C1–C4
Skull, neck, upper thoracic area
C3–C5
Diaphragm (lower thoracic area)
C5
Shoulder
C5–C7
Elbow
C6
Wrist
C7–T1
Finger
C8
Thumb
T1, T12
Chest wall and abdominal
L2–L4
Hip
L3
Knee
L4–S1
Ankle
L5, S1
Toe
S2–S5
Bladder, bowel and sex organs
1.1.2 Dermatomes
A comparable organization exists for the sensory system where each part of the body is associated with a specific pair of nerves and its spinal cord segment. Cervical segments receive sensory signals from the head (skull), neck, arms, and fingers. Thoracic segments receive inputs from the chest and abdominal area, whereas the lumbar and cervical segments integrate sensory information from the leg, foot, anal, and sex organ areas.
Main dermatomes:
C2 | Skull |
C3 | Rostral neck |
C4 | Caudal neck |
C5 | Clavicle |
C5–C7 | Lateral parts of upper limb |
C6 | Thumb |
C7 | 2nd and 3rd digit |
C8 | 4th and 5th digit |
T4 | Nipple (T4) |
T5 | Mammary fold |
T6–T7 | Xiphoid process |
T10 | Umbilicus |
T12 | Pubic bone |
T12–L1 | Inguinal or groin |
L1–L4 | Anterior and inner surfaces of leg |
L4 | Knee |
L4–S1 | Foot |
S1 | Foot and little toe |
S2–S3 | Sex organs |
S2–S4 | Perineum |
1.2 White Matter
1.2.1 Ascending Somatosensory Tracts
The white matter is divided into the dorsal, lateral, and ventral columns. The first two convey ascending sensory signals, whereas the ventral columns are associated mainly with descending motor signals. Each column is composed of bundles of axons, also called tracts. In the dorsal column, signals associated with touch, pressure, proprioception, and vibration travel from the spinal cord via relatively large myelinated fibers in the gracile fasciculus or the cuneate fusciculus (i.e., depending upon the level of entry) to different areas of the brain. Then, synapses and decussation occur in the medulla prior to being redirected (signals) toward the thalamus and eventually the sensory cortex.
In contrast, the lateral or so-called anterolateral columns convey information about pain and thermal sensation. Synapses and decussation occurs at the spinal cord level (close to the entry of the corresponding primary afferents) prior to ascend to the brain via a number of tracts (see section “Anterolateral (Ventrolateral) System”).
Dorsal Columns
The gracile fasciculus, often referred to as the posterior column-mediated lemniscus system or the tract of Goll (Swiss neuroanatomist), travels dorsomedially in the white matter and carries information about fine touch, vibration, and conscious proprioception from the lower part of the body (T6–S5) to the medulla oblongata (nucleus gracilis).
The cuneate fasciculus, also called the tract of Burdach, is located in the dorsal column just laterally to the gracile fasciculus. The cuneate fasciculus carries to the brain information about touch, vibration, and proprioception originating from the upper part of the body (C1–T6, e.g., arms).
Main dorsal column tracts:
Gracile fasciculus
Cuneate fasciculus
Anterolateral (Ventrolateral) System
Each tract composing the anterolateral system or columns mediates pain and temperature information that originates in different spinal cord regions and ascends to terminate in different areas of the brain. This posterolateral tract (Lissauer’s tract) is a relatively small ascending pathway located next to the cuneate fasciculus. Lissauer’s tract was described by the German neurologist Heinrich Lissauer in the late nineteenth century. It contains information about pain (non-discriminative) and temperature that typically enters in the gray matter one or two segments rostrally for synapsing onto the secondary sensory neuron and decussating contralaterally prior to penetrate again the white matter as part of the ascending spinothalamic tract in direction for the thalamus.
The dorsal spinocerebellar tract, also called the posterior spinocerebellar or Flechsig’s tract, carries information about proprioception after a first synapse in the Clarke’s nucleus. Unlike the ventral spinocerebellar tract, the Flechsig’s tract does not mainly decussate in the spinal cord or elsewhere. The ventral spinocerebellar tract, also called the anterior spinocerebellar or Gowers’s tract (described by Sir William Richard Gowers), conveys proprioceptive information (muscles, joints, etc.) to the cerebellum via one synapse (a primary and a secondary neurons). Decussation generally occurs in the spinal cord as well as in the brainstem (double-cross).
The spinomesencephalic pathway travels in the anterolateral system and ends in the mesencephalic region. The dorsal (posterior or lateral) spinothalamic tract is a pathway mainly composed of sparsely myelinated axons and slow-conducting, unmyelinated axons carrying to the thalamus pain, temperature, information from the skin and other organs. The neospinothalamic tract is one of two subclasses of dorsal spinothalamic tracts. It specifically carries fast pain signals (sparsely myelinated fibers). It decussates in the spinal cord and terminates in most cases in the thalamus (some terminates also in the brainstem, see subclasses below). In turn, the paleospinothalamic tract specifically conveys slow pain signals via unmyelinated C fibers to the brain (most terminate in the brainstem, whereas some terminate in the thalamus).
The ventral spinothalamic tract conveys information to the thalamus from the skin and other organs (itch and crude touch) after synapsing and decussating in the spinal cord and again in the brain (double-cross). The spinotectal pathway conveys information about pain and temperature to the inferior and superior colliculi. It is positioned next to the dorsal spinothalamic tract and decussates at the spinal cord level. The spino-olivary tract is more ventrally located than most of the other anterolateral tracts, and conveys information about muscles, tendons and skin to the olivary nucleus. The spinoreticular tract is located just beside the spinothalamic tract, and carries deep and chronic pain signals to the reticular formation. The spinovestibular tract is located near to the posterior spinocerebellar tract and carries information to the lateral, medial and spinal vestibular nuclei. The interfascicular tract, also called the comma tract of Schultze (discovered by Friedrich Schultze, a German neurologist), is located near the tract of Goll and conveys information about pain and temperature. The spinocortical tract is a bundle of ascending fibers traveling within the descending corticospinal tract conveying information to the cerebral cortex. It originates from all levels of the spinal cord, decussates in the brainstem and terminates in the deep layers of the cerebral cortex.
Main anterolateral system tracts:
Lissauer’s tract
Dorsal spinocerebellar tract
Ventral spinocerebellar tract
Spinomesencephalic tract
Dorsal (postero or lateral) spinothalamic tract
Neospinothalamic tract
Paleospinothalamic tract
Ventral (antero) spinothalamic tract
Spinotectal tract
Spino-olivary tract
Spinoreticular tract
Spinovestibular tract
Interfascicular tract
Spinocortical tract
1.2.2 Descending Motor Tracts
The descending motor system can be divided into the pyramidal and extrapyramidal tracts. The corticospinal tracts also called the pyramidal tracts contain about one million axons on each side that are involved essentially in skilled movements. In contrast, the extrapyramidal tracts are mainly involved in the control of complex behaviors such as postural control and locomotion.
Pyramidal Tracts
This ensemble of axons originates from the cerebral cortex and from some brainstem motor nuclei. It constitutes the most direct descending motor pathway (e.g., some monosynaptic connections) between the motor cortex (Brodmann’s areas 1, 2, 3, 4, and 6) and the final common motor pathway, namely motoneurons in the spinal cord located at all segmental levels. Although often referred to as upper motor neurons, axons composing the pyramidal tracks have their cell bodies in the motor cortex and should accordingly and more appropriately be called corticospinal neurons. The term upper motor neuron may be misleading because they are not really motor neurons although their activation produces movements and, inversely, movement disorders occur when they are injured. About¸ 80–90% of these corticospinal axons are known to decussate to the contralateral side at the pyramid level in the medulla oblongata (also called crossed lateral pyramidal tract). From there, they constitute the lateral corticospinal tract that sends input to spinal motoneurons (ventral horn area). The remaining 10–20% descends ipsilaterally as part of the ventral corticospinal tract and decussate in the spinal cord prior to synapsing with the corresponding motoneurons (also called uncrossed ventral corticospinal tract). Some of the corticospinal neurons form instead the corticobulbar tract that sends input to brainstem motoneurons involved in the control of face, head and neck muscles. An injury at this level is generally associated with the pyramidal tract syndrome characterized by spasticity, paralysis and loss of skilled movements.
Main pyramidal tracts:
Crossed pyramidal tract (large lateral corticospinal tract)
Direct pyramidal tract (uncrossed ventral corticospinal tract)
Corticobulbar tract
Extrapyramidal Tracts
Cell bodies of the extrapyramidal tracts are mainly found in subcortical nuclei in the pons (reticular formation) and medulla oblongata. They send their axons in the spinal cord to motoneurons at all segmental levels. Specifically, the red, vestibular, tectum (superior colliculus) and reticular formation nuclei have cell bodies sending their axons through their respective descending extrapyramidal tract—the rubrospinal, vestibulospinal, tectospinal, and reticulospinal tracts. The former tract descends laterally in the spinal cord, whereas the other three tracts travel ventrally (anterior column). The rubrospinal tract receives inputs from the motor and premotor areas 4 and 6. It sends projections to contralateral (decussation in the brain) motoneurons of the upper spinal cord segments for the control of both fine skilled movements and large powerful ones. It is generally considered to facilitate flexion and inhibit extension specifically in the upper extremities contralaterally. The tectospinal tract sends its axons in premotor lamina of the cervical spinal cord (VI–VIII) and is involved in neck and head motor control. In contrast with the other two tracks, the reticulospinal track sends projections to all levels of the spinal cord. It is involved in the control of autonomic functions (cardiovascular and respiratory functions, blood pressure, micturition, etc.). The vestibulospinal tract sends axons that travel either laterally or ventrally on the ipsilateral side of the spinal cord to premotor laminae VII and VIII. It is known to control muscular contraction levels specifically in extensors. The olivospinal tract originates in the inferior olivary nucleus and innervates contralaterally segments in the spinal cord. Lesioned extrapyramidal tracts are associated with the extrapyramidal syndrome characterized by involuntary movements, muscular rigidity, and immobility without paralysis.
Main extrapyramidal tracts:
Rubrospinal tract
Tectospinal tract
Reticulospinal tract
Vestibulospinal tract
Olivospinal tract
1.3 Gray Matter
As described above, a part of the spinal cord (i.e., white matter) serves as a relay for descending (motor control) and ascending (sensory-related) information between rostral and caudal structures. In recent years, spinal cord physiologists have begun unravelling the existence of other roles of the spinal cord associated mainly with neurons in the gray matter. Some of these neurons form local spinal reflex pathways (so-called reflex arcs), whereas others are part of more complex circuits involved in specific functions. As of today, it is unclear how many different populations (classes) of neurons exist in the spinal cord. However, based on some estimates, it may well exist between one and two billion spinal cord neurons.
1.3.1 Simple Reflex Pathways
A reflex may be defined as a rapid, predictable, repeatable, stereotyped and involuntary motor response or movement induced by a specific stimulus. Most reflexes are mediated locally (within one or two segments) by relatively simple neural pathways (arcs) in the spinal cord—involving generally either one (monosynaptic), two (disynaptic) or more (polysynaptic) synapses and neurons involving interneurons and motoneurons. Note that partially “involuntary controlled” motor behaviors such as locomotion should not be considered as reflexes (i.e., not induced stereotypically and specifically by a corresponding peripheral afferent input). There are two main types of reflexes—autonomic (related with inner organs, eyes, blood vessels, etc.) and somatic (related with skeletal muscle responses). This said, among all reflexes, it is the somatic reflex system mediated in the spinal cord that has been most extensively studied (mainly in cats). Here is a list of spinal reflexes and corresponding reflex arcs for which a detailed description has been provided.
Ia Reflex Pathway
The best known somatic reflex pathway is undoubtedly the Ia monosynaptic reflex arc which is also considered the simplest reflex. It mediates primary afferent (Ia) inputs originating from muscle spindles typically activated by muscle stretching (e.g., also called tendon jerk, tendon tap, or myotatic reflex). It is generally considered to play a role in tonus and postural adjustments. The Ia afferent input enters the spinal cord (dorsally via the corresponding spinal nerve) and establishes monosynaptic connections with homonymous alpha-motoneurons (e.g., soleus Ia afferent input sent to soleus motoneurons) in the gray matter (ventral horn area). This excitatory reflex is known to increase homonymous muscle contraction in response to muscle elongation. In addition to monosynaptic connections with the corresponding homonymous muscle, Ia afferents possess branches that also establish monosynaptic connections with synergistic alpha-motoneurons (e.g., soleus Ia afferent with gastrocnemius motoneurons).
Although generally considered as stereotyped responses, spinal reflexes can nonetheless be modulated. For instance, recent evidence has shown that activity levels in the Ia monosynaptic arc can vary depending upon circumstances, phases, and tasks—e.g., amplitudes of the H-reflex (experimentally induced myotatic reflex) decrease during walking compared with standing quietly. Also, during walking, H-reflex amplitudes are smaller during the swing phase (flexion) than the extension phase (extension). An assessment of Ia reflex pathway function can provide clinically valuable information on neuropathological conditions. A reflex hammer stimulation eliciting an exaggerated (hyperreflexia) or diminished (hyporeflexia) monosynaptic reflex is generally considered as a sign of CNS or PNS damage, respectively.
Ib Reflex Pathways
Also known as the inverse myotatic reflex or autogenic inhibitory reflex pathway, this reflex arc is associated with peripheral afferent inputs from the group Ib afferent fibers (Golgi tendon organs). It has been described initially as inhibiting the homonymous and synergistic alpha-motoneurons at rest although it may remain quiescent in other conditions such as during locomotion. Inputs from the Ib afferents enter the spinal cord and inhibit disynaptically (two synapses involving one inhibitory neuron called the Ib interneuron) alpha-motoneurons. This inhibitory reflex was originally believed to act as a protective mechanism against excessive muscle contraction. However, this view has been challenged since in normal individuals (e.g., not spinal cord-injured), Golgi tendon organs and Ib reflex pathways have been found to mediate inputs throughout a wide range of muscle activity and load. As with the Ia reflex, the Ib autogenic inhibitory reflex has been found to undergo extensive task-dependent modulation. Actually, during locomotor activity, it was found to completely disappear. It is replaced by another Ib afferent-induced reflex pathway that provides extensive excitation of homonymous and synergistic alpha-motoneurons (at all joints of the lower extremities). The candidate excitatory interneurons mediating this disynaptic reflex (and possibly also locomotor-dependent Ia afferent-induced excitation of extensors) is located in lumbar enlargement segments (premotor interneuron in lamina VII) and rhythmically active during locomotion.
Although the role of Ib autogenic inhibition remains largely unclear in resting conditions, the role of the Ib disynaptic excitatory pathway was proposed, during locomotion, to significantly enhance muscular contraction of extensors during the stance phase (20–50% contribution to final force generation). No clinically induced reflex movement has been associated with stimulation of Golgi tendon organs. However, the clasp knife reflex that was thought originally to be mediated only by autogenic inhibition is now believed to be the result of an interaction between Ia monosynaptic excitatory and Ib autogenic inhibitory actions on alpha-motoneurons in spastic patients (e.g., with injured descending tracts).
Flexion Reflex Pathways
Another well-described spinal reflex arc is the flexion reflex afferent (FRA) pathway activated by relatively high threshold fibers (e.g., associated with cutaneous nociceptor A or C fibers, group II, III, and IV muscle afferent fibers, etc.). On the ipsilateral side, it involves at least two interneurons (three or more synapses) in several segments of the spinal cord and alpha-motoneurons of several flexor muscles. It is also called the nocifensive reflex or withdrawal reflex pathway. Specifically, it comprises at least two excitatory interneurons (three synapses) for flexor motoneuron activation and two interneurons (one excitatory and one inhibitory) for extensor motoneuron inhibition. If the stimulus is strong (intense) enough, it may be accompanied by an activation of an accompanying reflex pathway, the crossed-extension reflex arc that produces an extension of the contralateral limb. Contralaterally, additional interneurons (and decussation) are involved for producing the crossed-extension reflex—two excitatory interneurons for contralateral extensor motoneuron activation and two interneurons (one inhibitory and one excitatory) for contralateral flexor muscle inhibition. These reflex pathways are thought to play a role in withdrawal of a limb (unilateral flexion and contralateral extension) from a painful stimulus.
Task-dependent modulatory responses have also been found during locomotor activity since a long-lasting burst of activity is unravelled ipsilaterally during pharmacologically induced fictive locomotion (long-lasting FRA response). The corresponding locomotor-related interneurons have even been proposed to be part per se of the Central Pattern Generator (CPG) for locomotion (specifically part of the flexor portion of a half-center organized-like network) since FRA stimulation under experimental conditions was shown to reset the step cycle to flexion.
Clinically, this reflex pathway, in resting conditions, may be investigated by induction of the Babinski sign (i.e., tongue depressor-induced plantar extension). Although normally found in infants, it is an indication of neurological problems in adults (e.g., spinal pyramidal tracts-induced injury caused by trauma or tumor). The long-lasting FRA response as well as myoclonus can also be uncovered following FRA stimulation in spinal cord-injured patients.
Reciprocal Inhibitory Reflex Pathway
Unlike the other classical reflexes describe above, this reflex pathway is not specifically associated with one type of peripheral afferent input. It has been found in association with the Ia monosynaptic reflex and the Ib autogenic inhibitory reflex, etc. The same principle remains in all cases which is that during a stereotypic response in the main muscle group, the group of antagonist muscles will display the opposite motor response in order to facilitate movement execution. For instance, Ia monosynaptic excitation of soleus motoneurons (extensor muscle) will be accompanied of reciprocal disynaptic inhibition of tibialis anterior motoneurons (flexor muscle) mediated by one inhibitory interneuron (that directly receives input from the primary afferent fiber). A comparable pathway exists for Ib autogenic inhibition which, in parallel, through synaptic connections with excitatory interneurons, will activate the antagonistic populations of motoneurons. Clinically, dysfunctional reciprocal inhibitory reflex pathways have been associated with increased co-contraction. It has been reported in different pathological conditions such as in Parkinson’s disease patients or in patients displaying spasticity.
< div class='tao-gold-member'>
Only gold members can continue reading. Log In or Register a > to continue
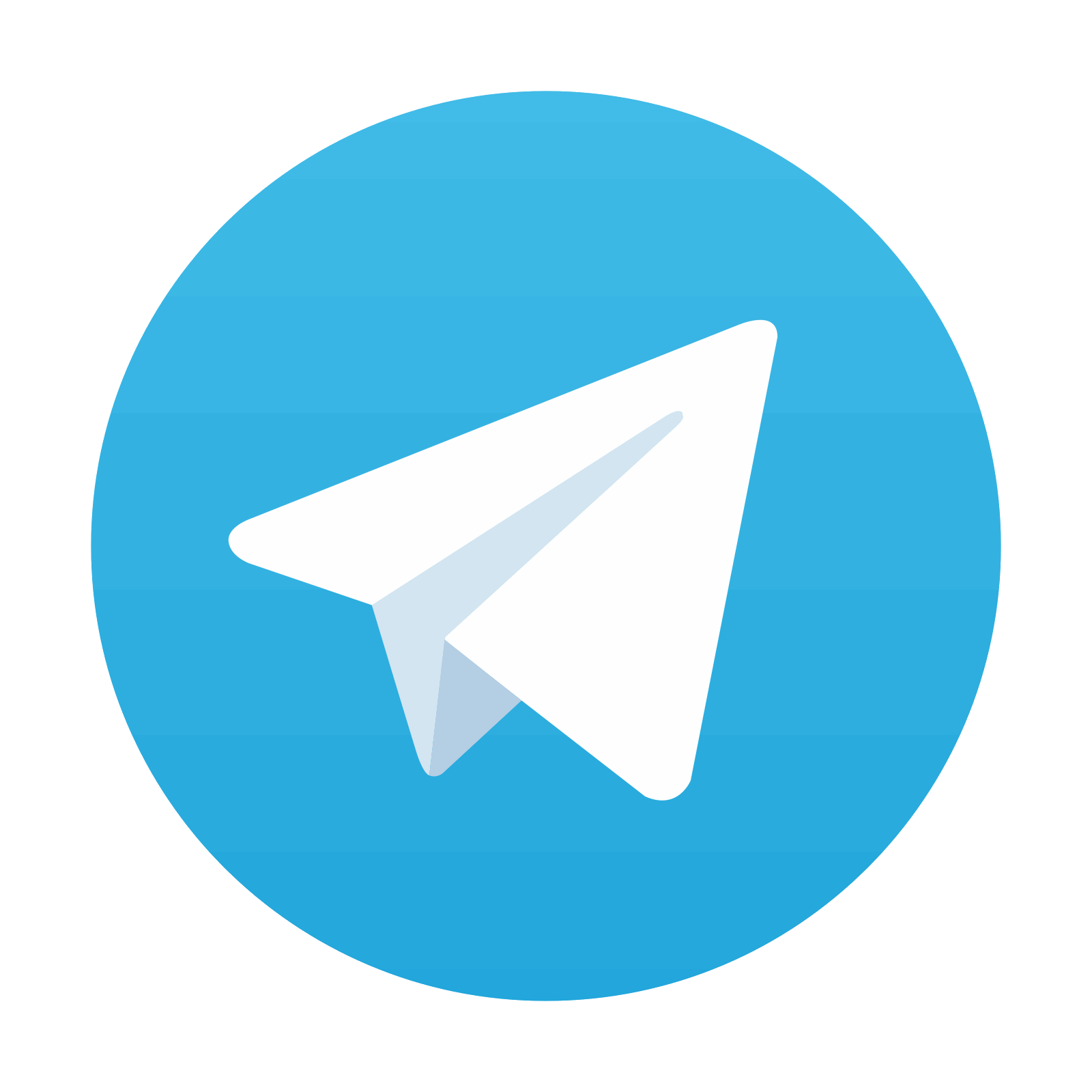
Stay updated, free articles. Join our Telegram channel

Full access? Get Clinical Tree
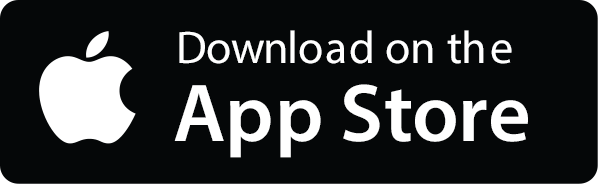
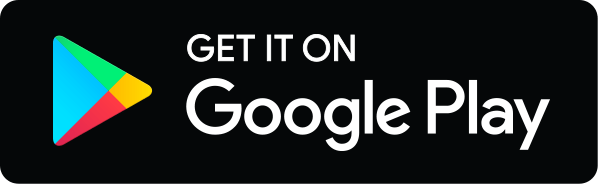