Chapter 11
Transfusion-Associated Complications
Shauna L. Blois
Department of Clinical Studies, Ontario Veterinary College, University of Guelph, Guelph, Ontario, Canada
Introduction
Blood transfusion is an essential part of therapy for critical patients. Commercial veterinary blood banks have made blood products accessible to many clinics. While transfusion of blood products is often a life-saving measure, this procedure is not without risk. Increasing use of blood products has led to increasing awareness of transfusion reactions in veterinary medicine. Additionally, new complications associated with transfusion, such as immunomodulation, are being identified in human medicine and such complications could have similar impact on veterinary patients. Novel transfusion strategies such as pre-storage leukoreduction and transfusion of fresh versus older blood products are being explored in human medicine to help mitigate transfusion-associated complications. While the benefits of these strategies are controversial, they are also increasingly investigated in veterinary medicine. The importance of complications associated with transfusion, efforts to prevent such reactions, and treatment of life-threatening transfusion reactions are further discussed in this chapter.
Transfusion reactions are likely under-recognized, but are reported to occur in 3.3–28% of canine patients and 1.2–8.7% of feline patients receiving blood products (Kerl and Hohenhaus 1993; Callan et al. 1996; Castellanos and Gray 2004; Weingart et al. 2004; Klaser et al. 2005; Holowaychuk et al. 2014). Immunologic transfusion reactions are caused by antigen–antibody reactions between cellular or protein content within the donor blood product and the recipient’s immune system, and include febrile, hemolytic, and allergic reactions. Non-immunologic transfusion reactions are caused by various mechanisms, including circulatory overload, improper collection or transfusion technique, transmission of infectious diseases, and bacterial contamination. Acute transfusion reactions can be detected within 48 hours of transfusion, but are usually apparent within the first 1–2 hours of starting the transfusion. Delayed transfusion reactions are often less severe than acute reactions and occur 2–5 days or longer after transfusion. Common transfusion reactions in veterinary patients are outlined in Table 11.1.
Table 11.1 Causes, clinical signs, and treatment of common transfusion reactions in dogs and cats
Reaction | Cause(s) | Clinical signs | Treatment | |
Immunologic | Febrile non-hemolytic | Residual donor WBCs, platelets cytokines in blood product | Body temperature increase >1°C after onset of transfusion Typically no other clinical signs | Slow (or discontinue) transfusion Active cooling when temperature >40°C; discontinue once <39.5°C to avoid hypothermia Antipyretics (e.g., dipyrone), if severe (>41.5°C) and not responsive to above therapy |
Acute hemolytic | Antigen–antibody hypersensitivity (e.g., DEA 1 exposure in a previously sensitized dog or any AB mismatched transfusion in cats) | Tachycardia, tachypnea, restlessness, fever Hemoglobinemia/hemoglobinuria | Stop transfusion Fluid resuscitation to maintain arterial blood pressure (mean >70–80 mmHg) Glucocorticoid (unproven efficacy) Epinephrine if needed Vasopressors if needed | |
Allergic | Immunoglobulin E-mediated immunologic reaction | Local or generalized pruritus, urticaria, angioedema in mild to moderate cases Generalized reactions can also show signs of shock or anaphylaxis (e.g., hypotension, respiratory distress, vomiting) | Mild to moderate local reaction:
Moderate to severe, generalized reaction:
| |
Delayed hemolytic | Low titer of alloantibodies (natural or previously sensitized) Induction of alloantibodies in unsensitized dog | Typically no clinical signs observed Unexpected decrease in patient hematocrit or packed cell volume | Usually none required | |
Non-immunologic | Non-immunologic hemolysis | Improper collection, storage, or transfusion technique (e.g., inappropriate fluid pump used) | Tachycardia, tachypnea, restlessness, fever Hemoglobinemia/hemoglobinuria | Stop transfusion As above for immunologic hemolytic reactions |
Circulatory overload | Rapid transfusion rates/large transfusion volume Patients with cardiac disease, kidney insufficiency, or chronic anemia | Tachypnea, orthopnea, dyspnea, cyanosis. | Stop transfusion Furosemide | |
Hypocalcemia | Large volume transfusion (citrate overload) | Tremors, muscle twitching, tetany, facial rubbing, restlessness, panting | Calcium gluconate supplementation if hypocalcemia (i.e., ionized Ca2+ <1.0 mmol/L) or if clinical signs seen | |
Sepsis | Bacterial contamination of blood product | Fever, tachycardia, tachypnea, hypotension Hemoglobinemia/hemoglobinuria Similar clinical signs as acute hemolytic reactions | Stop transfusion Broad-spectrum antibiotics pending culture/sensitivity results Fluid resuscitation and other support as needed |
Transfusion-associated or transfusion-related complications, especially acute lung injury (ALI), circulatory overload, and hemolytic reactions, are associated with increased mortality rate in humans (Gilliss et al. 2011; Hirayama 2013). However, transfusion-associated complications were not linked with increased risk of death in retrospective studies of dogs receiving packed red blood cell (PRBC) transfusions (Kerl and Hohenhaus 1993; Holowaychuk et al. 2014). Nevertheless, these complications impact the clinical status of affected patients and often require immediate intervention. Increased awareness of transfusion reactions allow for their rapid detection and management.
Immunologic transfusion reactions: acute
Febrile, non-hemolytic reactions
Febrile, non-hemolytic reactions are the most commonly described transfusion reactions in cats and dogs. These reactions are characterized by a temperature increase of >1°C during or immediately after transfusion. Other clinical signs can include vomiting and tremors. Most febrile, non-hemolytic reactions are mild and self-limiting. The pathogenesis of these reactions is likely multifactorial. Transfused blood products contain residual white blood cells (WBCs) from the donor. Circulating antibodies in the recipient react with the transfused WBCs, inducing release of cytokines and other mediators from the donor WBC. Blood products also contain biological response modifiers such as lipids, complement protein, and cytokines that induce a febrile response in the recipient (Blumberg et al. 2006; Fung and Heddle 2013). Fever can also be the first sign of an acute hemolytic transfusion reaction or sepsis, therefore any patient with a fever at the onset of a transfusion should be carefully monitored for the development of more serious clinical signs.
White blood cells, and in some instances platelets, are removed from blood products by passing whole blood through a leukoreduction filter (McMichael et al. 2010). Leukoreduction filtration is usually performed shortly after collection (“pre-storage”), but can also be performed immediately prior to transfusion (“post-storage”). Because >99% of WBCs are removed from the blood product, pre-storage leukoreduction abrogates in vitro production of cytokines (Corsi et al. 2014). Leukoreduction causes a significant decline in the number of febrile non-hemolytic transfusion reactions in humans (King et al. 2004; Paglino et al. 2004; Yazer et al. 2004). The effect of leukoreduction on inflammation caused by blood transfusion has been studied in healthy dogs. In one canine study, leukoreduction mitigated the inflammatory response caused by autologous transfusion when compared to transfusion of non-leukoreduced blood (McMichael et al. 2010). However, the magnitude of inflammation was similar after transfusion of leukoreduced versus non-leukoreduced blood in another study (Callan et al. 2013). Further study of the clinical benefits of leukoreduction in veterinary patients is needed.
Acute hemolytic reactions
Acute hemolytic transfusion reactions result from type II hypersensitivity (antigen–antibody) reactions, due to the presence of antibodies in recipient plasma against donor red blood cell (RBC) antigens. Antibodies (primarily immunoglobulin (Ig)M, also can include IgG) adsorb to the donor RBC surface and can activate the complement cascade. This results in intravascular hemolysis of transfused RBCs during or within 24 hours of transfusion. Extravascular hemolysis can also occur if complement activation is minimal or absent (Massey et al. 2013). Clinical signs range in severity and include fever, vomiting, defecation, tachypnea, hemoglobinuria, hemoglobinemia, tachycardia, recumbency, dyspnea/apnea, hypotension, and other signs of shock. Acute kidney injury, disseminated intravascular coagulation, and death are potential sequelae (Auer et al. 1982; Giger and Bücheler 1991).
Cats have naturally occurring alloantibodies to their non-self blood type; type A cats will have alloantibodies to the B blood group and vice versa (Bücheler and Giger 1993). In particular, type B cats can have strongly agglutinating and hemolyzing anti-A antibodies. Therefore, type B cats can have severe hemolytic reactions within minutes of transfusion of a small amount of type A blood (Auer et al. 1982). Transfused type A RBCs administered to type B cats have an average half-life of 1.3 hours, with all type A RBCs destroyed within 24 hours. Reports of type A cats receiving type B blood document relatively less severe hemolytic reactions, but this depends on the anti-B titer of the recipient. The average half-life of type B RBCs transfused to type A recipients is 2 days. In contrast, the half-life of type-matched transfused RBCs ranges from 29 to 39 days in cats (Giger and Bücheler 1991). Due to the presence of naturally occurring alloantibodies in cats and the potential for severe, fatal transfusion reactions, it is imperative that all cats are blood typed prior to transfusion and that type-matched blood is always administered.
Dog erythrocyte antigen (DEA) 1 is considered the most antigenic and clinically relevant canine blood type, as it usually causes the most serious alloantibody-mediated transfusion reactions (Hale 1995). In recent years, this blood group system has undergone nomenclature changes. Previously, the DEA 1 blood group system described at least two subgroups: DEA 1.1 and DEA 1.2. A third type, DEA 1.3 (A3), was also described, but could not be verified due to lack of reagent availability (Symons and Bell 1991; Andrews et al. 1992; Hale 1995). Quantitative flow cytometry research has since discovered a large variation in DEA 1 expression among DEA 1 positive dogs. Dogs previously classified as DEA 1.2 positive are instead thought to have weak DEA 1 expression. Current clinical recommendations are to classify dogs as DEA 1 positive or negative; DEA 1 positive dogs should also be classified based on the strength of their DEA reaction (weak to strong) according to the blood-typing method used (Acierno et al. 2014).
Dogs do not have naturally occurring alloantibodies to DEA 1, but can develop them after a DEA 1 mismatched transfusion. The expected half-life of transfused RBCs ranges from 43 to 104 days in dogs (Novinger et al. 1996; McDevitt et al. 2011). Previous sensitization of a DEA 1 negative dog to DEA 1 positive blood can lead to an acute hemolytic reaction upon subsequent transfusion of DEA 1 positive blood (Giger et al. 1995). It is unknown if dogs with weak DEA 1 expression will have a transfusion reaction to blood with strong DEA 1 expression. Current clinical recommendations are to transfuse weak DEA 1 positive recipients with DEA 1 negative blood (Acierno et al. 2014). Natural antibodies to DEA 3, 5, and 7 exist in some dogs, but transfusion reactions caused by these incompatibilities are typically mild and delayed (Hale 1995). Sensitization to other DEAs and subsequent hemolytic transfusion reactions have been reported (Callan and Giger 1995; Hale 1995; Melzer et al. 2003). Current evidence shows that pregnancy does not induce DEA alloantibody formation in bitches (Blais et al. 2009).
Some patients lack high-frequency RBC antigens (Blais et al. 2007; Weinstein et al. 2007). Certain Dalmatians, Doberman Pinschers, and Shih Tzus lack an antigen (Dal) commonly found on the surface of most dog RBCs. Transfusion with Dal-positive blood sensitizes Dal-negative transfusion recipients, leading to acute or delayed hemolytic reactions with subsequent transfusions (Blais et al. 2007; Goulet et al. 2014). Similarly, a common RBC antigen (Mik) is absent in some cats. Some Mik-negative cats have naturally occurring alloantibodies against this antigen, which can lead to an acute hemolytic transfusion reaction despite administration of compatible AB blood type. Other Mik-negative cats acquire alloantibodies after transfusion with Mik-positive blood and are at risk of a hemolytic reaction during subsequent transfusion. Pre-existing anti-Mik alloantibodies might be detected on a crossmatch, but this depends on the titer present in the recipient (Weinstein et al. 2007).
Allergic/anaphylactic reactions
Allergic transfusion reactions range in severity from mild to severe anaphylaxis and occur within minutes to hours of transfusion initiation. Type I hypersensitivity responses are the typical cause of allergic transfusion reactions. These reactions can be triggered by patient exposure to a protein or other substance in the donor plasma, especially if the recipient has previously been sensitized to this allergen. The allergen binds to antibody (IgE or IgG) bound by Fc receptors on the surfaces of mast cells and basophils. As mast cells and basophils become activated, granules and chemical mediators (e.g., histamine, platelet activating factor) are released to cause a type I hypersensitivity reaction. Biological response modifiers might also cause non-allergen dependent hypersensitivity reactions. Chemokines and cytokines such as vascular endothelial growth factor and transforming growth factor β1 can directly activate mast cells and basophils, leading to a similar allergic reaction (Fung and Heddle 2013). Plasma and platelet products increase the risk of allergic transfusion reaction in humans. Removing plasma from blood products significantly decreases incidence of allergic transfusion reactions, suggesting that plasma proteins are important mediators of these complications (Tobian et al. 2011).
Clinical signs of allergic transfusion reactions include urticaria, angioedema, edema, and erythema (Figure 11.1). Allergic reactions can be non-systemic or local, comprising focal angioedema and other cutaneous signs. Systemic (generalized) allergic or anaphylactic reactions are more severe and might include skin, respiratory, gastrointestinal, and circulatory clinical signs. Vomiting, diarrhea, tachypnea, tachycardia, hypotension, and other signs of shock can also be noted (Fung and Heddle 2013).

Figure 11.1 Facial angioedema several hours after plasma transfusion in a Golden Retriever. The angioedema was presumably secondary to a type I hypersensitivity/allergic reaction. (Courtesy of Andrea Steele, MSc, RVT, VTS (ECC).)
Transfusion-related acute lung injury
Transfusion-related acute lung injury (TRALI) is one of the most common causes of mortality associated with transfusion in human patients. TRALI is characterized by non-cardiogenic pulmonary edema in the absence of circulatory overload or pre-existing lung injury and occurs within minutes to hours after initiation of transfusion. Transfusion of plasma and platelet products appears to increase risk of TRALI in people. Clinical signs vary in severity and include respiratory distress, fever, tachycardia, and hypotension. Pulmonary infiltrates are observed on radiographs and blood gas analysis documents hypoxemia in patients with TRALI (Toy et al. 2005).
Two main mechanisms are proposed to explain the development of TRALI in people. The antibody-mediated hypothesis proposes that TRALI occurs secondary to transfusion of donor anti-human leukocyte antigen (HLA) or anti-human neutrophil antigen (HNA) antibodies directed against recipient WBCs. This leads to activation of recipient neutrophils in pulmonary capillaries and release of cytotoxic neutrophil enzymes, resulting in capillary damage and leakage. A certain threshold of antibody could be sufficient to cause this complication, or the antibody-mediated mechanism might be part of the “two-hit” mechanism. The non-antibody-mediated hypothesis postulates that TRALI might be the consequence of two consecutive events (“two-hit” mechanism). Patient condition (e.g., sepsis) or previous transfusion can cause increased expression of cellular adhesion molecules and adhesion of activated neutrophils to the pulmonary microvascular endothelium. Subsequently, biologically active substances (e.g., cytokines, lipids, and antibody) are administered with the blood product transfusion, leading to priming and activation of neutrophils, which results in capillary damage and leakage as above. These two mechanisms are not mutually exclusive, as anti-HLA and HNA antibody exposure can be the second event in a previously primed patient (Toy et al. 2005, 2012; Bux 2011).
The majority of TRALI cases in people appear to be antibody-mediated (Toy et al. 2012). Compared to non-parous donors, previously pregnant women are more likely to have HLA and HNA antibodies through exposure to fetal blood, therefore blood products from such donors might increase the risk of TRALI (Toy et al. 2012; De Clippel et al. 2014). Due to the low rate of previously parous veterinary donors, TRALI arising from such a mechanism is unlikely to occur in veterinary patients.
TRALI has not been recognized widely in veterinary patients, but ALI and respiratory distress have been reported in dogs receiving blood transfusions. In a prospective observational study, 3.7% of dogs requiring blood products were diagnosed with ALI after blood transfusion based on the presence of radiographic pulmonary infiltrates, hypoxemia, and clinical signs of respiratory compromise (Thomovsky and Bach 2014). In a retrospective study, 9% of dogs had signs consistent with ALI after PRBC transfusion (Holowaychuk et al. 2014). Acute respiratory distress syndrome is common in critically ill dogs not receiving a blood transfusion, therefore it can be difficult to know whether the ALI was caused by transfusion or occurred secondary to the dog’s primary disease (Figure 11.2).

Figure 11.2 Left lateral (a) and dorsoventral (b) thoracic radiographs from a dog with acute respiratory distress immediately after a plasma and PRBC transfusion. The radiographs demonstrate diffuse interstitial to alveolar pulmonary pattern, more pronounced on the right side. A jugular catheter and gastrostomy tube are present on radiographs. Thoracic radiographs prior to the transfusions were normal. Post-mortem examination identified diffuse pulmonary edema and no evidence of bacterial infection. The main differential diagnosis was non-cardiogenic pulmonary edema, potentially associated with the transfusions.
Immunologic transfusion reactions: delayed
Delayed hemolytic transfusion reactions
Delayed hemolytic transfusion reactions occur >24 hours (usually 3–5 days) after the transfusion and are usually due to a secondary or anamnestic immune response. After a first mismatched transfusion, patients can be sensitized to a specific RBC antigen and mount an antibody titer. This reaction might not result in clinical abnormalities and, over time, the antibody titer declines. Because of this low antibody titer, crossmatching prior to transfusion might not detect the incompatibility. After repeated exposure to the same RBC antigen during a subsequent transfusion, the secondary immune response rapidly induces a higher level of antibody formation, leading to a delayed hemolytic reaction (Massey et al. 2013). Delayed hemolytic transfusion reactions can also occur after first exposure to a novel RBC antigen, triggering a primary immune response. Hemolysis can occur within weeks after transfusion in these recipients, upon sufficient alloantibody production (Massey et al. 2013). Delayed hemolytic transfusion reactions are typically mild in comparison to acute hemolytic reactions and are less likely to be detected clinically. Signs of a delayed hemolytic transfusion reaction include an unexpected decrease in the hematocrit or packed cell volume (PCV) after transfusion, icterus, and rarely hemoglobinemia/hemoglobinuria.
Some dogs that are negative for DEA 3, 5, or 7 have low titers of naturally occurring alloantibodies against the corresponding antigen (Swisher and Young 1961; Hale 1995), therefore transfusion of the corresponding antigen can lead to a delayed transfusion reaction. Although an acute immunologic transfusion reaction is unlikely upon transfusion of DEA 1 positive RBCs to an unsensitized DEA 1 negative recipient, a delayed hemolytic reaction can occur. Anti-DEA 1 antibodies can form within 2 weeks, causing a rapid decline in transfused RBCs as the anti-DEA 1 titer increases in the patient (Young 1949). This delayed reaction is unlikely to cause clinical signs, but it decreases transfusion efficacy and duration of benefit.
Immune complex (type III hypersensitivity) formation
Type III hypersensitivity reactions are caused by antigen–antibody complex formation. Immune complexes can be deposited in tissues where the complement cascade is activated and chemotactic factors are released. Neutrophils migrate to this site and release enzymes and oxidants that cause acute inflammation and tissue damage. Immune complexes can also be formed in circulation when an antigen directly enters the blood. Circulating immune complexes are commonly deposited in glomeruli, resulting in glomerulonephritis. Circulating complexes can also deposit in endothelial cells, causing vasculitis, and the synovium causing arthritis (Tizard 2013). Clinical signs occur within hours to weeks of antigen exposure.
Human serum albumin (HSA) has been used to treat hypoalbuminemia in critically ill dogs and cats. Despite the potential benefits of HSA, severe adverse reactions following transfusion of this product have been reported. In a report of six healthy dogs given 25% HSA, one dog developed an immediate hypersensitivity reaction and all dogs developed delayed reactions consistent with type III hypersensitivity within 2 weeks of transfusion. Clinical signs included peripheral edema, primary vasculitis, and lameness. Two of six dogs developed more severe signs, including disseminated intravascular coagulation (DIC) in one dog and oliguric kidney failure in both dogs. Despite supportive treatment, both of the severely affected dogs died (Francis et al. 2007). Other studies have reported immediate and delayed hypersensitivity reactions in healthy dogs receiving initial and subsequent 25% HSA transfusion (Cohn et al. 2007). Retrospective studies of critically ill and hypoalbuminemic dogs and cats transfused with 5% and 25% HSA do not reveal any severe short-term hypersensitivity reactions, but long-term data is lacking and delayed reactions might not have been reported (Mathews and Barry 2005; Viganó et al. 2010). It is possible that critically ill dogs mount a diminished immune response to HSA compared to healthy dogs, explaining the more severe reactions after HSA transfusion in healthy dogs. However, one report describes type III hypersensitivity reactions leading to vasculitis in two critically ill dogs after HSA transfusion (Powell et al. 2013). Based on these reports, the safety of HSA transfusions is questionable in dogs and cats.
Post-transfusion purpura
A marked thrombocytopenia occurring 5–10 days after transfusion has been reported in human patients. Post-transfusion purpura is thought to result from the secondary immune response, leading to production of alloantibodies against specific platelet antigens after repeated transfusions. This reaction also destroys the patient’s own platelets and can cause clinical signs of bleeding (Murphy 2013). Post-transfusion purpura has been reported in a dog after receiving multiple transfusions (Wardrop et al. 1997a). The overall incidence of this transfusion reaction in veterinary patients is unknown but appears to be rare.
Non-immunologic transfusion reactions: acute
Non-immunologic hemolysis
Non-immunologic hemolysis of RBCs can occur ex vivo (prior to or during the transfusion) secondary to RBC aging, improper storage, bacterial contamination of the product, or improper administration (Prittie 2003; Patterson et al. 2011). Hemolysis of stored RBCs secondary to aging is a marked manifestation of storage lesions, which compromise cell viability.
Improper RBC storage can lead to hemolysis; freezing RBCs will cause cell membrane rupture. To minimize hemolysis of RBCs prior to transfusion, RBC products should be stored in a dedicated refrigerator with a constant temperature set between 1 and 6°C. Temperature changes outside of recommended storage conditions can lead to decreased cell deformability and increased osmotic fragility, resulting in decreased RBC survival (Perrotta and Snyder 2001). Storage at warmer temperatures increases the metabolic rate of RBCs, causing the cells to use nutrients in storage media at a faster rate. Refrigeration slows down storage lesions, compared to RBCs stored at room temperature. Refrigeration also inhibits bacterial growth within RBC units. Bacterial contamination can decrease oxygen within a stored red cell product, decreasing stored RBC lifespan. Release of endotoxins and bacterial enzymes can cause excessive hemolysis and discoloration of the blood product (Hess 2010).
Veterinary guidelines outlining the optimal orientation of RBC units during storage, or whether units should be periodically mixed, are lacking. One veterinary report advises vertical storage with space between bags, on fridge shelves rather than in drawers (Figure 11.3) (Prittie 2003; Patterson et al. 2011). A human study showed that horizontal storage and weekly mixing of PRBC units led to less hemolysis and better maintenance of RBC morphology compared to vertical storage (Högman et al. 1995).

Figure 11.3 Example of a dedicated RBC product storage refrigerator in which blood units are stored in a vertical manner.
Improper transfusion delivery technique can also induce hemolysis of blood products. Ideal methods to deliver blood transfusions allow control of the blood flow rate, while not damaging the transfused RBCs. Damage to transfused RBCs would presumably decrease their lifespan and the efficacy of the transfusion. Transfusions can be administered via gravity flow, through a volumetric peristaltic fluid pump, or through a syringe pump. In-line filtration devices prevent large cellular aggregates from being transfused into the recipient (Figure 11.4).

Figure 11.4 Examples of in-line blood product filters used to remove aggregates from red blood cell products before transfusion. Blood component infusion set with 170–260 micron diameter blood component filter and Luer adapter, attached to a syringe pump (a) and fluid pump (b) for delivery of packed red blood cells.
One canine study showed that volumetric peristaltic fluid pump and syringe pump delivery methods led to more short-term RBC loss compared to gravity flow delivery (McDevitt et al. 2011). A similar feline study comparing RBC transfusion via gravity flow or a syringe pump with attached micro-aggregate filter did not detect any difference in short- or long-term RBC survival between the two methods (Heikes and Ruaux 2014). There is no consensus regarding the optimal transfusion delivery method in veterinary patients. While gravity flow can lead to increased survival of transfused RBCs, this method does not allow for precise control of flow rate or volume delivered. However, new devices are available to assist with drip rate counting, which may alleviate this concern (Hallowell 2015). If fluid pumps are used to administer blood products, only those pumps approved for blood product delivery by the manufacturer should be used. Other causes of hemolysis related to delivery method include the use of small gauge catheters, micro-aggregate filters with small pores, and rapid or forceful transfusion delivery (Prittie 2003). Red cells become hemolyzed when mixed with hypotonic fluids and should only be administered with isotonic calcium-free fluids such as 0.9% saline (Ryden and Oberman 1975).
Storage lesions
Pathophysiology
During refrigerated storage, RBCs undergo progressive oxidative damage and other metabolic and morphologic changes referred to as storage lesions. Storage lesions compromise the integrity of RBCs, leading to fewer intact RBCs being transfused to the recipient and therefore making the transfusion less effective (Flegel et al. 2014; Lacerda et al. 2014; Obrador et al. 2015). Prolonged RBC storage times prior to transfusion might increase risk of morbidity and mortality, although this is controversial (Dzik 2008). The exact mechanism explaining why prolonged storage of blood is linked to poorer patient outcome or complications in some studies is not known but could relate to the changes in RBC products that accumulate over time.
The sole energy source for stored RBCs is glycolysis, eventually leading to adenosine triphosphate (ATP) production. A byproduct of ongoing RBC metabolism in storage is lactic acid and protons, creating an acidic environment in the stored product over time. Acidosis inhibits glycolysis, eventually resulting in decreased levels of ATP. RBCs contain 2,3-diphosphoglycerate (DPG), which is bound to hemoglobin and facilitates tissue oxygen delivery in the body. During storage, RBCs become deficient in 2,3-DPG, which inhibits oxygen transfer from hemoglobin during circulation through oxygen-depleted tissues. Nitric oxide (NO), a vasodilator secreted by endothelial cells, facilitates capillary blood flow and tissue oxygen delivery. A small proportion of hemoglobin molecules are bound to NO, which further promotes the role of NO in facilitating oxygen delivery. Hemoglobin molecules lose their bond to NO over time in stored RBC products and concentrations of 2,3-DPG and NO become depleted from RBCs during storage. However, RBCs regain these molecules several hours after transfusion into the recipient and their role in storage lesions is currently unknown (Hess 2010).
Normal RBCs in circulation are deformable, permitting RBC passage into small capillaries and effective tissue oxygenation. Normal ATP concentrations are important in maintaining a healthy RBC membrane. During storage, RBCs undergo membrane loss secondary to factors such as diminished ATP concentrations and declining pH. Some RBCs in storage can lyse, while others shed microparticles from their cell membrane (Hess 2010). Upon transfusion, stored RBCs are less deformable, causing decreased blood flow in capillaries and increased viscosity. RBC-derived microparticles can act as pro-inflammatory and procoagulant stimuli in the recipient upon transfusion (Herring et al. 2013). Microparticles in stored RBC units might have detrimental effects on transfusion recipients, including TRALI and thrombosis (Jy et al. 2011). Stored RBCs are also more likely to adhere to endothelial cells after transfusion, potentially disrupting blood flow and oxygen delivery to peripheral tissues (Anniss and Sparrow 2006).
Storage lesions can cause a high rate of RBC clearance shortly after transfusion. Approximately 1% of RBCs are normally cleared from circulation daily, leading to hemoglobin release. This source of iron can be coupled with a transport protein such as transferrin, protecting the body from the oxidative damage that free iron can create. In contrast, transfusion of RBC with storage lesions in circulation can lead to a massive infusion of free hemoglobin and iron, overwhelming the body’s capacity to transport iron and causing free, non-transferrin bound iron and potentially oxidative damage (Hod and Spitalnik 2012; Spitalnik 2014). Depending on the additive solution used, RBC products can be stored in the refrigerator for up to 35 days (Wardrop et al. 1997b). In human medicine, standards regulate that stored RBC products have free hemoglobin comprising <0.8–1% of total hemoglobin, and that at least 75% of RBCs must remain in circulation 24 hours after transfusion (Hess et al. 2009).
While many mechanisms are involved in these progressive RBC changes during storage, the presence of residual WBCs in the blood bags might contribute to these storage lesions. Pre-storage leukoreduction might ameliorate the effects of storage lesions and transfusion of older RBC units (Phelan et al. 2010, 2012). Leukoreduction significantly decreased microparticle formation in PRBC units stored ≥14 days, compared to non-leukoreduced PRBC in one canine study (Herring et al. 2013). Leukoreduction of human RBC units caused fewer RBCs to adhere to endothelial cells after storage, compared to non-leukoreduced RBC (Anniss and Sparrow 2006).
Clinical impact of storage lesions in human patients
In human medicine, transfusion of older stored blood products is associated with increased risk of patient mortality and complications such as sepsis and multiple organ failure (Koch et al. 2008; Gauvin et al. 2010; Weinberg et al. 2010; Wang et al. 2011; Manlhiot et al. 2012). However, other human studies have not shown an association between longer storage times and complications after transfusion (Fergusson et al. 2012; Kor et al. 2012; Lebiedz et al. 2012; Lelubre and Vincent 2013). Many of the studies examining the risks of using older stored blood are retrospective, therefore large-scale randomized, controlled prospective trials are needed and ongoing in human medicine (Steiner et al. 2010). One such trial showed that transfusing neonates with fresh (<7 days old) blood did not improve outcomes compared to standard transfusion practices in which mean RBC unit age was 14 days (Fergusson et al. 2012). Another study revealed that transfusing critically ill adults with fresh (<8 days old) blood versus older stored blood did not decrease mortality rates (Lacroix et al. 2015).
Clinical impact of storage lesions in veterinary patients
The effect of blood unit storage times on outcome in dogs has been minimally investigated (Obrador et al. 2015). Dogs with experimentally induced pneumonia and sepsis had decreased survival times and more severe lung damage after massive transfusion with 42-day-old blood compared to 7-day-old blood (Solomon et al. 2013). Healthy dogs transfused with 28-day-old PRBC demonstrated increased inflammatory responses compared to transfusion with 7-day-old PRBC, regardless if pre-storage leukoreduction was performed (Callan et al. 2013). In a large retrospective study of dogs, increased RBC storage times did not adversely affect the overall patient outcome or complication rate. However, longer PRBC storage times were associated with decreased survival in dogs with hemolysis (primarily immune mediated hemolytic anemia), as well as complications such as thromboembolic disease and new or worsening coagulation failure after transfusion (Hann et al. 2014).
Pro-inflammatory mediators such as hemoglobin, microparticles, and other products accumulate in the supernatant during in vitro storage of PRBC, and might exacerbate the inflammatory response of dogs with IMHA (Callan et al. 2013; Corsi et al. 2014; Hann et al. 2014). Additionally, transfusion of older stored blood might induce or propagate coagulation abnormalities due to the direct effects of inflammation on hemostasis (Cheng et al. 2011). The impact of storage lesion on blood transfusion and patient outcome warrants further study in veterinary medicine.
Transfusion-associated circulatory overload
Some blood products exert a significant colloid effect, therefore high doses of blood products can increase the risk of circulatory overload (Tocci 2010; Holowaychuk et al. 2014). Circulatory overload can result from rapid transfusion rates or high transfusion volumes. Patients with cardiac dysfunction, pulmonary disease, kidney failure, and chronic anemia might be at higher risk of circulatory overload and therefore should be administered transfusions at slower rates (Tocci 2010). Clinical signs of circulatory overload are related to the development of pulmonary edema or pleural effusion and can include dyspnea, tachypnea, cyanosis, hypoxemia, and respiratory distress. Pulmonary venous distention and pulmonary edema or effusion might be evident on thoracic radiographs (Figure 11.5).

Figure 11.5 Ventrodorsal thoracic radiograph of a cat that developed respiratory distress during a whole blood transfusion. The cat had an increase in size of the cardiac silhouette and right caudal lobar artery and caudal vena cava, as well as increased alveolar infiltrate compared to pre-transfusion radiographs. The primary differential diagnosis for the radiographic findings and clinical signs was transfusion-associated circulatory overload and cardiogenic pulmonary edema.
Citrate toxicity
Most blood products are stored in a citrate anticoagulant, which is normally metabolized to bicarbonate after transfusion. However, large transfusion volumes can overwhelm this metabolic pathway, leading to citrate toxicity and hypocalcemia (Blumenthal et al. 1999; Jutkowitz et al. 2002). Clinical signs of hypocalcemia can be observed including tremors, seizures, and arrhythmias. Because citrate is metabolized in the liver, patients with liver dysfunction are at increased risk of citrate toxicity (Callan 2013; Webert and Heddle 2013).
Hypothermia
Rapid or massive transfusions of refrigerated blood can lead to hypothermia. Stored RBC products are not routinely warmed to room temperature or 37°C unless being given rapidly in large volumes. Because transfusions are often given over a few hours, the product approaches ambient temperature during this time (Chiang et al. 2011; Lee et al. 2014). Only commercial warmers should be used to warm blood products; excessive heating can lead to hemolysis (Poder et al. 2015).
Bacterial contamination of blood products
Bacterial contamination of blood products can result from donor bacteremia, skin contaminants, or contaminated collection devices. Visual inspection of a blood product can help detect bacterial contamination. RBC products contaminated with bacteria can have dark or black discoloration and the presence of bubbles (Hohenhaus et al. 1997; Ramirez-Arcos and Goldman 2013). Bags suspected of contamination must not be used for transfusion and should be submitted for bacterial culture. Many blood banks routinely culture bags after transfusion as a way to monitor aseptic collection technique. Blood bags should be cultured after any suspected transfusion reaction that could have been caused by bacterial contamination.
Transfusion of bacteria contaminated blood products can induce sepsis and an acute hemolytic transfusion reaction. Clinical signs include vomiting, diarrhea, fever, hypotension, icterus, hemoglobinemia, and hemoglobinuria. Signs of septic shock and disseminated intravascular coagulation can also occur (Hohenhaus et al. 1997; Roth et al. 2000).
Risks of massive transfusions
Massive transfusions are defined as transfusion volumes that exceed the patient’s estimated blood volume (dogs 80–90 mL/kg; cats 40–60 mL/kg) delivered within 24 hours, or half of the patient’s blood volume delivered within 3 hours (Groom and Rowlands 1958; Finsterer et al. 1973; Jutkowitz et al. 2002; Hopper et al. 2012). Veterinary patients receiving massive transfusions have high mortality rates (>70%), likely attributable to the severity of the underlying illness (Jutkowitz et al. 2002). Complications of massive transfusions include hypocalcemia, hypomagnesemia, other electrolyte disturbances, alteration of acid-base status, thrombocytopenia, dilutional coagulopathy, and hypothermia (Jutkowitz et al. 2002; Roux et al. 2008; Sihler 2010).
Non-immunologic transfusion reactions: delayed
Bloodborne infectious disease transmission
Infectious disease screening is strongly recommended prior to using dogs and cats as blood donors and routine parasite prophylaxis is suggested in donors to decrease the risk of bloodborne infections (Wardrop et al. 2005). Ideally, annual infectious disease screening should be performed and cats that go outdoors should be omitted from the donor pool. Specific infectious diseases that are screened for vary according to the geographic region and donor lifestyle, but include both vector-borne and non-vector-borne agents (Wardrop et al. 2005; Tasker and Warman 2013). Transmission of Babesia gibsonii and Leishmania spp. via blood transfusion resulting in clinical disease has been reported in dogs (Owens et al. 2001; Stegeman et al. 2003).
Transfusion-related immunomodulation
Transfusion of blood products can induce pro-inflammatory reactions and quell the recipient’s immune response (Izbicki et al. 2004; McMichael et al. 2010; Jackman 2013). As a result, blood transfusions can negatively impact patient outcome. Transfusion-related immunomodulation (TRIM) is a broadly used term encompassing both the transient immunosuppression, as well as the pro-inflamamtory response observed in human patients after blood transfusion (Vamvakas and Blajchman 2007). The exact mechanism causing TRIM is unknown, but is likely to be multifactorial and involve mediators such as WBCs and soluble plasma proteins within RBC units. During storage of RBC units, a more acidic environment develops, leading to WBC degradation over approximately 2 weeks. The WBCs become activated, leading to release of cytokines and eventual WBC death (Vamvakas and Blajchman 2007; Vamvakas 2013). As a result, the supernatant of RBC units becomes rich in pro-inflammatory and procoagulant mediators over time (Sparrow and Patton 2004). Transfusion of donor WBCs might also cause direct suppression of the recipient’s immune system (Bordin et al. 1994). Ultimately, the suspected mediators of TRIM appear to cause a shift in T helper (Th) cell type profile from Th1 to Th2, resulting in suppression of cell-mediated immunity. The functions of natural killer cells and macrophages, as well as other immune system roles, are also suppressed after blood transfusion (Vamvakas 2013).
TRIM has been associated with both beneficial and detrimental patient outcomes. Human patients receiving blood transfusion prior to renal transplants had improved graft survival, a potential benefit of TRIM (Opelz et al. 1973, 1997; Marti et al. 2006; Vamvakas 2013). Suspected detrimental effects of TRIM in human patients include recurrence or metastasis of cancer, increased rate of perioperative and other infections, and increased short-term mortality. However, there is conflicting evidence describing the role of autologous blood transfusion in such adverse sequelae (Vamvakas 1996, 2007; McAlister et al. 1998; Motoyama et al. 2004; Chalfin et al. 2014; Kluth et al. 2014; Rohde et al. 2014; Yeoh et al. 2014;).
Pre-storage leukoreduction appears to mitigate activity of the WBC-derived pro-inflammatory and immunomodulating mediators implicated in TRIM, but does not reduce other soluble plasma factors such as HLA molecules (Sparrow and Patton 2004; Vamvakas 2007, 2013). While leukoreduction was associated with decreased patient mortality in people after cardiac surgery, this strategy has not consistently improved the suspected consequences of TRIM such as increased mortality or post-operative infections in other studies (Hébert et al. 2003; Vamvakas 2007; Englehart et al. 2009).
Diagnosis of transfusion reactions
Diagnosis of transfusion reactions relies on veterinary professionals identifying patient factors that could increase individual risk of transfusion reactions, rapidly recognizing the signs of a reaction, and providing vigilant patient monitoring. Detecting transfusion reactions can be challenging, in part due to the variety of clinical signs that can signal a reaction (see Table 11.1). Signs of transfusion reaction can be non-specific (e.g., fever could be due to acute hemolytic or non-hemolytic reactions or bacterial contamination). Additionally, the patient’s underlying disease can make it challenging to determine if new clinical signs are related to disease progression or a transfusion reaction (Figure 11.6).

Figure 11.6 A Great Dane develops suspected transfusion-associated complications, including peripheral edema and acute lung injury, after receiving a blood transfusion for the treatment of hemoabdomen secondary to a ruptured splenic mass and liver nodules. (Courtesy of Marie K. Holowaychuk, DVM, DACVECC.)
Baseline complete blood count, serum biochemical profile, coagulation profile, and urinalysis are recommended in patients prior to transfusion. While these results are important in the diagnostic approach to the patient’s underlying disorder and assessing clinical status, the parameters might also serve as future comparison points in cases of suspected transfusion reactions.
There is no consensus as to how frequently to monitor patients during a blood transfusion. In general, baseline measurements of PCV (or hematocrit), total solids (TS), plasma color (hemolysis, icterus), mentation, body temperature, heart rate, respiratory rate and effort, and blood pressure should be recorded just prior to initiating the transfusion. The presence of hemoglobinemia/hemoglobinuria, vomiting, pruritus, erythema, and urticaria prior to transfusion should also be noted. As a minimum, vital parameters should be compared to baseline values once within the first 15 minutes after initiating the transfusion, then periodically throughout the transfusion. Vital parameters should be taken again within 1–2 hours of completing the transfusion, and rechecked at least 12 and 24 hours after transfusion or more often as dictated by patient status. Reassessment of PCV/TS and changes in plasma color (for presence of icterus or hemolysis) should be reassessed at least once 12–24 hours after transfusion (Figure 11.7).


Figure 11.7 Example of a blood transfusion request form used at the Ontario Veterinary College, including a table to record the recommended patient monitoring parameters. (Courtesy of Marie K. Holowaychuk, DVM, DACVECC and the University of Guelph.)
Treatment of transfusion reactions
Transfusion reactions require prompt attention and have the potential to be fatal. At the first sign of a transfusion reaction, the transfusion should be slowed or discontinued. Changes in vital parameters and other clinical signs will help to dictate what therapy is required (Table 11.2). Monitoring of urine output, kidney values, clotting times, and other parameters related to the patient’s clinical status is recommended throughout the treatment of a transfusion reaction.
Table 11.2 Recommended drugs for acute transfusion reactions including indications, doses, route, and frequency of administration
Indication | Drug | Dose | Route | Frequency | Note |
Severe febrile non-hemolytic reaction | Dipyrone | 10–20 mg/kg | IV given slowly | Once | Treatment usually not indicated |
Cardiac arrest associated with transfusion reaction | Epinephrine | 0.01–0.1 mg/kg | IV | Q 3–5 minutes | As per current CPR guidelines (Fletcher et al. 2012) |
Acute hemolytic reaction | Dexamethasone | 0.25 mg/kg | IV, IM, or PO | Q 24 h | Unproven efficacy |
Prednisone, prednisolone | 2 mg/kg | PO | Q 24 h | Unproven efficacy | |
Allergic/anaphylactic reactions | Diphenhydramine | 2 mg/kg | IM or PO | Q 24 h | |
Dexamethasone | 0.25 mg/kg | IV, IM, or PO | Q 24 h | ||
Prednisone, prednisolone | 2 mg/kg | PO | Q 24 h | ||
Famotidine | 0.5–1 mg/kg | IV or SC | Q 12 h | ||
Emesis associated with transfusion | Ondansetron | 0.1–1 mg/kg | IV given slowly; PO | Q 6–12 h | |
Maropitant | 1 mg/kg | SC | Q 24 h | ||
Metoclopramide | 0.1–0.4 mg/kg | SC or IM | Q 6 h | ||
0.3 mg/kg bolus followed by 1–2 mg/kg/day CRI | IV | CRI | |||
Circulatory overload | Furosemide | 2–4 mg/kg (up to 8 mg/kg in dogs) | IV or IM | Q 1–2 h or as clinically indicated; CRI of 0.66 mg/kg/hour can be used | |
Hypocalcemia/citrate toxicity | Calcium gluconate 10% | 0.5–1.5 mL/kg | IV given over 10–20 minutes | Once, to effect | Document hypocalcemia (ionized Ca < 1.0 mmol/L) prior to treatment; monitor ECG during administration |
Hypomagnesemia/citrate toxicity | Magnesium sulfate | 0.75–1 mEq/kg/day CRI | IV | CRI |
CPR, cardiopulmonary resuscitation; CRI, continuous rate infusion; h, hours; IM, intramuscular; IV, intravenous; PO, per os (oral); PRN, as needed; Q, every; SC, subcutaneous.
Febrile non-hemolytic reactions
Mild febrile non-hemolytic transfusion reactions are often self-limiting, requiring no specific therapy. The transfusion rate can be decreased and the patient monitored closely for the development of other clinical signs (Tocci 2010). More severe reactions might require discontinuation of the transfusion. Intravenous fluid support can be sufficient therapy to treat a febrile reaction. Active cooling might be necessary in some cases of fever, but antipyretic therapy (e.g., dipyrone) is uncommonly used. Vigilant patient monitoring is required in case fever is the first sign of a more serious reaction (such as acute hemolysis or sepsis).
Acute hemolytic reactions
Hemolytic transfusion reactions require immediate, intensive intervention. If these reactions are suspected, the transfusion should be discontinued immediately and vital parameters measured. Emergency interventions might include volume resuscitation with intravenous crystalloid and/or colloid fluid support, supplemental oxygen, intubation and manual ventilation, and/or epinephrine. Further cardiovascular support with vasopressors and other interventions might also be needed (Fletcher et al. 2012). Glucocorticoids can suppress the mediators of acute hemolytic reactions, but the efficacy of this treatment is not established (Brooks 2003). Fluid therapy should be tailored to maintain adequate blood pressure (mean >70–80 mmHg) and urine output (1–2 mL/kg/hour), ensuring adequate hydration and perfusion (Tocci 2010). During acute transfusion reactions, serial assessment and documentation of PCV/TS, plasma color, and pigmenturia enables monitoring the degree of hemolysis (Figure 11.8).

Figure 11.8 Hemolytic plasma in a hematocrit tube from a dog with primary immune-mediated hemolytic anemia, immediately following transfusion. The degree of hemolysis was mildly worsened compared to that before transfusion. A mild hemolytic transfusion reaction could not be ruled out, but progression of disease was also considered as a possible cause of this change.
Because septic shock has similar clinical signs as acute hemolytic reactions, broad-spectrum antibiotic therapy is recommended while patient and blood product bacterial cultures are pending. Patients experiencing a severe acute transfusion reaction should also be monitored for the development of acute kidney injury, disseminated intravascular coagulation, and other complications.
Allergic and anaphylactic reactions
Mild to moderate allergic and generalized allergic/anaphylactic reactions are treated with diphenhydramine and corticosteroids (Tocci 2010; Hirayama 2013). Treatment of allergic reactions also includes H2 blockers (e.g., famotidine); antiemetic therapy (e.g., maropitant, metoclopramide, ondansetron) might be indicated depending on the clinical signs. Patients experiencing generalized allergic/anaphylactic reactions might require similar emergency interventions as outlined for patients with acute hemolytic reactions (volume resuscitation, oxygen therapy, ventilation, and other cardiovascular support), as well as intensive monitoring.
Acute mild to moderate local allergic reactions usually resolve shortly after discontinuing the transfusion. If the reaction is mild, the transfusion can often be resumed at a slower rate once the clinical signs have resolved (Tocci 2010).
Circulatory overload
In cases of circulatory overload resulting in tachypnea, hypoxemia, or respiratory distress, diuretic therapy (furosemide) and supplemental oxygen therapy are necessary. Once clinical signs have resolved, the transfusion can be initiated again at a slower rate.
Citrate toxicity
Citrate toxicity can cause clinical signs of hypocalcemia including tremors, muscle twitching, tetany, facial rubbing, restlessness, and panting. If clinical signs are suggestive of citrate toxicity, ionized calcium concentrations should be measured. If ionized hypocalcemia (<1.0 mmol/L) is documented, 10% calcium gluconate can be administered slowly to effect while monitoring the patient’s ECG (Schneck et al. 2012). Hypomagnesemia is also common with citrate toxicity and can contribute to the previously mentioned clinical signs as well as cardiac arrhythmias (Meilke and Milne 2000; Jutkowitz et al. 2002). Hypomagnesemia can be treated with a magnesium sulfate infusion.
Delayed transfusion reactions
Delayed transfusion reactions are mild and usually only noted incidentally during recheck blood work. These reactions do not require treatment, nor is corticosteroid therapy efficacious in treating such reactions (Massey et al. 2013).
Premedication prior to transfusions
The efficacy of premedication with anti-inflammatory drugs or diphenhydramine is not proven in human patients to prevent transfusion reactions (Wang et al. 2002; Sanders et al. 2005; Martí-Carvajal et al. 2010). Pretreatment with acetaminophen and diphenhydramine might decrease incidence of febrile non-hemolytic transfusion reactions in people with a previous history of transfusion reaction (Kennedy et al. 2008). However, acetaminophen is contraindicated in cats and generally not used in dogs, given its narrow safety margin (Sellon 2013). As a result, extrapolating the potential benefits of premedication prior to transfusion is difficult for veterinary patients and currently this practice is not routinely recommended.
A survey of referral and veterinary teaching hospitals revealed that 8% of survey respondents routinely administered diphenhydramine prior to all blood transfusions (Jagodich and Holowaychuk 2016). A retrospective study investigating 558 dogs receiving 935 transfusions found that premedication with corticosteroids and/or antihistamines did not influence the overall risk of transfusion reactions. However, administration of antihistamines prior to transfusion decreased the likelihood of allergic transfusion reactions (Bruce et al. 2015). While premedication prior to blood transfusion is not routinely recommended for veterinary patients, the potential benefits of this practice might warrant future investigation.
Approach to transfusion reactions
After identifying a suspected transfusion reaction, the transfusion should be slowed or discontinued, and emergency treatment of the patient initiated as previously outlined. In addition to the emergency care of the patient, several immediate steps should be taken to investigate the reason for the transfusion reaction (Box 11.1). The appropriate species and blood type of the blood product and the recipient should be verified. In addition, the blood product’s expiry date should be confirmed. The blood product should also be assessed for hemolysis by centrifuging an aliquot of blood from the bag in a hematocrit tube and examining the plasma for hemolysis. In cases when a crossmatch was not performed prior to transfusion, this test should be performed if pre-transfusion blood from the recipient is available. The crossmatch can indicate if there is a pre-existing immunologic reaction, suggesting the presence of alloantibody prior to transfusion. The blood product should also be Gram stained and submitted for bacterial culture to investigate possible contamination. Blood transfusion reactions should be well documented in the patient’s and blood donor’s file.

Full access? Get Clinical Tree
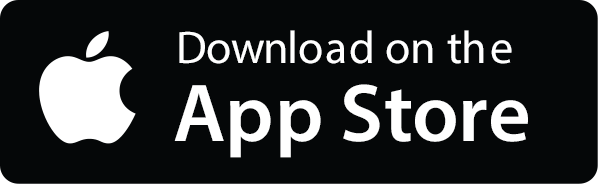
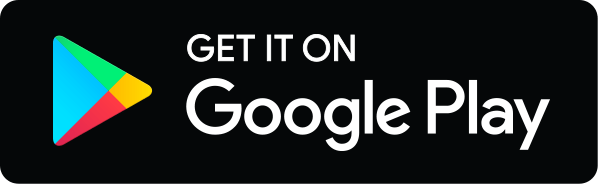