Benjamin M. Brainard Department of Small Animal Medicine and Surgery, College of Veterinary Medicine, University of Georgia, Athens, Georgia, USA Interest in the process of both normal and disordered hemostasis in the veterinary patient has experienced resurgence in recent years. This is due in part to the availability of newer tests and techniques that can better illustrate the process and products of coagulation. At the same time, new understanding of the formation of clots in vivo has made it clear that traditional testing provides only a limited view into the actual mechanisms and pathophysiology of clot formation and platelet function. The cell‐based model of hemostasis provides a framework for understanding the complex interactions between cellular components, soluble factors, and the vascular endothelium in the formation of a clot. In some cases, this model explains why certain conditions (e.g., factor deficiencies) have a particular hemostatic phenotype. Coagulation as described by this model consists of three phases: initiation, amplification, and propagation [1]. The vast majority of coagulation in vivo occurs as a result of tissue factor (TF, factor III) initiation of the extrinsic coagulation cascade (as opposed to contact activation via the intrinsic coagulation cascade [also termed the “contact‐activation pathway”], which may occur on the surfaces of foreign materials like intravenous catheters) [2]. TF is exposed following intimal injury of a blood vessel and may also be present on the surface of circulating monocytes and microparticles, especially in the setting of systemic inflammation. Microparticles are small membrane blebs released from activated endothelial cells, platelets, or leukocytes. The other important player in the initiation of coagulation is the platelet. Following activation, the platelet undergoes a shape change, and anionic phospholipids such as phosphatidyl serine are exposed on the outer platelet membrane. These phospholipids provide a surface for the assembly of coagulation factors into complexes that promote clot amplification [3]. In the initiation step, exposed TF comes into contact with activated factor VII (fVIIa) and activated factor X (fXa), forming a TF‐fVIIa‐fXa complex [1]. A small amount of thrombin (factor IIa) is generated by this complex. The small amount of thrombin released in the initiation step promotes the amplification stage, where additional platelets and coagulation factors V, VIII, and XI are activated [2]. Thrombin is generated in the propagation phase from complexes of these factors assembled on cell membranes. The tenase complex, composed of factors IXa, VIIIa, and calcium, activates coagulation factor X. The presence of factor Xa then allows formation of the prothrombinase complex, which is composed of factors Xa, Va, and calcium. The prothrombinase complex is an extremely efficient complex that promotes the formation of thrombin (factor IIa) from prothrombin, which then catalyzes the formation of fibrin from fibrinogen [4]. Endogenous coagulation inhibitors are present at each stage of the coagulation process to prevent excessive thrombin formation. Tissue factor pathway inhibitor (TFPI) is a potent inhibitor of the TF‐fVIIa‐Xa complex [1]. Antithrombin acts to inhibit factors Xa and IIa. The protein C/protein S system supports the inhibition of factors Va and VIIIa. Patients with urinary losses of antithrombin and humans with congenital low concentrations of protein C are thought to have an increased risk for the development of thromboembolism. Dogs with sepsis have decreased activities of protein C and antithrombin [5]. Dogs with sepsis have also been reported to have increased thrombin‐activatable fibrinolysis inhibitor (TAFI) activity [6]. TAFI is activated in the presence of thrombin and acts to decrease the speed of fibrinolysis or clot breakdown. The complex in vivo physiology of clot formation is very difficult to mimic in vitro. Most laboratory tests of coagulation function are focused on individual aspects of the coagulation process. Platelet function may be assessed using tools such as optical or impedance aggregometry, which document the response of platelets (in platelet‐rich plasma or whole blood, respectively) to discrete agonists such as adenosine diphosphate (ADP), collagen, or arachidonic acid [7]. Most aggregometry testing is performed under low shear settings, which do not necessarily reflect the in vivo physiology of the high‐shear condition of flowing blood. The PFA‐100 (Siemens‐Dade Behring) is a whole blood test that aspirates blood through a small aperture using a vacuum [8]. The aperture is coated with a combination of either epinephrine and collagen or ADP and collagen. As platelets pass through the aperture, they are activated by the agonists and form a plug, stopping the flow of blood. The time it takes for this to occur is termed the “closure time” and is measured in seconds. In dogs with von Willebrand disease (vWD, which impairs the ability of platelets to tether and adhere to sites of vascular injury) and in animals treated with drugs that decrease platelet activity, the closure time is prolonged [9]. This is a rapid, benchtop test that can be used to assess an animal’s risk of bleeding due to platelet function abnormalities. Low hematocrits and platelet counts less than 90 × 109 platelets/L may cause prolonged closure times unrelated to platelet function. Buccal mucosal bleeding time (BMBT) is another test that can diagnose platelet function abnormalities. It is simple and can be done rapidly as part of a presurgical screen. To perform this test, the buccal mucosa is exposed and a standard incision (5 mm long and 1 mm deep) is made using a SimPlate® device [10]. Once the incision is made, the area is observed for the formation of a gel‐like platelet plug. The bleeding time is defined as the time until bleeding has ceased and is between 3–5 min in normal animals. Because cats have a small buccal surface, the oral mucosal bleeding time (OMBT) is performed instead, by making the incision in the gums above the upper canine tooth [11]. Cats generally need to be sedated or anesthetized for this test. As with the PFA, the BMBT will be prolonged if patients have thrombocytopenia (< 75 × 109 platelets/L). Assessment of coagulation factor activity is generally performed using citrated plasma but may also be assessed in whole blood systems. Most tests monitor for the formation of a fibrin clot by exposing citrated plasma to calcium in combination with an activating substance and report the time until clot formation (which may be sensed optically or mechanically) as the clotting time [12]. The prothrombin time (PT) is initiated using a mixture of TF and phospholipid and primarily assesses the extrinsic and common pathway of coagulation (i.e., factors VII, X, V, II). Activated partial thromboplastin time (aPTT) is initiated with a strong contact activator such as kaolin and reflects activity in the intrinsic and common pathways (i.e., factors XII, XI, IX, VIII, X, V, II). The thrombin time is dependent on the blood fibrinogen concentration and assesses only the formation of fibrin in the common pathway following the addition of thrombin. Modifications of both PT and aPTT have been developed to use citrated whole blood for coagulation testing as well [13]. The activated clotting time (ACT) adds whole blood to diatomaceous earth (a strong contact activator) and measures the time until it forms into a clot. The reference intervals for coagulation times of normal animals vary depending on methodology and technique. Viscoelastic coagulation testing using techniques such as thromboelastography (TEG), rotational thromboelastometry (ROTEM), or Sonoclot use citrated whole blood to evaluate both primary and secondary hemostasis, in addition to fibrinolysis [14]. Viscoelastic testing displays the change in viscosity as blood gels into a clot and can identify both hypocoagulable and hypercoagulable states. Transfusion algorithms based on intraoperative viscoelastic monitoring have resulted in more targeted transfusion strategies and decreased waste of blood products in human medicine [15]. Viscoelastic techniques have been described in dogs, cats, and horses [16–18]. In human medicine, arterial thromboses are thought to result from activation or hyperactivity of platelets, while venous thrombosis or thromboembolism is more associated with blood stasis and activation of coagulation factors [19]. While these two pathophysiologic consequences dictate different treatment approaches, the reality in veterinary medicine balances the need for patient safety and ease of administration. For this reason, most anticoagulants used in veterinary medicine are antiplatelet agents that can be administered orally. Injectable anticoagulants that target the coagulation cascade are also used, especially in hospitalized patients, and newer oral anticoagulants may provide a safer approach to providing tailored long‐term anticoagulation in veterinary patients. Platelet activation results in a series of events that allow the platelet to adhere to sites of vascular damage (tethered by molecules such as von Willebrand Factor and collagen) and also cause the release of substances that support coagulation and activate adjacent platelets. Molecules released from activated platelets include ADP, thromboxane, serotonin, and calcium. Because there are specific receptors for many of these agonists, antiplatelet agents have been designed to affect the binding or release of these agonists. Aspirin (acetylsalicylic acid, ASA) decreases platelet responsiveness and has been evaluated in most domestic species. Aspirin exerts an antiplatelet effect by irreversible inhibition of platelet cyclo‐oxygenase (COX), which produces thromboxane A2 (TXA2) from arachidonic acid in response to platelet activation [20,21]. TXA2 is a potent vasoconstrictor and platelet activator, although platelet responses vary between species and individuals. In dogs, aspirin at various doses has been recommended to decrease platelet activity in patients with immune‐mediated hemolytic anemia (IMHA) and other diseases that are associated with hypercoagulable states (e.g., protein‐losing nephropathy) [5]. In many of these cases, a low dose of aspirin (0.5–1.0 mg/kg PO q 12 h) has been recommended, although the efficacy of these lower doses on platelet aggregation is unclear [22]. Interestingly, certain breeds or families of dogs may not be as responsive to aspirin‐mediated platelet inhibition [23]. The same may be true in humans, and a syndrome of “aspirin resistance” has been described [24]. In cats, aspirin has been recommended for thromboprophylaxis in animals with hypertrophic cardiomyopathy, although efficacy for inhibition of platelet aggregation or for prevention of intracardiac thrombus formation has not been definitively demonstrated [21,25]. Aspirin has been used to treat horses with jugular venous thrombosis. Other veterinary‐labeled non‐steroidal anti‐inflammatory drugs may cause transient impairment of platelet function in dogs, which is unlikely to cause clinically significant perioperative bleeding unless used in patients with other comorbidities that decrease platelet function (e.g., vWD). Clopidogrel (Plavix®, Sanofi Synthelabo, New York) is an irreversible antagonist of the platelet P2Y12 receptor, one of two ADP receptors on the platelet surface. In dogs, cats, and horses, clopidogrel effectively decreases platelet aggregation in response to ADP [11,26,27]. Clopidogrel is a prodrug, requiring hepatic biotransformation to an active compound. Co‐administration of drugs that affect the hepatic P450 enzyme system may alter the pharmacokinetics of clopidogrel; however, recent studies do not indicate a clinically relevant interaction with proton pump inhibitors in dogs [28]. As with aspirin, there are human patients who do not demonstrate decreased platelet aggregation following clopidogrel therapy. The mechanism may be altered hepatic processing or variations of platelet receptor affinity, and this phenomenon likely occurs in veterinary species as well [29,30]. Because both aspirin and clopidogrel result in irreversible inhibition of platelet function, it is necessary for patients to release new platelets to regain platelet function. In animals with normal bone marrow function, a new platelet population is generally present 5–7 days after cessation of antiplatelet medications, although clinical response to platelet agonists may return prior to this point. Aspirin and clopidogrel are frequently prescribed for veterinary patients diagnosed with hypercoagulable conditions because they are generally well tolerated, easily administered at home, and not associated with adverse events such as hemorrhage when used individually. When clopidogrel and aspirin were used together in human patients, the antithrombotic effects were more pronounced, but there was also an increased incidence of gastrointestinal bleeding [31]. Warfarin is an oral vitamin K epoxide reductase antagonist that prevents the hepatic recycling of vitamin K, resulting in decreased coagulation factor production. It impairs the production of vitamin K‐dependent coagulation factors (i.e., II, VII, IX, and X) [32]. Warfarin is difficult to titrate. Clinical use requires initial weekly measurements of PT, a tightly regulated diet, and a strict dosing schedule to minimize the chances for hemorrhage. Warfarin therapy has been used as a component of medical management in dogs with venous thrombi [33] and was also utilized in a small number of cats with hypertrophic cardiomyopathy. However, in those cats, its use was associated with a shorter survival time [25]. The effects of warfarin can be antagonized using either oral vitamin K (phytonadione) therapy or by transfusions of fresh frozen or stored plasma [34]. Despite the fact that warfarin is administered orally, it is not frequently used in veterinary medicine due to the potential severity of complications and difficulty in ensuring owner compliance. Other oral anticoagulant medications such as rivaroxaban (Xarelto®, Bayer Healthcare) and apixaban (Eliquis®
31
Treatment of Coagulation and Platelet Disorders
Introduction
The cell‐based model of hemostasis
Coagulation and platelet function testing
Platelet function testing (primary hemostasis)
Coagulation testing (secondary hemostasis)
Anticoagulant therapy
Antiplatelet drugs
Coagulation inhibitors
Stay updated, free articles. Join our Telegram channel

Full access? Get Clinical Tree
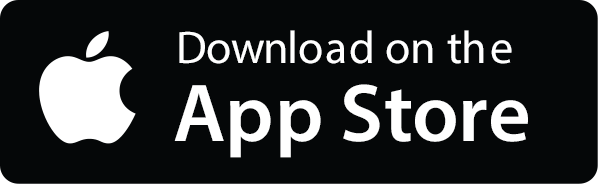
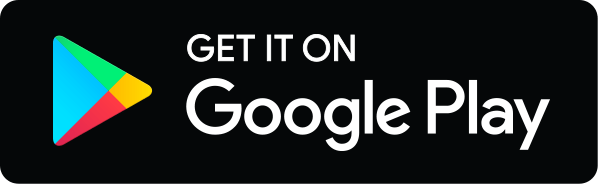