6 J. Glenn Songer, Ashley E. Harmon, and M. Kevin Keel The virulence of C. difficile is mediated by two members of the large clostridial cytotoxin family, toxin A (TcdA) and toxin B (TcdB). An enormous amount is known about these toxins and their variants, as well as about their regulation and the possible effects of accessory toxins and strain differences in the occurrence and severity of C. difficile infection (CDI), but many questions remain. To date, understanding toxin function and the role in pathogenesis has formed the basis for numerous advances in prevention and therapy. With time, it seems likely that abrogating the effects of these toxins will be the major key in allowing prevention and control of CDI. Toxins A (TcdA) and B (TcdB) are the essential virulence factors of C. difficile. The genes, tcdA and tcdB, respectively, are located on a large pathogenicity locus (PALoc) in the bacterial chromosome. Molecular mechanisms regulating toxin production are, in a sense, directly responsible for disease. The toxin region of the PALoc containing the tcdA and tcdB, and accessory genes, is shown in Figure 6.1. Figure 6.1 Genetic organization of the PALoc toxin region of C. difficile showing the tcdA and tcdB genes, and accessory tcd genes. tcdC and tcdR are genes involved in regulating toxin production, and tcdE encodes a holin protein involved in toxin secretion. Sequence differences in the tcdA and tcdB genes are used in PCR-based “toxinotyping”, of which over 30 types are recognized. These differences can have profound effects on toxin production and potency. TcdA is an enterotoxin, and TcdB is a potent cytotoxin in vitro; for many years it was thought, based on hamster studies, that TcdB had little activity in vivo unless there was prior damage to mucosal epithelium. The toxins were thought to act synergistically, with TcdA creating widespread damage to the mucosa that permitted TcdB to affect epithelial cells. There are numerous reports of CDI in patients infected by TcdA-negative, TcdB-positive strains of C. difficile. The long-held paradigm belief that TcdA was the major toxin virulence determinant in CDI, based on the activity of purified toxins and on immunoprotection in hamsters, has been challenged by recent tcdA and tcdB mutation studies, supported by clinical observations. TcdA+ TcdB– mutants do not occur naturally, whereas TcdA– TcdB+ mutants are both fairly common and fully virulent. Strains producing TcdB alone are associated in CDI with both severe, localized intestinal damage and systemic organ damage. Disease associated with tcdB only strains is equally, and sometimes more, severe than disease produced by tcdA+ tcdB+ strains. Thus, TcdB may be responsible for the onset of multiple organ dysfunction syndrome (MODS), an often fatal complication of CDI. TcdB is also primarily responsible for induction of the innate immune and inflammatory responses. Gene mutation studies, supported by clinical observation, have thus changed the paradigm to suggest that TcdB is the major virulence factor of C. difficile. These mutation studies have not involved gene complementation and the conclusions are based on a very specific animal model, which does not fully represent human CDI. Nevertheless, these studies and clinical observations importantly focus possible preventive strategies on TcdB and not on TcdA. Further work is, however, required to understand the relative roles of these toxins in CDI. Toxin synthesis is regulated by an accessory gene regulator quorum-signaling system, mediated via a small thiolactone that can be detected in stools of CDI patients. Interfering with toxin regulation may form the basis for future development of quorum-signaling-based non-antibiotic therapies to combat CDI. TcdA and TcdB consist of single, large polypeptide chains with folds stabilized by disulfide bonds (Figure 6.2). They have no export signature. The mechanism by which these large toxins are secreted from bacterial cells is not yet clear but involves TcdE (Figure 6.1), a holin-like protein. A TcdE mutant was restored to full ability to secrete toxins by complementation with functional TcdE. Three possible translation start sites have been identified, and it has been suggested that each isoform may play a specific role in toxin release by the TcdE-mediated process. The different functional domains of TcdA and TcdB (Figure 6.2) are involved in binding and entry into epithelial cells, glycosylation of Rho GTPase in the cytosol, and N-terminal insertion into the endosome. Figure 6.2 Schematic of the functional domain structure of TcdA and TcdB. The four major domains have N-terminal glucosyltransferase activity (GT), autocatalytic cysteine protease activity (CPD), central translocation (TMD), and C-terminal receptor binding (C) (see text). The carboxy-termini of both toxins include the receptor-binding domain (Figure 6.2); that for TcdA apparently differs significantly from that for TcdB. Carbohydrate receptors for TcdA include Galα1-3β1-4GlcNAc-R (α-Gal epitope), Galβ1-4{Fuβ1-3}GlcNAc (Lewis X), Galβ1-4GlcNAcβ1 (Lewis Y), Galβ1-14GlcNAcβ1-3Galβ1-4(Glc) (Lewis I), and sucrose-isomaltase. These receptors are on the brush border of susceptible intestinal epithelial cells and may occur elsewhere as well. A receptor for TcdB has not been identified, but may be present on the basolateral aspect of epithelial cells. Physical trauma, or the effects of TcdA, may compromise cell–cell contacts, providing TcdB access to receptors that are normally unavailable. The toxin amino-termini are catalytic (Figure 6.2) and are highly conserved; both function in the target cell cytoplasm by similar mechanisms. Receptor binding by TcdA initiates endocytosis by coated pits and the resulting vesicles fuse with lysosomes. The mechanism of TcdB attachment and endocytosis has not been described. Acidification of the endolysosome produces a toxin conformational change, which is followed by its activation and escape into the cytoplasm. In the cytoplasm, the toxins specifically inactivate Rho, and other Rho-subtype GTPases, by glycosylation of threonine. This class of GTPases comprises signal transduction molecules associated with apoptosis and maintenance/regulation of actin filaments. Actin filaments are a necessary part of some cell adhesion molecules (for example, zonula adherens, tight junctions, and focal adhesions) and their disruption results in the loss of cell–cell contacts, in increased paracellular permeability of mucosal surfaces, in cell rounding, and, eventually, in cell death. TcdA and TcdB amplify the disease process beyond simple disruption of function and death of mucosal epithelial cells. Initiation of an inflammatory cascade that can damage host tissues is particularly important. TcdA causes mitochondrial dysfunction, with depletion of ATP and intracellular generation of reactive oxygen intermediates. Degradation of IκB follows degradation and nuclear translocation of NF-κB, with elaboration of IL-8 and possibly other pro-inflammatory mediators. Factors regulating disease severity are poorly understood. Secretion of toxins A and B causes inflammation and tissue damage, and a portion of this tissue damage may be due to an inappropriate host immune response. Toxins A and B, in combination with both bacterium- and host-derived danger signals, can induce expression of pro-inflammatory cytokines IL-1β and IL-23. Studies have shown that IL-1β signaling enhances IL-23 production and could lead to increased inflammation during CDI. IκB is degraded before glycosylation of Rho and is inhibited by antioxidant pretreatment of TcdA-exposed cells. IL-8 is a chemokine associated with mobilization, activation, and degranulation of neutrophils, as well as chemotaxis of basophils and T-cells. Another potent chemotaxin of neutrophils, macrophage inhibitory protein-2 (MIP-2), is elaborated by rat ileal epithelial cells within 30 minutes of exposure to TcdA. TcdA can also directly stimulate neutrophil migration in vitro. Neutrophils play a prominent role in the pathophysiology of CDI by directly contributing to necrosis of host tissues. Inhibition of neutrophil infiltration by blocking the activity of CD18 or MIP-2 reduces lesion severity. Macrophages and monocytes also participate in the inflammatory cascade associated with CDI. TcdA causes lamina propria macrophages to express cyclooxygenase-2 (Cox-2), with subsequent production of prostaglandin E2 (PGE2
Toxins of Clostridium difficile
Introduction
Toxins A and B
Introduction
General features
Genetic regulation of toxin production
Toxin secretion mechanism
Toxin receptors
Receptor binding: subsequent activity
Toxin effects: beyond cytotoxicity
Stay updated, free articles. Join our Telegram channel

Full access? Get Clinical Tree
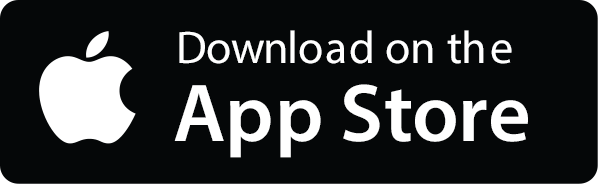
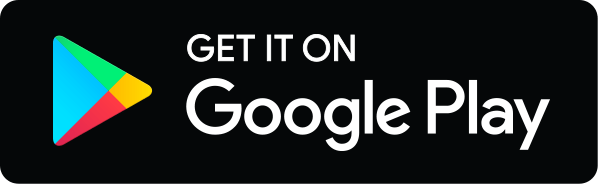