender difference, with male cat microsomes being less efficient demethylators and hydroxylators than female cat microsomes (Lainesse et al. 2007b) In a study of the pharmacokinetics of clomipramine in six adult spayed cats, the mean half‐life of clomipramine after administration of a single IV dose of 0.25 mg kg−1 was 12.3 hours, with a range of 7.7 hours to 18.7 hours (Lainesse et al. 2006) Seventy‐six spayed and neutered cats given a single dose of clomipramine in the dose range of 0.32 to 0.61 mg kg−1 orally had peak levels of clomipramine occur at one to six hours, with a mean of three hours, while peak doses of the active metabolite desmethylclomipramine occurred at 1 to 24 hours, with a mean of seven hours. Females had a significantly faster Cl F−1 (0.36 l h−1 kg−1) than males 0.21 l h−1 kg−1) and a significantly higher mean MR (0.53 compared to 0.36) (Lainesse et al. 2007a). Normal dogs treated with 3 mg kg−1 clomipramine daily, PO, have a lower ratio of 5‐hydroxyindoleacetic acid (HIAA) to 3‐methyl 4‐hydroxyphenylglucol (MHPG) in the cerebrospinal fluid than do dogs treated with placebo (Hewson et al. 1995). Desmethylclomipramine has anticholinergic effects on gastrointestinal smooth muscle, inhibiting motility and antagonizing muscarinic receptors, but does not do so as much as clomipramine. It is a more potent inhibitor of norepinephrine and dopamine reuptake than clomipramine. It has antidepressant activities that are probably due to its monoamine uptake inhibition (Benfield et al. 1980). There is a faster rate and higher levels of absorption in dogs that are fed than in dogs that are fasted. Overall bioavailability is about 25% greater in dogs that are fed as opposed to fasted. Plasma half‐life is 2 to 9 hours in fed dogs, but 3 to 21 hours in fasted dogs, presumably due to delayed absorption (Novartis 2000; King et al. 2000b). When dogs are repeatedly dosed, the half‐lives of clomipramine and desmethylclomipramine increase with increased dosage. At doses of 1, 2, and 4 mg kg−1 twice a day (b.i.d.), the accumulation ratios for clomipramine are 1.4, 1.6, and 3.8, respectively, while for desmethylclomipramine they are 2.1, 3.7, and 7.6. There are two main possibilities for this observation. First, the main route of elimination of both clomipramine and desmethylclomipramine may be saturable. Second, the increasing numbers of molecules may themselves directly inhibit the elimination process (King et al. 2000a). In dog cells, clomipramine inhibits P‐glycoprotein, a multidrug transporter that removes toxins and certain other molecules from cells (Schrickx and Fink‐Gremmels 2014). Clomipramine is used to treat obsessive‐compulsive disorder in humans. Clomipramine should not be given to patients with a history of sensitivity to clomipramine or other TCAs. It should not be given in conjunction with an MAOI or within two weeks of discontinuation of administration of an MAOI. Avoid or use cautiously in patients with a history of epilepsy, cardiac arrhythmias, glaucoma, or urine or stool retention. While clomipramine is not as cardiotoxic in dogs as it is in humans, sufficiently large doses can induce cardiotoxicity. In a study in which anesthetized dogs were given a continuous intravenous infusion of clomipramine until cardiotoxicity occurred, toxic effects were observed at an average of 65 mg kg−1 (range 53–72). This is much higher than the dose at which cardiotoxicity occurred in dogs infused with amitriptyline (Lheureux et al. 1992a). Clomipramine should not be given to male breeding dogs, as testicular hypoplasia may occur (Novartis 2000). In humans, concurrent administration of phenobarbital with clomipramine results in increased plasma levels of clomipramine. Side effects include sedation, mydriasis, regurgitation, appetite changes, and urinary retention (Pfeiffer et al. 1999; Litster 2000). Clomipramine may also potentiate the side effects of various CNS depressants, including benzodiazepines, barbiturates, and general anesthetics. In humans, on which there is much more data than in the veterinary population, a broad spectrum of side effects has been reported, including cardiovascular effects, mania, hepatic changes, hematologic changes, CNS disorders, sexual dysfunction, and weight changes (Novartis 1998). Rats given approximately five times the maximum daily human dose exhibited no impairment of fertility, while rats given up to 20 times the maximum daily human dose exhibited no clear evidence of carcinogenicity of clomipramine. A rare tumor, hemangioendothelioma, did occur in a small number of the rats. When pregnant rats and mice were given up to 20 times the maximum daily human dose, there were no teratogenic effects, although there was some evidence of fetotoxic effects. Clomipramine does enter the milk (Novartis 1998). Use in pregnant and nursing females should be avoided if possible. There is no specific antidote for overdose with clomipramine. Decontaminate and provide supportive therapy. Emesis is contraindicated. Animals that have been given clomipramine daily for several weeks should be withdrawn gradually. Cats given up to five times the clinical dose for 28 days exhibited mild sedation, occasional pupillary dilatation, and a slight decrease in food consumption (Landsberg 2001). Cats given 10 mg day−1 of clomipramine did not exhibit any significant changes in their electrocardiogram. Cats given 10 mg day−1 of clomipramine for 28 days did exhibit some decreases in total thyroxine (T4), triiodothyronine (T3), and free throxine (fT4), specifically 25%, 24%, and 16% serum values, respectively. This effect could lead to a misdiagnosis of euthyroidism in cats with subclinical hyperthyroidism (Martin 2010). In an open trial of cats with various anxiety‐related and compulsive disorders, six cases of urine spraying, three cases of over‐grooming, and one case of excessive vocalization resolved or were substantially improved when treated with clomipramine at a dose of 0.2–0.55 mg kg−1 daily, combined with behavior modification. Some cats became sedated at the higher dose range, but were successfully treated when the dose was lowered (Seksel and Lindeman 1998, 1999). In a double‐masked clinical trial of spraying cats, clomipramine at 0.5 mg kg−1 q24h was found to be equally effective as fluoxetine given at 1 mg kg−1 q24h (Hart et al. 2005). In a single‐blind trial Dehasse (1997) reported a 75% decrease in the number of urine‐spraying incidents in 80% of cats given 5 mg day−1 of clomipramine as opposed to when they were on placebo. A few of the cats were mildly sedated while on medication. Landsberg (2001) and Landsberg and Wilson (2005) likewise have had improvement in over 80% of spraying cats given 0.5 mg kg−1 for one month. Six of 25 cats, or about a quarter of the patients, became calmer, friendlier and more affectionate while on clomipramine. The most common side‐effects were increased sleep and lethargy, decreased appetite and anticholinergic effects, including decreased frequency of urination or defecation, and dry mouth (Landsberg and Wilson 2005). In a meta‐analysis of the use of clomipramine as a treatment for urine spraying in cats, Mills et al. (2011) found a significant association between clomipramine use and the number of cats that ceased urine spraying or decreased the behavior by 90%. Clomipramine has also been used to successfully treat cats with psychogenic alopecia. In a retrospective study, five of five cats treated with clomipramine at doses of 1.25–2.5 mg (total dose) q24h responded (Sawyer et al. 1999). In a prospective, double‐blind, placebo‐controlled, randomized trial, clomipramine, at a dose of 0.5 mg kg−1 q12h PO was more effective than placebo in the treatment of feline psychogenic alopecia (Mertens and Torres 2003; Mertens et al. 2006). A cat with psychogenic alopecia manifesting as mutilation of the tail responded with a treatment of clomipramine at 0.5 mg kg−1 daily, combined with behavior modification and environmental changes for two months. The cat had previously had a partial caudectomy because of the self‐mutilation of the tail. The caudectomy failed to resolve the problem (Talamonti et al. 2017). Of 14 cats treated with clomipramine for a variety of anxiety‐related behavior problems, including spraying (12 cats), tail‐chasing (one cat), nocturnal vocalization (one cat), and aggression to the owner (one of the cats that sprayed), the problem resolved in six cats and was improved in the remaining eight cats. The total daily dose ranged from 0.4 to 1.32 mg kg−1 (Litster 2000). The most definitive results on the efficacy of clomipramine in the treatment of urine spraying in cats were obtained in a randomized, double‐blind, placebo‐controlled, multicenter clinical trial. Sixty‐seven neutered cats were treated with placebo, 0.125–0.25 mg kg−1 daily (low dose), 0.25–0.5 mg kg−1 daily (moderate dose), or 0.5–1.0 mg kg−1 daily (high dose) for three months. Various other treatments had been tried previously and been unsuccessful, including pheromones (17 cats), amitriptyline (7), buspirone (2), diazepam (4), megestrol acetate (12), progestogens (4), and corticosteroids (1). At all doses, clomipramine was more effective than placebo. The moderate and high doses were more effective than the low dose. There was no effect of age, sex, whether or not previous attempts had been made to treat the urine spraying and whether or not the cat lived in a single‐cat household versus a multicat household. Aggression toward familiar cats, unfamiliar cats, and animals other than cats was significantly decreased in the high‐dosage group. During the third month of treatment, the amount of time spent in stereotypic behaviors other than licking or grooming was also significantly decreased in both the moderate and high‐dosage groups as compared with the low‐dosage group. Sedation was the most common side effect and always occurred during the first month of treatment. The frequency and severity of sedation were dose related. However, the overall behavior patterns were not changed, and it is possible that some of what the owners were reporting as sedation was simply the consequence of the cat being less confrontational and more relaxed with its housemates. This possibility requires further study (King et al. 2004a). The substantial variation in rate of metabolism of clomipramine in cats, discussed in the Clinical Pharmacology section, may explain some of the variation in clinical response to this medication (Lainesse et al. 2007a; Lainesse et al. 2007b). In humans, only moderate overdoses of clomipramine are cardiotoxic, with such sequelae as increased heart rate, decreased blood pressure, and slow intracardiac conduction. Research conducted on dogs has demonstrated that this drug is more benign in this species. Dogs given 20 mg kg−1 daily for seven days, which is five times the maximum recommended label dose, exhibited a significant reduction in heart rate, with the peak effect occurring about 12 hours after medication. Doses of 4 or 12 mg kg−1 of clomipramine did not induce any changes in heart rate. There were no significant changes in the electrocardiogram (EKG) (Pouchelon et al. 2000). In another study, canine patients given clomipramine at doses of 1.5–2.49 mg kg−1 q12h for ≥45 days did not exhibit EKG changes. Duration of the P‐wave significantly positively correlates with serum concentration of clomipramine, but in studies to date has remained within the clinically normal range for dogs given clinically appropriate doses (Reich et al. 2000). In healthy dogs given 3 mg kg−1 of clomipramine q12h for 112 days, there are significant decreases in total thyroxin (T4), free thyroxin (fT4), and 3, 3′, 5′–triiodothyronine (reverse T3, rT3). T4 decreased 35% while fT4
Tricyclic Antidepressants
Action
TCA
NE
5‐HT
α‐1
α‐2
H1
Musc
Amitriptyline
±
++
+++
±
++++
++++
Clomipramine
+
+++
++
0
+
++
Desipramine
+++
0
+
0
0
+
Doxepin
++
+
++
0
+++
++
Imipramine
+
+
+ +
0
+
++
Nortriptyline
++
±
+
0
+
++
Overview of Indications
Contraindications, Side Effects, and Adverse Events
Adverse Drug Interactions
Overdose
Discontinuation
Clinical Guidelines
Specific Medications
TCA
Cat
Dog
Amitriptyline
0.5–2.0 mg kg−1 q12–24 h
1–6 mg kg−1 q12h
Clomipramine
0.25–1.3 mg kg−1 q24h
1.0–3.0 mg kg−1 q12h
Desipramine
1.5–3.5 mg kg−1 q24h
Doxepin
0.5–1.0 mg kg−1 q12h
3.0–5.0 mg kg−1 q8–12 h
Imipramine
0.5–1.0 mg kg−1 q12–24 h
0.5–2.0 mg kg−1 q8–12 h
Nortriptyline
0.5–2.0 mg kg−1 q12–24 h
1.0–2.0 mg kg−1 q12h
Animal
Dose
Parrots
Clomipramine
2.0–4.0 mg kg−1 q12h
Doxepin
0.5–5.0 mg kg−1 q12h
Horses
Imipramine
0.75–2.0 mg kg−1
I. Amitriptyline
Clinical Pharmacology
Uses in Humans
Contraindications
Side Effects
Overdose
Discontinuation
Other Information
Effects Documented in Nonhuman Animals
Cats
Dogs
Horses
II. Clomipramine Hydrochloride
Clinical Pharmacology
Uses in Humans
Contraindications
Side Effects
Overdose
Discontinuation
Effects Documented in Nonhuman Animals
Cats
Dogs
Stay updated, free articles. Join our Telegram channel

Full access? Get Clinical Tree
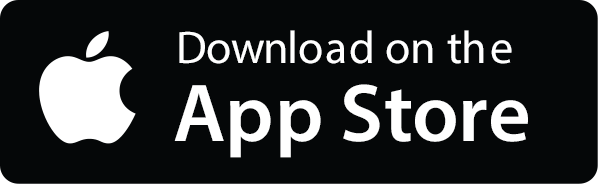
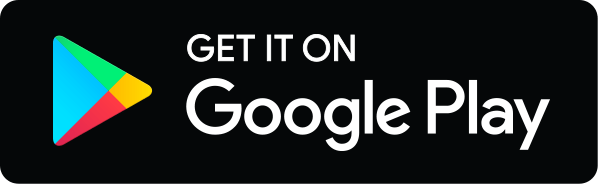