4 Michel R. Popoff In animals, Clostridium chauvoei, Clostridium novyi, Clostridium septicum, and Clostridium sordellii are responsible for severe toxico-infections, which result mainly from wound contamination, except for C. chauvoei which can induce the so-called endogenous myositis (blackleg). These clostridia produce potent toxins that are the main virulence factors involved in the generation of lesions and clinical signs. C. chauvoei has more invasive properties than the other clostridia in this group. Histotoxic clostridia produce an array of different toxins with various modes of action that contribute synergistically to the local and systemic lesions and symptoms. These toxins recognize a wide range of cell types including epithelial, muscle and red blood cells, and lymphocytes. They attack the cells in different ways: disorganization of the actin cytoskeleton and intercellular junctions, and membrane damage by pore formation through the membrane and/or degradation of membrane lipid bilayers. In addition, hydrolytic enzymes secreted by these bacteria such as DNase, protease, collagenase, and hyaluronidase amplify the tissue degradation initiated by the main toxins. A remarkable feature of the lesions produced by these histotoxic clostridia is the paucity or absence of the inflammatory response because of the cytotoxic effect of the toxins on the inflammatory cells and their inhibition of migration. The toxins produced by the histotoxic clostridia can be divided into two families: Table 4.1 Main toxins and other virulence factors of histotoxic clostridia The major toxins produced by C. novyi and C. sordellii belong to the large clostridial glucosylating toxin (LCGT) family. The LCGT family encompasses C. novyi alpha-toxin (TcnA), C. sordellii lethal toxin (TcsL), and hemorrhagic toxin (TcsH), as well as Clostridium difficile toxins A and B (TcdA and TcdB) and TpeL (toxin C. perfringens large cytotoxin) from C. perfringens. TcdB and TcsL are highly related (76% amino acid sequence identity) and are more distantly related to TcdA and TcnA (48–60% identity). These toxins target Rho- and/or Ras-GTPases. Most C. sordellii isolates are non-toxic after isolation from human or animal patients with infection by this microorganism. Toxin genes are likely on plasmid or other mobile elements which are easily lost after sub-culturing. Most toxigenic C. sordellii strains contain only a functional tcsL gene and no or a truncated tcsH gene. Only a few strains produce both TcsL and TcsH. tcsH is located downstream from the tcsL gene, as in C. difficile where tcdA is downstream of tcdB, but tcsH is in the opposite orientation compared to tcsL. In contrast to C. difficile, which shows numerous genetic variations in toxin genes, only two variants of TcsL have been reported (TcsL-82 from IP82 and TcsL-9048 from VPI9048). C. novyi strains are divided into types A, B, C, and D (the latter reclassified as Clostridium haemolyticum) which are closely related to C. botulinum group III (Chapter 1, Figure 1.1). The name of C. novyi sensu lato has been proposed to designate this group of strains. These strains contain numerous plasmids or circular prophages, but horizontal gene transfer between these strains mainly results from phage transduction. Like botulinum neurotoxin genes type C, D, or mosaic C/D, D/C, which are harbored by phages in C. botulinum group III, tcnA is located on C. novyi prophages and these phages can be interchanged between C. botulinum and C. novyi. However, TcnA is highly conserved (99% identity at the amino acid level) in the different C. novyi strains. In contrast, C. novyi beta-toxin is located on the chromosome and is produced mainly by C. haemolyticum and also by C. novyi type B (Table 4.2). Gamma toxin is reported to be a phospholipase but awaits further characterization. Table 4.2 Toxins of Clostridium novyi and Clostridium haemolyticum 1 Gamma toxin is reported to be a phospholipase and needs further characterization. +: toxin produced; ++ large amounts of toxin produced; -: no toxin produced. LCGTs are single protein chains containing at least four functional domains (receptor binding, translocation, cysteine protease, and catalytic domains). The one-third C-terminal part exhibits multiple repeated sequences (31 short repeats and 7 long repeats in TcdA), which are involved in the recognition of a cell-surface receptor. A tri-saccharide (Gal-α1-3Gal-β1-4GlcNac) has been found to be the motif recognized by TcdA, but this motif is absent in humans. Related carbohydrates could be involved as TcdA receptor. A member of the heat shock protein family, gp96, has been proposed to bind TcdA to the plasma membrane of enterocytes. The receptor for the other large clostridial toxins has not been characterized. TcdA repeats consist of a β-hairpin followed by a loop and the carbohydrate-binding domain adopts a β-solenoid fold. Additionally, the intermediate LCGT part harbors an alternative receptor-binding domain. The central part contains a hydrophobic segment and probably mediates the translocation of the toxin across the membrane. The enzymatic site characterized by the DxD motif surrounded by a hydrophobic region, and the substrate recognition domain are localized within the 543 N-terminal residues corresponding to the natural cleavage site in TcdB. The N-terminal catalytic domain is cleaved off through an auto-proteolytic process stimulated by inositol hexakisphosphate and released into the cytosol. Indeed, a cysteine protease site (D587, H653, C698 in TcdB) has been identified close to the cutting site. The overall structure of the enzymatic domains of TcsL, TcdB, TcdA, and TcnA is conserved and consists of a β-strain central core (about 235 amino acids) forming an active center pocket surrounded by numerous α-helices (Figure 4.1). The structure of the central core is similar to that of the glucosyltransferase A family. The first aspartic residue of the DxD motif binds to ribosyl and glucosyl moieties of UDP-glucose and the second aspartic residue binds to a divalent cation (mainly Mn2+) which increases the hydrolase activity and/or the binding of UDP-glucose. Other amino acids in TcdB with an essential role in enzymatic activity have been identified, such as Trp102, which is involved in the binding of UDP-glucose, Asp270, Arg273, Tyr284, Asn384, Trp520, as well as Ile383 and Glu385, important for the specific recognition of UDP-glucose. Differences in α-helices, insertions − deletions, probably account for the substrate specificity of each toxin. Chimeric molecules between TcdB and TcsL have been used to identify the sites of Rho-GTPase recognition. Amino acids 408 to 468 of TcdB ensure the specificity for Rho, Rac, and Cdc42, whereas in TcsL, the recognition of Rac and Cdc42 is mediated by residues 364 to 408, and that of Ras proteins by residues 408 to 516. The four N-terminal helices which mediate the binding of TcsL to phosphatidylserine, are possibly involved in membrane interaction. Amino acids 22–27 of Rho and Ras GTPases, which are part of the transition of the α1-helix to the switch I region, are the main domain recognized by the glucosylating toxins. Figure 4.1 Domain organization and structure of the catalytic domain of large clostridial glucosylating toxins (LCGTs). TcsL is shown as a representative member of this toxin family. LCGTs contain C-terminal repeats involved in the recognition of the cell surface receptor and a central hydrophobic domain mediating the translocation of the N-terminal catalytic domain into the cytosol through the endosome membrane. The catalytic domain is cleaved from the rest of the molecule by an autocleavage process involving the cysteine protease domain which contains the active site DHC. The catalytic domain structure shows a compact core of β-sheet surrounded by numerous α-helices with central catalytic motif (DxD) and an extension of four N-terminal helices. Large clostridial glucosylating toxins enter cells by receptor-mediated endocytosis. The cytotoxic effects are blocked by endosomal and lysosomal acidification inhibitors (monensin, bafilomycin A1, ammonium chloride) and the inhibiting effects can be bypassed by an extracellular acidic pulse. This indicates that the large glucosylating clostridial toxins translocate from early endosomes upon an acidification step. At low pH, TcdA, TcdB, and TcsL induce channel formation in cell membranes and artificial lipid bilayers, and show an increase in hydrophobicity, as determined with fluorescence methods. Membrane cholesterol seems critical for TcdA pore formation. This process probably involves a conformational change and insertion of the toxin into the membrane, possibly mediated by the hydrophobic segment of the central domain, but the exact mode of translocation remains to be determined. The N-terminal domain is then delivered into the cytosol by an auto-proteolytic activity stimulated by inositol hexakisphosphate. A cysteine protease domain has been identified close to the cutting site in TcdB (amino acids 544–955), which is conserved in all large clostridial toxins. Large glucosylating clostridial toxins catalyze the glucosylation of Rho- and/or Ras-GTPases from UDP-glucose, except TcnA, which uses UDP-N-acetylglucosamine as co-substrate. TcdA and TcdB glucosylate Rho, Rac, and Cdc42 at Thr-37, whereas LT glucosylates Ras at Thr-35, and Rap, Ral, and Rac at Thr-37 (Table 4.3). The large glucosylating clostridial toxins cleave the co-substrate and transfer the glucose moiety to the acceptor amino acid of the Rho proteins. The conserved Thr, which is glucosylated, is located in switch I. Thr-37/35 is involved in the coordination of Mg2+ and subsequently in the binding of the β and γ phosphates of GTP. The hydroxyl group of Thr-37/35 is exposed to the surface of the molecule in its GDP-bound form, which is the only accessible substrate of glucosylating toxins. The nucleotide binding of glucosylated Ras by LT is not grossly altered, but the GEF activation of GDP forms is decreased. Glucosylation of Thr-35 completely prevents the recognition of the downstream effector, blocking the G-protein in the inactive form. The crystal structure of Ras modified by LT shows that glucosylation prevents the formation of the GTP conformation of the effector loop of Ras, which is required for the interaction with the effector Raf. Similar results were found with RhoA glucosylated by ToxB. In addition, glucosylation of GTPase slightly reduces the intrinsic GTPase activity, completely inhibits GAP-stimulated GTP hydrolysis, and leads to accumulation of the GTP-bound form of Rho at the membrane where it is tightly bound. Table 4.3 Substrate specificity of Clostridium sordellii and Clostridium novyi large toxins (Genth et al., 2014) Large clostridial glucosylating toxins induce cell rounding by inactivating Rho proteins, resulting in loss of actin stress fibers, reorganization of the cortical actin, disruption of the intercellular junctions, and thus an increase in cell barrier permeability. Rac inactivation is a major player in actin cytoskeleton disorganization. Indeed, TcsL and the other LCGTs induce paxilline dephosphorylation in a Rac-dependent manner, leading to disassembly of the focal adhesions, adherens junctions, and actin filament disorganization. TcdA and TcdB disrupt apical and basal actin filaments and subsequently disorganize the ultrastructure and component distribution (ZO-1, ZO-2, occludin, claudin) of tight junctions, whereas E-cadherin junctions show little alteration. In contrast, TcsL, which only modifies Rac among the Rho proteins, alters the permeability of intestinal cell monolayers, causing a redistribution of E-cadherin, whereas tight junctions are not significantly affected. In vivo, LT causes a marked edema in the cardio-respiratory system by altering E-cadherin junctions of lung endothelial cells. TcdB and TcdA induce apoptosis as a consequence of Rho glucosylation and caspase activation or possibly cell necrosis. TcdB and TcsL may cause apoptosis by targeting mitochondria. In addition to the effects on the cytoskeleton, the inactivation of Rho proteins impairs many other cellular functions such as endocytosis, exocytosis, lymphocyte activation, immunoglobulin-mediated phagocytosis in macrophages, NADPH oxidase regulation, smooth muscle contraction, phospholipase D activation, activation of the pro-apoptotic RhoB, and transcriptional activation mediated by JNK, and/or p38. RhoB has been found to exhibit pro-apoptotic activity in apoptosis induced by those LCGTs that preferably glucosylate Rho-GTPases (including TcsH, TcdA, and TcdB). In contrast, RhoB exhibits cytoprotective activity in apoptosis induced by TcsL, which preferably glucosylates Ras-GTPases. The effect of RhoB seems to depend on the background of the activity of other GTPases. In a background of inactive RhoA and almost active Ras (such as in cells treated with TcsH, TcdA, or TcdB), RhoB exhibits pro-apoptotic activity, whereas on a background of active RhoA but inactive Ras, RhoB exerts cytoprotective activity (such as in cells treated with TcsL). TcdA and TcdB produce a severe inflammatory response in the mammalian intestine characterized by epithelial cell necrosis and massive infiltration with inflammatory cells. In monocytes, TcdA stimulates cytokine (TNF-α, IL-1β, IL-6, and IL-8) release and activation of p38 MAP kinase, whereas the activation of ERK and JNK is only transient. p38 activation is required for IL-8 production, IL-1β release, monocyte necrosis, and intestinal mucosa inflammation. TcdA-induced p38 activation could be mediated by toxin binding to a membrane receptor independently of Rho-GTPase glucosylation. TcdB also induces an inflammatory response including the production of Il-8 via EGF receptor and ERK activation. Other Rho-independent cellular effects induced by TcdA include the activation of NF-kB and subsequent release of IL-8 and possibly other inflammatory cytokines, mitochondrial damage, apoptosis, and activation of a neuro-immune pathway. TcsL also activates JNK independently of small GTPase glucosylation, but JNK activation facilitates target glucosylation by TcsL.
Toxins of Histotoxic Clostridia: Clostridium chauvoei, Clostridium septicum, Clostridium novyi, and Clostridium sordellii
Introduction
Clostridium
Toxin
Toxin family
Molecular size (kDa)
Biological activity
Substrate
Cellular effects
C. sordellii
Lethal toxin (LT or TcsL)
LCGT
270.26
Glucosylation
Rho/Ras
(UDP glucose)
Actin cytoskeleton and intercellular junction alterations
Cell necrosis
Hemorrhagic toxin (HT or TcsH)
LCGT
298.87
Glucosylation
Rho/Ras
(UDP glucose)
Actin cytoskeleton and intercellular junction alterations
Cell necrosis
Sordellilysin
CDC
55.22
Pore formation
Cell necrosis
Phospholipase
Phospholipase
45.58
Membrane damaging
Phosphatidyl-choline
Cell necrosis
Neuraminidase
44.61
Leucocyte proliferation, leucocytose
Collagenase
C. novyi
Alpha toxin (TcnA)
LCGT
249.83
Glucosylation
Rho
Actin cytoskeleton and intercellular junction alterations
Cell necrosis
Beta toxin
Phospholipase C
45.87
Inflammation, edema, increased vascular permeability, muscle contraction
Novyilysin
CDC
Pore formation
Cholesterol
Cell necrosis
Hemolysis
Phospholipase C
Phospholipase
Membrane damaging
Phosphatidyl-choline
sphingomyelin
Hemolysis
C. septicum
Alpha toxin
β-PFT
49.71
Pore formation
Cell necrosis
Septicolysin
CDC
Pore formation
Cell necrosis
Hemolysis
C. chauvoei
CctA
β-PFT
35.51
Chauveolysin
CDC
Pore formation
Cholesterol
Cell necrosis
Hemolysis
Neuraminidase
Other virulence factors: DNase, hyaluronidase
A – Intracellularly active toxins from histotoxic clostridia
C. novyi type
Alpha toxin (TcnA)
Beta toxin
Gamma toxin1
Disease
A
+
−
+
Gangrene humans, animals
B
+
+
−
Gangrene animals;
Necrotizing hepatitis animals
C
−
−
+?
No disease reported
D (C. haemolyticum)
−
++
=
Bacillary hemoglobinuria animals
Structure
Mode of action
Toxin
Substrate
RhoA
RhoB
RhoC
RhoG
Rac1
Cdc42
TC10
TCL
H-Ras
N-Ras
K-Ras
Rap2a
RalC
R-Ras1
R-Ras2
R-Ras3
TcsL-82
+
+
−
+
+++
−
+
+
+++
+++
+++
+++
++
++
++
++
TcsL-9048
+
+
+
+
+++
+
++
++
++
++
++
++
++
−
−
+
TcnA
+++
+++
+
+
+++
++
++
++
−
−
−
−
−
−
−
−
TcsH-9048
++
++
++
+
+++
++
+
+
+
+
+
++
+
−
+
−
Cellular effects
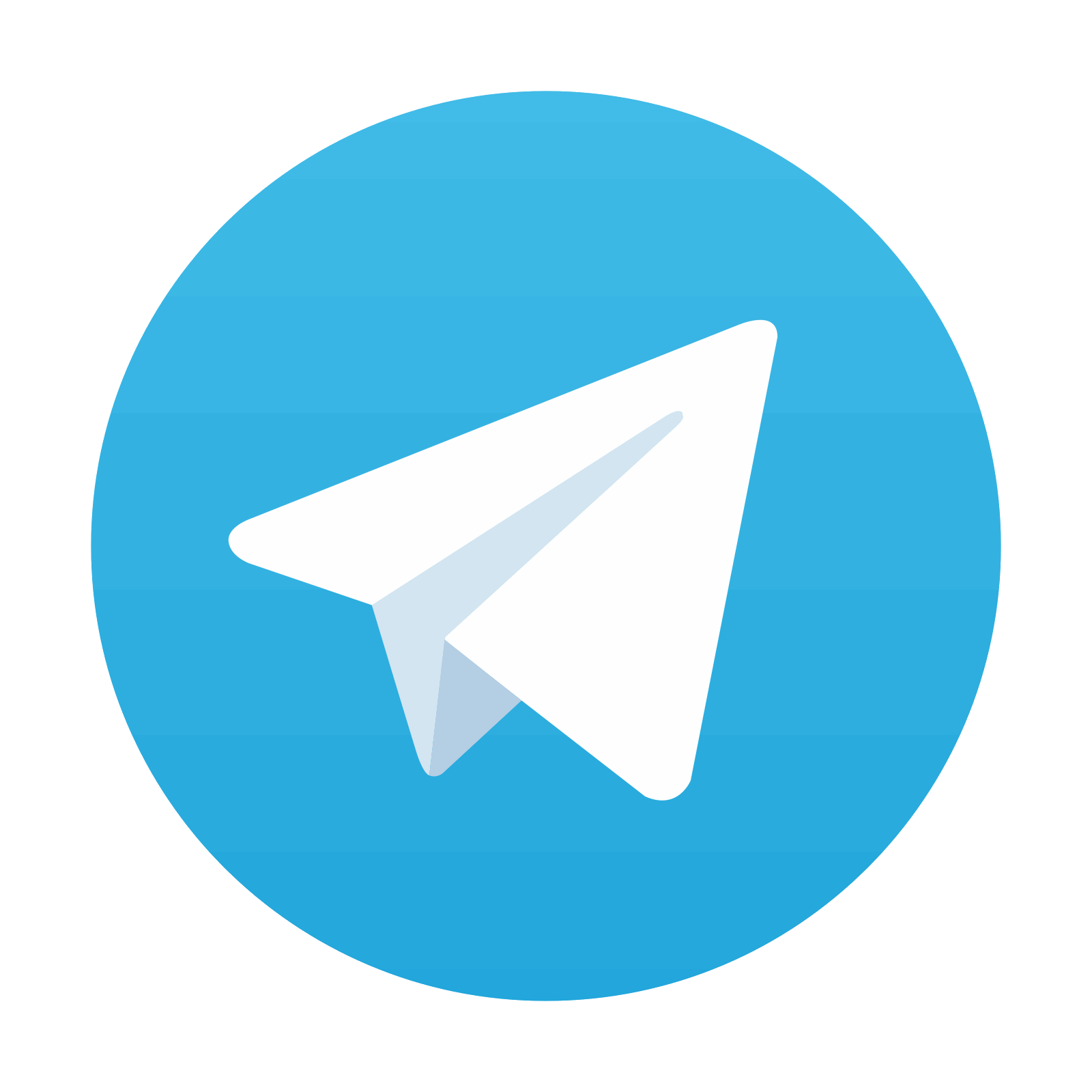
Stay updated, free articles. Join our Telegram channel

Full access? Get Clinical Tree
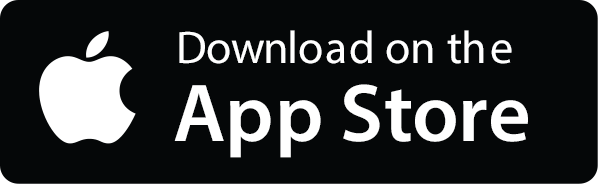
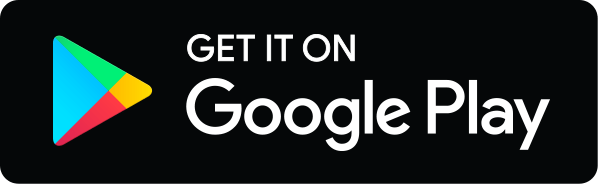