Fig. 4.1
Severe mottling, chalkiness, and attrition of teeth in a fluorotic cow
Excessive intake of fluoride interferes with amelogenesis and dentinogenesis resulting in defective enamel and dentin formation (Swarup and Dwivedi 2002). Ameloblasts, the dental pulp cells, and the odontoblasts are primary target cells in chronic fluorosis. Fluoride has cytotoxic action on odontoblasts and pulp cells (Rodriguez and Rosselot 2001; Chang and Chou 2001). Atrophy and necrosis of the ameloblasts result in defects in enamel. The pulp cells may undergo fibrous and osseous metaplasia. The odontoblasts become atrophic and brown discoloration appears on dentin (Krook and Maylin 1979).
Mottled and defective enamel is an indication of inorganic fluoride exposure during the development of teeth, as adverse effects are not apparent in teeth that have already erupted prior to the fluoride exposure (Susheela et al. 1999). In cattle, the period during which the developing teeth (incisors) are sensitive to fluoride extends from about 6 to 30 months of age (Swarup et al. 2001; Swarup and Dwivedi 2002). Therefore, if cattle are exposed to high fluoride after attaining adulthood, the frequency and severity of dental lesions will be low. Dental lesions do not reflect the current high fluoride intake in adult animals. Once developed, dental lesions largely remain irreversible, although fluoride concentration in teeth may vary with age. Preventive and curative measures for fluorosis in goats in terms of improvement in dental lesions were found effective only when they were taken before eruption of permanent teeth (Wang et al. 1995).
The severity of fluorosis in animals can be classified on the basis of dental examination, particularly the lesions on incisors (Swarup and Dwivedi 2002). Shupe et al. (1979) proposed a 0–5 scale classification system for evaluation of the degree of dental fluorosis in livestock (Table 4.1).
Score | Type | Description |
---|---|---|
0 | Normal | Translucent, smooth, glossy white enamel, teeth are normal in shape |
1 | Questionable effect | Slight deviation from the usual translucency of normal enamel. Cause is not precise. May have enamel flecks; cavities, if present may be unilateral or bilateral, but no mottling is evident |
2 | Slight effect | Slight mottling of enamel; may have some discoloration, but no abrasion. Teeth have normal shape |
3 | Mild effect | Moderate mottling (large patches of chalky enamel), discoloration of enamel; teeth may have slight abrasion |
4 | Marked effect | Definite mottling, discoloration, hypoplasia and hypocalcification; may have pitting of enamel; enamel may be cream colored; definite abrasion of teeth |
5 | Excessive effect | Definite mottling, discoloration, hypocalcification, may have pitting of enamel; enamel may be cream colored, excessive abrasion of teeth |
Another system of classification of dental lesions in fluorotic cattle was described by Krook et al. (1983) which includes five expressions of dental lesions (Table 4.2).
Score | Lesions |
---|---|
1 | Hypercementosis with tooth ankylosis, cementum necrosis, and cyst formation |
2 | Delayed eruption of permanent incisor teeth |
3 | Necrosis of alveolar bone with recession of bone and gingiva |
4 | Oblique eruption of permanent teeth, hypoplasia of teeth with diastema |
5 | Rapid progression of dental lesions and tooth loss |
4.2.2.2 Osteofluorosis
Bone is considered a natural sink for fluoride. In chronic fluorosis in livestock, the bones and joints become enlarged and extra bone growths or exostosis may appear in different parts of the skeleton (Fig. 4.2). Calcification of ligaments and related structures may occur, which is manifested clinically as stiffness and intermittent lameness (Fig. 4.3). The forelimbs are more affected than hind limbs, and in occasional cases, the animal may move around on its knees and assume “knee posture.” Palpable bony exostosis may appear on the metatarsal, metacarpal, mandible, or ribs of cattle and buffaloes (Wheeler and Fell 1983).
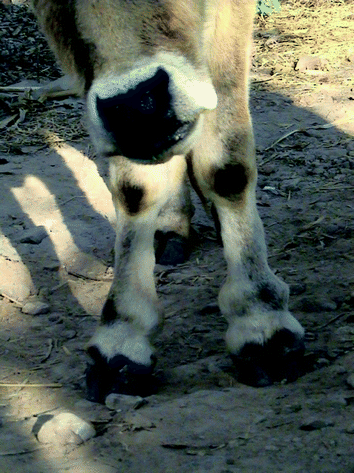
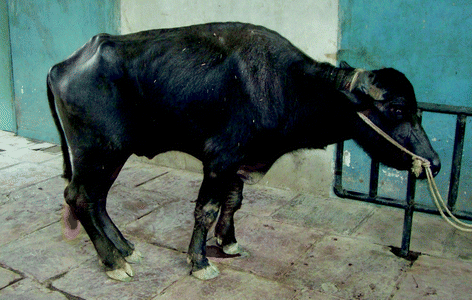
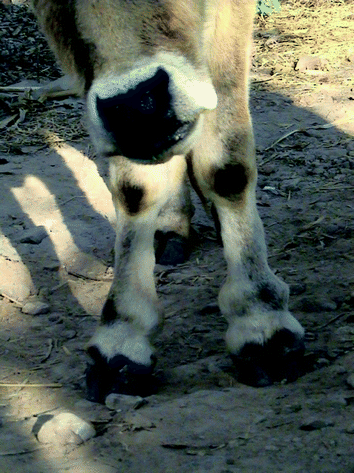
Fig. 4.2
A heifer showing stunted growth, enlarged joints, and lameness. Plasma fluoride concentration was 2.17 ppm
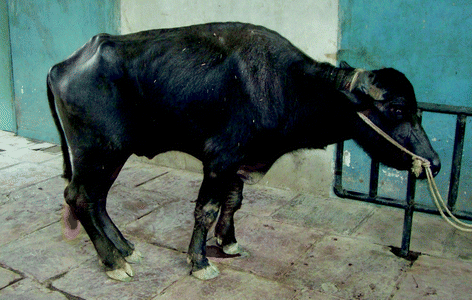
Fig. 4.3
A buffalo showing poor body condition, stiffness, and lameness
Deposition of fluoride in high concentration in bone has been reported in natural (Boivin et al. 1989) as well as experimental (Khandare et al. 2005) fluorosis. Inorganic fluoride uptake by bone tissues is primarily through the replacement of hydroxyl groups of calcium hydroxyapatite and incorporation of inorganic fluoride as calcium fluorapatite. In fluoride toxicity, bony lesions show wide variability including osteosclerosis (increase in bone density), periosteal hyperostosis (increase in the size of the cartilage-capped protuberance at the end of the bone), osteoporosis (decrease in the bone tissue density), osteomalacia (failure of ossification due to decreased availability of calcium ion), or osteophytosis (presence of bony outgrowth). The type and extent of these changes depends upon several factors including nature, dose, and duration of fluoride exposure, age and sex of the subject, hormonal responses, type of bone affected, and nutritional status of the animal. Variation in response is perhaps a cumulative effect of all these factors. Soriano (1968) opined that smaller doses of fluoride stimulate osteogenesis and new bone formation, and larger doses result in increased bone resorption and defective matrix formation. This hypothesis was strengthened by the finding that a high level of fluoride resulted in a reduction in collagen synthesis in humans (Nichols and Flanagan 1966). Poor nutrition, especially with a negative calcium balance was reported to exacerbate osteoporosis (Krishnamachari and Krishnaswamy 1973; Wang et al. 1994). The severity of fluorotic lesions in bone is also influenced by the type of bone and the stress and strain subjected on the bone (Swarup and Dwivedi 2002).
Osteofluorosis can be clinically categorized into four distinct stages in human beings. In asymptomatic preclinical stage I, patients have a slight increase in bone density that could be detected radiographically and bone ash F concentrations range from 3500 to 5500 mg/kg. In stage I skeletal fluorosis, occasional stiffness or pain in the joints and some osteosclerosis of the pelvis and vertebra are observed and bone ash fluoride concentration ranges from 6000 to 7000 mg/kg. In stage III, bone ash fluoride concentrations exceed 7500–8000 mg/kg and pathological changes and clinical signs include dose-related calcification of ligaments, osteosclerosis, exostoses, possible osteoporosis of long bones, muscle wasting, and neurological defects due to hypercalcification of vertebrae. Osteofluorosis in advanced cases is manifested as kyphosis (abnormally increased convexicity in the curvature of the thoracic spine as viewed from the side), scoliosis (lateral curvature of the vertebral column), flexion deformity (the act of bending or the condition of being bent) of knee joints, paraplegia (paralysis of the lower part of the body including legs), and quadriplegia (paralysis of all four limbs). In severe cases, paralysis can occur due to the pressure caused by osteophytes (bony outgrowth) or narrowing of the intervertebral foramen and increase in size of the body of the vertebrae or narrowing of the spinal canal. Men suffer more than women (approximately in the ratio 10:1), perhaps due to more involvement in strenuous work. A higher incidence of bone cancer in men than women was reported in workers exposed to fluoride (Grandjean et al. 1992). Genu valgum (knock knees: legs angle inward at the knee) and genu varum (bow legs: legs are bowed outward at the knee) are two important forms of skeletal fluorosis observed in children suffering from fluorosis in India. This is associated with excess fluoride along with low dietary calcium intake (Kirshnamachari and Krishnaswamy 1973; Krishnamachari 1976).
In animals, normal bone fluoride concentration varies between 200 and 300 mg F/kg and it rarely exceeds 1200 mg/kg in fat-free bone on a dry matter basis. After excess fluoride intake, fluoride deposition in the skeletal tissues proceeds rapidly at first and then more slowly, until eventually a saturation stage is reached. When bone fluoride concentration reaches 15,000–20,000 mg/kg, the capacity to accumulate fluoride by bone is lost. Bony lesions and radiographic changes usually appear when the bone fluoride concentration exceeds 5000 mg/kg. Radiographic changes closely parallel gross pathological changes in bone. Inasmuch as response of bony tissues varies widely, it is quite obvious that the radiographic appearance will show wide variations. The spectrum of radiographic features in fluorosis include increased bone density, blurring or haziness of trabeculae, compact bone thickening, periosteal bone formation, and ossification of the attachments of tendons, ligaments, and muscles (Wang et al. 1994). These changes are more appreciable in the axial skeleton (Christie 1980; Lian and Wu 1986). Despite wide variation, radiography can serve as a valuable tool for the diagnosis of fluorosis. Raj Roholm proposed a method for diagnosis of skeletal fluorosis in humans using bone radiographs. He classified bony changes detectable in radiographs into three distinct stages (Roholm 1937). Later on, Fritz described two additional phases of radiographic changes that could help early diagnosis of osteofluorosis in humans (Frankel 1979). In cattle, the fluorosis can be classified into three stages based upon bone fluoride concentration (Swarup and Dwivedi 2002). In stage I, the fluoride concentration may go up to 2500 ppm without showing any structural or functional changes in bone. Stage II is characterized by a bone fluoride level between 1500 and 5000 ppm. In this stage too, no gross or radiographic changes are evident, but the microarchitecture of the bone shows alterations. In stage III, bone fluoride concentration goes above 5000 ppm and gross lesions become apparent.
4.2.3 Effects on Soft Tissues (Nonskeletal, Nondental Effects)
Excess fluoride uptake can produce deleterious effects on several organs including the gastrointestinal tract, lungs, heart, kidneys, and liver (Perumal et al. 2013). Toxic effects of fluoride on noncalcified tissues and organs are diverse including cytotoxicity, genotoxicity, immunotoxicity, carcinogenicity, and teratogenic effects (El-Nasser et al. 1995; Kleinsasser et al. 2001; Zhan et al. 2006). Many of these effects appear to be associated with free radicals and oxidative stress (Ranjan et al. 2009b; Ekambaram et al. 2010), although some workers have raised doubt over it (Reddy et al. 2003).
4.2.3.1 Cytotoxicity
The cytotoxic potential of fluoride is due to its capacity to inhibit several cellular enzymes, particularly those related to energy metabolism (Barbier et al. 2010). However, Tokunaga et al. (2003) opined that the cytotoxic potential of fluoride is mediated by inhibitory action on glucose uptake by the cells and not by enzyme inhibition. Fluoride exposure disrupts collagen synthesis and leads to the breakdown of collagen in various tissues and organ systems including bone, tendon, muscle, skin, cartilage, lungs, kidneys, and trachea (Susheela and Mukherjee 1981; Sharma 1982).
4.2.3.2 Renal and Hepatic Toxicity
In healthy individuals, about 50 % of ingested fluoride is excreted by the kidneys. The renal system, therefore, appears at high risk of fluoride toxicity. The highest accumulation of fluoride in the kidneys among various soft tissues can be attributed as one of the possible reasons (Whitford 1996). In fluoride-exposed rats, kidney damage has been reported at levels as low as 1 ppm in drinking water (Varner et al. 1998). Fluoride toxicity induces a wide range of structural and functional changes in the renal system. Fluoride inhibits various enzyme systems in the kidneys (Jankaurkar 1974) and decreases Na, K, and ATPase activities (Suketa and Terui 1980). The increase in serum urea nitrogen and creatinine is well documented in fluoride toxicosis in humans and animals (Singh and Swarup 1995; Shivashankara et al. 2000). Histopathological observations in fluoride toxicosis include cloudy swelling, degeneration of tubular epithelia, centrilobular necrosis, atrophy of glomeruli, periglomerular fibrosis, and tubular necrosis (Shashi et al. 2002).
The liver is the organ responsible for detoxification of many endogenous and exogenous toxins. Several studies have revealed an increase in activities of the liver-specific enzymes in the serum of fluorotic animals (Maiti et al. 2004; Upadhyay et al. 2005). An increase in activities of serum transaminases, sorbitol dehydrogenase, and decreased levels of serum total protein and albumin reflecting altered liver function were reported in fluorotic cattle (Arya et al. 1990) and goats (Tsunoda et al. 1985). Hepatocellular necrosis, degenerative changes, hepatic hyperplasia, extensive vacuolization in hepatocytes, and centrilobular necrosis were reported in experimental fluoride toxicity in rabbits (Shashi and Thapar 2000). Histopathological changes of a similar kind have also been reported in experimental fluorosis in calves (Kapoor et al. 1993).
4.2.3.3 Reproductive Toxicity
Reproductive toxicity of fluoride has been widely investigated in laboratory and domestic animals. Experimental studies suggest that fluoride toxicity is associated with morphometric and histological changes in testis (Susheela and Kumar 1991) as well as ovaries and hence reduced fertility in both males and females (WHO 2002). In males, excess fluoride uptake disrupts spermatogenesis as well as hormones regulating sexual maturation and reproduction such as testosterone, estrogen, melatonin, and thyroid hormones (Long et al. 2009). Disruption in steroidogenesis (Ghosh et al. 2002) and spermatogenesis was reported in various animal species including ram (Zakrzewska et al. 2002), rat (Sprando et al. 1998), and rabbit (Kumar and Susheela 1994). Adverse effects on sperm motility, sperm morphology, and semen hyaluronidase activity was also recorded in in vitro studies on bovine semen (Schoff and Lardy 1987; Tanyildizi and Bozkurt 2002).
Females appear less susceptible than males, as reproductive performances did not vary significantly even after exposure to excess fluoride for three generations in mice (Tao and Suittie 1976). It is assumed that chronic fluoride toxicity has no adverse effect on reproductive efficiency of female cattle (USEPA 1980). The absence of teratogenic effects of fluoride in the female rat was also observed in several studies (Collins et al. 1995; Heindel et al. 1996; Al-Hiyasat et al. 2000; WHO 2002). It seems that ill effects observed on female reproduction are secondary to impairment of general body health rather than direct effect of fluoride on reproductive organs. However, some recent studies documented a reduction in reproductive hormone concentrations and abnormalities related to receptor protein expression after fluoride exposure leading to decreased fertility in female mice (Zhou et al. 2013a, b).
4.2.3.4 Neurotoxicity
Several clinical and experimental studies have shown that fluoride induces change in cerebral morphology and biochemical processes in nervous tissues that affect neurological development as well as cognitive processes, such as learning and memory (Valdez-Jimenez et al. 2011; Liu et al. 2014). The developing brain is sensitive to excess fluoride as evident by poor mental development, and low intelligence, learning capacity, and memory power in children and young laboratory animals exposed to excess fluoride (Xiang et al. 2003; Wang et al. 2004). Neurotoxic effects of fluoride on the adult brain resulting in diminished mental acuity, memory impairment, hyperactivity, and cognitive deficits have also been observed in several experimental studies (Ross and Daston 1995; Verner et al. 1998). Concurrent iodine deficiency or exposure to other neurotoxic pollutants may exacerbate the neurological manifestations in fluoride toxicity (Wang et al. 2004).
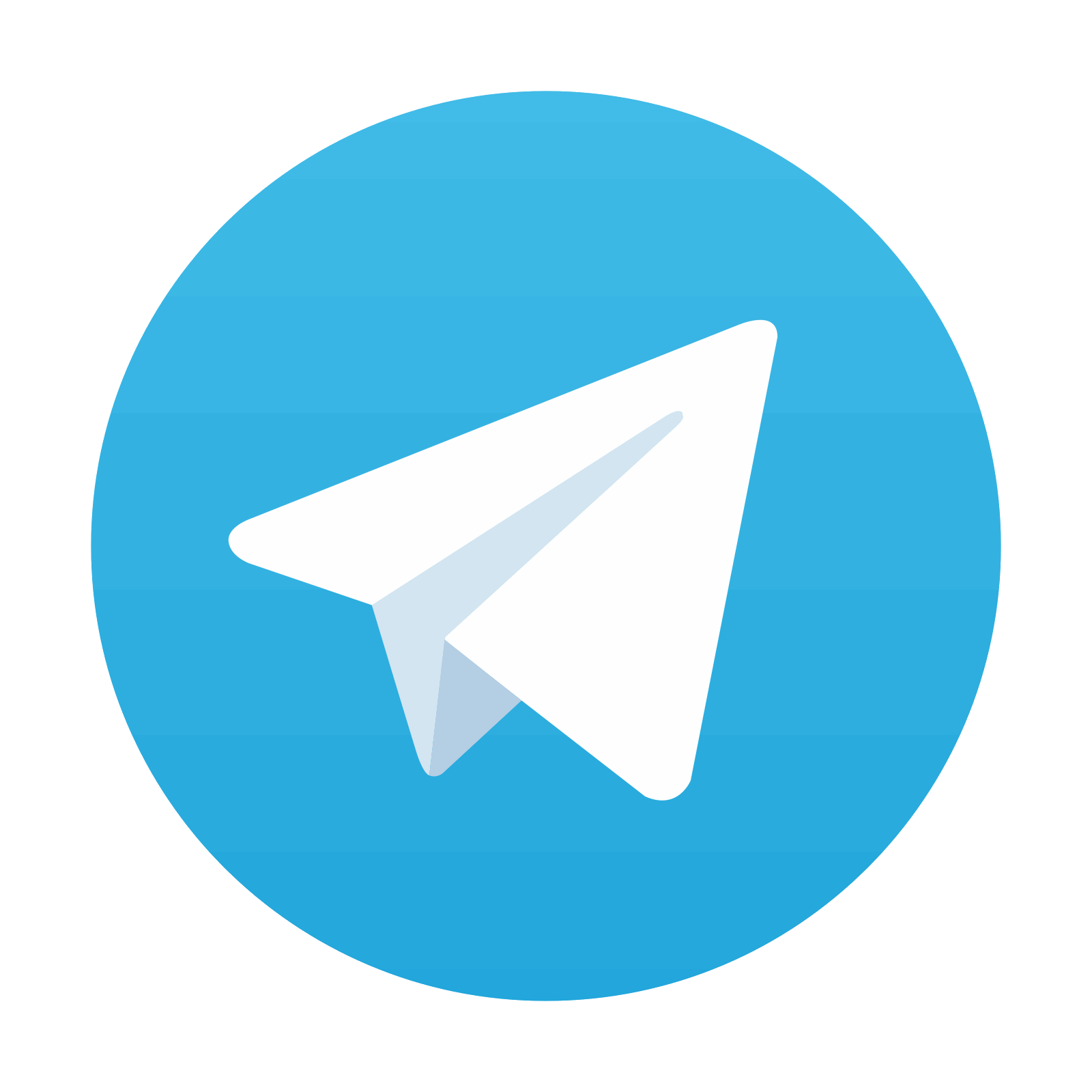
Stay updated, free articles. Join our Telegram channel

Full access? Get Clinical Tree
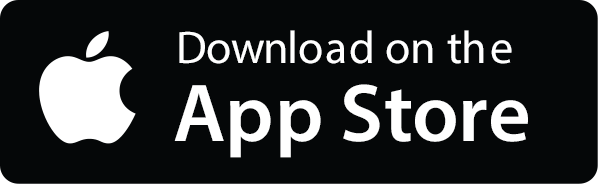
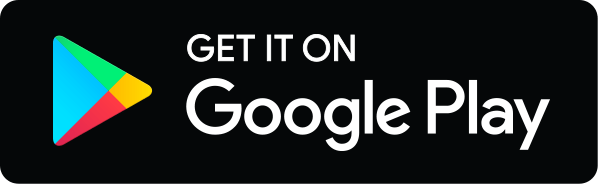