Fig. 6.1
Relative spectral sensitivity of the photopic domestic broiler fowl and the human, normalized to a sensitivity of 100% at 550 nm. (Adapted from Prescott and Wathes, 1999a)
Birds respond to short wavelengths (near UV) and are more sensitive than humans to longer wavelengths in the red-orange range. Overall, they can perceive a broader range of wavelengths. In addition to differences in perception of light intensity, certain colour-mediated visual cues for social or sexual communication may be altered by light source since the spectral outputs (the ranges of wavelengths emitted) of different types of lamps can change the colour appearance of objects. For example, the redness of a flock-mate’s comb may look quite different when illuminated by cool white fluorescent light than when lit by incandescent light. Finally, most sources of artificial light are devoid of UV wavelengths. It has been demonstrated that certain feather patterns are only visible in UV-rich light (Prescott and Wathes, 1999b) and that these may influence mate choice and mating behaviour in broiler breeders (Jones et al., 2001).
6.2.1.3 Motion Detection and Critical Fusion Frequency
Another aspect of the visual system that varies across species has to do with the ability to detect motion. Motion detection depends on the temporal resolution of neurons in the visual system. The flicker-fusion or critical fusion frequency (CFF) is the frequency at which motion can no longer be detected, or in other words, the frequency at which a discontinuous light source (flicker) appears to be continuous. In human beings, the CFF is 60 Hz. This means that at frequencies higher than 60 Hz, images blend together and appear to be continuous – the phenomenon on which the motion picture depends. Birds generally have much better motion detection than do human beings. The domestic fowl’s CFF was initially estimated at around 105 for blue light with lower sensitivities for other colours (Nuboer et al, 1992). This high CFF of fowl led to an interesting question when it comes to lighting of the bird’s environment. Common fluorescent light sources powered by magnetic ballasts flicker at twice the frequency of the electricity supply. In North America the supply frequency is 60 Hz, whereas in Europe it is only 50 Hz. This means that fluorescent lights flicker at 120 and 100 Hz, respectively, in those places. Human beings cannot detect this flicker because it is well above our CFF. Because birds’ CFF is near the flicker frequency of fluorescent light sources it has been speculated that they may be able to see the flicker of fluorescent lighting.
In order to determine whether domestic hens find fluorescent lighting aversive, Widowski et al. (1992) allowed hens to choose between two rooms illuminated respectively by either standard incandescent lighting or a compact fluorescent source during a preference test. The rooms were identical except for type of illumination. Light intensities were similar to those used in laying-hen houses. The hens spent around 73% of the time in fluorescent light and only 27% in incandescent. This meant that either the birds did not perceive the flicker of fluorescent lights, or if they did, they did not avoid it. In fact, they found some aspect of the fluorescent light more attractive than incandescent and spent most of the time in it. Similar results have been obtained from preference tests conducted on turkeys (Sherwin, 1999). It is not clear why birds prefer fluorescent lighting over incandescent, but it probably is due to differences in the wavelengths of light emitted. When hens were given a choice between compact fluorescent lights emitting the same wavelengths but differing only in the rates of flicker (Widowski and Duncan, 1996) the birds spent similar amounts of time in the two lighting conditions. More recently, Jarvis et al, (2002) determined that hens could not detect a flicker of 100 Hz at 100 lx level of illumination. Therefore it is unlikely that any flicker of fluorescent lighting will pose a welfare problem in poultry houses.
6.2.1.4 Lighting Systems and Poultry Welfare
In many poultry production systems, lighting is a critical aspect of the overall management of the birds, and artificial lighting is carefully controlled to maximize production and reproduction. There are three basic aspects to any lighting system that can alter the biology of the birds. These are photoperiod, intensity and type of light source (e.g., incandescent, fluorescent). These different aspects of the light environment can influence the bird either directly through stimulation of photosensitive physiological processes or indirectly by altering behaviour through the visual system. Some photosensitive processes, such as the setting of biological clocks and reproductive function do not require the presence of the eye, as domestic fowl have extra-retinal photoreceptors in the pineal gland and hypothalamus that receive light directly through the skull (Lewis and Morris, 2006). The effect of lighting on the welfare of poultry has been extensively reviewed (Manser, 1996; Prescott et al., 2003a) and only some aspects will be considered here.
6.2.1.5 Photoperiod
The day-length for birds in closed houses can range from just a few hours of light (8L) to continuous or nearly continuous light (23L), depending on the production setting. For birds that are producing eggs (layers, broiler breeders or breeding stock) lighting schedules are dictated by the photoperiods necessary to stimulate and maintain reproduction. In nature, domestic fowl are seasonal breeders and long days (14L) or increasing day-length stimulate gonadotropin release which controls egg laying in hens and spermatogenesis in roosters. A period of darkness is necessary to prevent photorefractoriness which inhibits reproduction.
In growing birds, lighting schedules are much more flexible and a variety of daylengths are used in practice. Traditionally, broiler chickens have been kept in either continuous lighting or a 23L with the notion that these lighting schedules promote feeding, faster growth rates and improved productivity. For the same reason, replacement pullet chicks are often kept on 23L for the first few days or weeks and then their lighting schedules are reduced to approximately 8 h per day until they near sexual maturation. Continuous light may be detrimental to birds in that they are less active overall while at the same time may not obtain sufficient rest. Natural brooding by the hen involves alternating periods of rest and activity, and this cycle of activity may be important for the health of the developing chick (Malleau et al., 2007).
The domestic chick is commonly used as an animal model for vision research and there is an abundance of published work in the biomedical literature that indicates continuous light, continuous darkness and disruption of normal visual stimulation induces abnormal ocular growth. The visual system of chicks begins forming during the first few days of embryonic development and by day E3, embryonic chicks respond to light by increasing movement (Rogers, 1995). Exposure to light during both the pre- and post-hatching stages of development can affect both structural and functional development of the visual system. Light during incubation, for example, results in an increase in the size and weight of the right eye compared to the left, since the left eye is usually obstructed from light by the position of the chick within the egg. This differential exposure to light apparently results in brain lateralization as the right eye plays a dominant role in the ability of chicks to recognize food (Rogers, 1995). Differential exposure to light and other visual stimuli in the early days post-hatch can also influence ocular growth resulting in changes in the size and shape of the eye that affect visual acuity.
Continuous lighting has been shown to lead to eye abnormalities such as corneal flattening and hyperopia (farsightedness) during the first few weeks of post-hatch development (Oishi and Murakami, 1985) and to thinned lens, and retinal and choroid damage over longer periods of time (Li et al., 1995). Interestingly, at least some of the detrimental effects of continuous light are not due to direct exposure of the eye itself to light but rather to a disruption of the circadian rhythm of eye growth patterns. Diurnal growth rhythms have been observed in the chick eye with periods of growth occurring during the day (Weiss and Schaeffel, 1993). This diurnal rhythm disappears when birds are kept under continuous light. Li and Howland (2003) found that covering chicks heads with a hood for 12 h per day while exposing the chicks eyes to continuous light was nearly as effective for reducing corneal flattening and preventing hyperopia as was covering the birds eyes or rearing chicks in 12L:12D. Covering the birds’ heads prevented light from reaching the extra-retinal photoreceptors in the pineal gland, and this appeared to be sufficient for maintaining a circadian rhythm. By exposing chicks to various light:dark cycles Li et al. (2000) found that a minimum of 4 h of continuous darkness was necessary for normal ocular development.
Although the body of evidence from biomedical research indicates that constant light permanently alters the visual system of birds, any long-term effects of lighting schedule during rearing on the visual capabilities of adult birds used for poultry production is generally not known. It may be that the ability to use visual cues for recognizing group-mates, identifying resources or negotiating their environments are affected by the lighting schedules used during the first few days or weeks of rearing.
Broiler chickens can be grown under alternative lighting schedules which have the potential to improve their welfare. Intermittent lighting schedules which involve a repeating schedule of a few hours of light followed by 1 or 2 h of darkness have been shown to be beneficial in reducing leg abnormalities in broilers (Renden et al., 1991). Lighting programs in which birds are initially kept on short days with gradually increasing daylengths have also been shown to be beneficial in reducing leg abnormalities and fast growth problems in broilers (Classen and Riddell, 1989; Classen et al., 1991; Renden et al., 1993). The effects of these programs on reducing welfare problems may be twofold; the birds eat less during the short days so that growth rate is slowed leading to more normal development of the skeletal and cardiovascular systems in relation to muscle tissue; and the birds are more active during the shorter light periods which promotes exercise. While the use of specialized lighting programs for reducing fast growth problems seems promising for improving broiler welfare, these types of programs can only be applied when birds are kept in closed houses under artificial lighting. In many areas of the world, broiler chickens are reared in open sheds or naturally ventilated houses where birds are exposed to natural daylight and seasonal photoperiods.
6.2.1.6 Intensity
Lighting levels in poultry houses are often kept extremely low in order to reduce energy costs, to improve the feed conversion ratios and to control behaviour problems such as feather pecking and cannibalism. At low light intensities birds are less active and use fewer calories for maintenance. The average light intensities used in broiler and layer houses generally range from 3 to 30 lux (Prescott et al., 2003). However values within a house can be quite variable. For example, Prescott and Wathes (1999b) measured illumination levels ranging from less than 2 to over 200 lux for individual hens within a layer house, depending on their location. Thus, illumination levels that birds experience in closed houses are considerably lower than those which occur in bright sunlight (100,000 lux), on overcast days (1,000 lux) or even at twilight (10 lux). Because domestic fowl rely so heavily on vision, keeping them at low light intensities raises welfare concerns because it essentially deprives them of sensory input (Manser, 1996).
A variety of operant and preference tests have been used to demonstrate that domestic fowl will work for light and have preferences for different levels of illumination. Both broiler and layer strain hens were willing to work in an operant system for light (15 lux) (about 4 h per day) Savory and Duncan (1982/83) and this usually was associated with feeding. Those same birds however were not willing to work for darkness. When hens were offered the choice to eat in light levels of <1, 6, 20 or 200 lux, they spent the most time eating in the bright light (39%) although they still spent 14% of total feeding time in the dimmest light (Prescott and Wathes, 2002). Hens were also more willing to press a switch to access feed in the bright compared to the dim light, but when the task was made more difficult by increasing the number of pecks required to feed in the bright light, the hens were not prepared to work for it. Their feeding efficiency (pecks/min and food consumed) was lower at <1 lux compared to any of the other higher light levels. Thus it appears that while hens have a preference for feeding in brighter light, their preference is not that strong.
Preferences for different intensities have been shown to change with the age of the bird (Davis et al., 1999). Given free choice of lighting levels of 6, 20, 60 and 200 lux, both broiler and layer chicks spent most time in 200 lux at 2 weeks of age but by 6 weeks they spent most time in the dimmest light. Low light levels may be especially problematic in aviary systems in which hens must be able to negotiate perches and change levels safely, since bone breakage due to trauma is prevalent in these systems. Hens trained to jump between perches, took longer to perform the task, were less likely to complete a jump and vocalized more during tests, when light intensities were low (< 1 lux compared to 35–40 lux) (Taylor et al., 2003). While it might be argued that birds should be kept in light intensities that are high enough to allow them to perform their full behavioural repertoire, behaviour problems such as feather pecking and cannibalism occur more frequently at higher light intensities (Kjaer and Vestergaard, 1999) and low lighting levels are an effective way of reducing these problems.
6.2.1.7 Light Source
The differences in spectral sensitivity have several implications for lighting of poultry houses. Standard measures of light intensity (lux) are based on the human spectral sensitivity; the intensity of different light sources that birds perceive are likely to be very different from the intensity indicated by the standard light meter. Therefore, the use of alternative units for estimating perceived light intensity by domestic fowl that account for differences in spectral sensitivity (“clux”) have been proposed (Nuboer et al., 1992; Prescott and Wathes, 1999a).
6.2.2 Chemical Senses
The chemical senses, which allow animals to detect various chemical stimuli in the external environment, fall into three types: olfaction or smell, gustation or taste and chemesthesis, also referred to as the common chemical sense. Olfaction generally refers to the ability to detect airborne chemical stimuli. Gustatory receptors responsible for the sense of taste are usually stimulated when in direct contact with a relatively large number of chemical molecules. Trigeminal receptors, which are a major component of the chemesthetic system, are free nerve endings located in various mucous membranes in the cranium that respond to chemical irritants as well as to mechanical and thermal stimuli. This system activates reflex and avoidance responses to potentially damaging chemical stimuli. There is often close proximity among receptors of the three systems and some chemicals may stimulate more than one of these chemical detection systems (Mason and Clark, 2000).
Until recently, chemoreception in domestic fowl has not been considered to be important because of the notion that birds in general have limited capabilities in responding to chemical stimuli (Jones and Roper, 1997). However, there is now a large body of evidence from neuroanatomical, neurophysiological and behavioural studies that the chemical senses do play a functional role in birds and specifically in domestic fowl (Jones and Roper, 1997; McKeegan, 2004a). Because poultry are exposed to a variety of odours and gasses during production in poultry houses and during gaseous stunning at depopulation and slaughter, their perception of chemical stimuli has important implications for their welfare.
6.2.2.1 Olfaction and Chemesthesis
Birds have a fully developed olfactory bulb and share some of the same anatomical and physiological features of the main olfactory system of mammals. Their olfactory system consists of receptor cells arranged along the epithelium that lines the nasal cavity. This system allows detection of molecules in the stream of air that flows over the nasal passages and the detection of chemical stimuli within the nasal cavity. The anatomy of the trigeminal systems of birds is also similar to that of mammals, although birds and mammals may respond to very different chemical stimuli. In domestic fowl, branches of the trigeminal (5th cranial) nerve receive input from eyes, nasal cavity and mouth. Free nerve endings of the trigeminal system are closely associated with gustatory receptors in the mouth and palate and with olfactory receptors in the nasal mucosa (Mason and Clark, 2000).
Although the anatomy of avian olfactory and trigeminal systems has been known for some time, neurophysiological studies of the responses of domestic fowl to different chemical stimuli have only recently been done. McKeegan (2002a) measured electrical activity in single neurons of the olfactory bulbs of laying hens in response to the odours of geraniol, limonene and clove oil (standard stimuli in tests of olfaction) and ammonia. All of the odours were capable of causing either inhibition or excitation of spontaneous firing in some neurons, but ammonia most commonly evoked a response compared to the other odours. The firing response patterns observed in the hens were intermediate to those previously reported for mammals and reptiles. In a similar study McKeegan et al (2002) measured electrical activity of olfactory bulb neurons in laying hens exposed to different concentrations of ammonia and hydrogen sulfide, two air pollutants commonly found in poultry houses. The stimulus-response curves observed indicated that the olfactory system of hens is able to distiguish differences in concentrations of both of these gasses. McKeegan et al. (2004b) also demonstrated concentration-response curves for trigeminal nerves in the nasal mucosa and palate of laying hens to ammonia, carbon dioxide and acetic acid vapour which indicated that the trigeminal system is able to detect all three of these compounds. In follow up to the neurophysiological studies, McKeegan et al (2005) measured the behavioural responses of hens to brief (7s) pulses of varying concentrations of ammonia (5–100 ppm), hydrogen sulfide (1–20 ppm) and carbon dioxide (10–80 ppm). The hens interrupted their previous activity during exposure to all three of the gasses but showed distinct responses to each gas. They oriented to source of the odour at all concentrations of ammonia and hydrogen sulfide (olfactory detection) but not to CO2 (trigeminal detection) and responded to CO2 with mandibulation (rapid opening and closing the beak) and gasping. Hens only showed avoidance and eye shutting in response to hydrogen sulfide and blinking only in response to ammonia. Thus, the neurophysiological studies indicate that hens have the ability to detect these gasses, and the behavioural studies indicate that they also perceive them and respond to them in different ways.
6.2.2.2 The Effect of Atmospheric Ammonia on the Welfare of Birds
Ammonia is the most common air pollutant in poultry houses, and it can cause reduced growth, increased susceptibility to respiratory disease and inflammation (or permanent damage) of the cornea and conjunctiva when it occurs at relatively high concentrations (see review by Kristensen and Wathes, 2000). The most commonly recommended maximum concentration for poultry houses is 25 ppm, which is based on human safety guidelines rather than any measures of poultry welfare.
There have been a few studies aimed at determining whether domestic fowl will actively avoid environments with high levels of atmospheric ammonia. Laying hens given the choice among compartments with ammonia concentrations of 0, 25 or 45 ppm spent significantly more time foraging, preening and resting in the fresh air compared to the other two compartments (Kristensen et al, 2000). However, even though the hens were observed most often in the compartment at 0 ppm (approximately 42% of observations), the majority of time they were observed in the higher ammonia concentrations (29 and 29% for 25 and 45 ppm, respectively). In two separate experiments, female broiler chickens given free choice of compartments with atmospheric ammonia concentrations of 4, 11, 20 and 37 ppm spent 80–90% of the time at the two lower concentrations. These studies indicate that the threshold for avoidance of ammonia by domestic fowl may be lower than the 25 ppm commonly recommended, but the aversiveness of varying concentrations still needs to be determined. Ammonia levels much less than 25 ppm may be difficult to achieve in practice, especially in deep litter houses in cold climates. For example, mean ammonia concentrations in poultry layer and broiler houses measured over different seasons in Northern Europe ranged from 5 to 30 ppm with a number of houses exceeding the recommended 25 ppm (Groot Keerkamp et al., 1998).
6.2.3 Hearing
The auditory receptor cells in the inner ear encode both the frequency (pitch) expressed in Hertz (Hz), and the intensity (loudness) of sound expressed in decibels (dB). There are two different aspects of sound perception by animals that have received a great deal of study: range of hearing and ability to localize the source of a sound. Most sounds or auditory signals relevant to animals are not composed of pure tones, of course, but rather are a complex mixture of different pitches and intensities.
The range of hearing refers to an animal’s ability to detect sounds of different frequencies. The ears of birds differ from those of mammals in several respects. Birds generally lack an external auricle (pinna) and their middle ear is anatomically different and generally less specialized than that of mammals (Necker, 2000a). In general the range of hearing in birds is narrower than that of mammals. Although their hearing is not as sensitive as mammals’, most birds nevertheless use a complex repertoire of calls and songs for vocal communication. Approximate perceivable sound-frequency limits for domestic fowl based on discrimination trials are roughly 500–6,000 Hz (Temple et al., 1984), which corresponds to the frequencies of most vocalization given by the birds. Their most sensitive range is between 3,000 and 5,000 Hz.
6.2.3.1 Effects of Noise on Welfare
Noise can be defined as sound that is noxious or unpleasant. There are several sources of noise in animal environments that can be either aversive or cause physical damage to the auditory system. They include sounds associated with feeding and cleaning operations, which may be especially problematic with automated systems. Noise may be generated by the animals themselves, either from vocalizations or from banging and rattling feeding and caging equipment. Heating and ventilation equipment (especially fans) are a common source of sonic and ultrasonic noise in animal accommodations. During transportation, vehicular noises also can be a problem.
Durham et al. (2002) recorded average sound pressure levels of 90 dB in both egg layer and broiler breeder barns with the majority of the sound either below 100 Hz or between 700 and 7,000 Hz. This is approximately the same noise level experienced when running a lawn mower, driving a tractor or operating a chainsaw. The maximum allowable exposure limits for workers in Canada is between 85 and 90 dB for an 8-h workday. Durham et al. (2002) also identified evidence of severe cochlear damage in broiler breeders and subsequently found a breed difference in cochlear damage between broiler breeder and layer strains from commercial barns. Rearing broilers in quieter laboratory conditions resulted in less damage (Smittkamp et al., 2002). Therefore it appears that broiler strains may have a genetic predisposition to auditory damage and that the noise levels common under commercial conditions can cause the damage. Outside of the biomedical literature, hearing loss due to noise in commercial poultry houses has not been studied, and any consequences for behaviour and welfare needs to be determined.
Another situation that may involve high levels of noise is during transport. However, when birds were trained to peck a key to alter either noise, or a combination of noise and motion (simulating transport), the birds only changed their pecking rate to alter motion and noise and therefore did not find the noise aversive in this case (Nicol et al. 1991). However the noise exposure was simply that of a motor and neither the frequency, intensity, or similarity with what a bird would experience during actual transport, were described.
6.2.4 The Beak as a Sensory Organ
Birds use their beaks in a wide variety of ways including foraging, feeding, drinking, preening, nestbuilding, arranging eggs in the nest and as a weapon during aggressive encounters and predator evasion. Therefore the beak serves not only as a tool for pecking, grasping and moving objects but also as a highly specialized sensory organ for discriminating food from non-food items, detecting appropriate sources of water and generally exploring the physical and chemical properties of the environment. In order to serve these functions, the beak is highly innervated with gustatory receptors, mechanoreceptors, which detect pressure and touch, thermoreceptors, which detect changes in temperature, and nociceptors, which detect intense or noxious mechanical and thermal as well as painful stimuli (Necker, 2000b).
The anatomy, physiology and function of the beak of domestic fowl have been studied in great detail (Gentle, 1985; Gentle and Breward, 1986; Lunam, 2005). The structures of the upper and lower beak are supported by the premaxillary and mandibular bones, respectively. The external surface of the beak is covered by a tough layer of keratin tissue. Below this keratin layer lies a layer of epithelial cells, forming the epidermis. Between the epidermis and the bone is the dermis, which is supplied by numerous veins, arteries, nerve fibres and sensory receptors. The bill tip organ, an area characterised by 15–20 small projections (dermal papillae) that are densely packed with mechanoreceptors, is considered to provide fine tactile discrimination for feeding. In many avian species such as geese and ducks, bill tip organs are located at the ends of both the upper and lower beaks, but in domestic fowl the bill tip organ is only present on the lower beak. However, specialised encapsulated mechanoreceptors, the Herbst and Grandry corpuscles, are also located near the tip of the upper beak, decreasing in number from the tip to the nares. In addition to the mechanoreceptors, numerous free nerve endings that respond to thermal, chemical and painful stimuli are located in the tip of the upper beak and the bill tip organ. Removing the highly innervated tip of the beak therefore, results in significant deprivation of sensory input and is one of the major welfare concerns regarding beak trimming. The taste buds of domestic fowl are found primarily in the palate and floor of the oral cavity in close proximity to salivary ducts, with fewer taste buds located on the tongue.
6.2.5 Effects of Experience on Locomotory Development
Experience with aspects of the physical environment during rearing can have permanent effects on the locomotory abilities of animals. For example, laying hens that do not have opportunities to perch as chicks often have difficulty adapting to commercial aviary systems where nest boxes, feeders and drinkers are arranged on different levels off the ground and must be accessed by perches (Appleby et al., 1988). Some of the difficulty may arise because of physical deficiencies – the birds simply have not developed the muscle mass or bone strength to perform the necessary motor patterns. However there is also evidence that the lack of experience during early stages of development results in a deficiency in the cognitive spatial skills necessary for negotiating three-dimensional space (Appleby and Duncan, 1989). If an animal is to be kept in a relatively complex environment as an adult it must have the opportunity to develop the required locomotory skills early in life.
6.3 Thermal Biology of Domestic Fowl
6.3.1 Heat Balance and Modes of Heat Exchange
Domestic fowl are homeotherms in that they maintain a constant body temperature within a range of environmental conditions. Their deep body temperature in the brain and visceral organs is around 41°C, but the temperature of peripheral areas of the body can vary widely, as the birds use warming or cooling of the extremities as one means to control heat flow to and from the environment. Regulation of body temperature involves numerous physiological and behavioural mechanisms that serve to maintain the balance of heat produced from metabolism with gains and losses to the environment. The relationship of heat exchange between the body of the bird and the environment is described by the classic heat balance equation:


Where Δ= gain or loss of body heat; H = heat production; E = heat lost through evaporation; R = heat gained or lost though radiation; K= heat gained or lost through conduction; and C = heat gained or lost through convection (Dawson and Whittow, 2000). Thus if metabolic heat production is greater than the amount of heat that can be lost to the environment, body heat, and consequently body temperature will rise. Conversely, if more heat is lost to the environment than is produced by metabolism, body heat and body temperature will drop. When environmental conditions are such that no energy expenditure is necessary to maintain body temperature, the bird is considered to be in its thermo-neutral zone (Hillman et al., 1985).
The rate of heat production is a function of the basal metabolism together with excess heat that is produced when birds are feeding, active, laying down tissue during growth or producing eggs. The amount of heat produced when a bird is fasting, resting and in thermo-neutral conditions is determined by its basal metabolic rate, in which virtually all of the energy that is involved in oxidative reactions required for bodily functions are released as heat in the tissues. Basal metabolic heat production depends on metabolic body size, which can be estimated from the bird’s body weight. Ingestion and the digestive processing of food produces heat and this heat increment of feeding varies with the type of foodstuff. When energy intake is greater than the amount of energy used for basal metabolism, which is usually the case for poultry in production, the bulk of that energy is converted into product. However, since the conversion of feed energy to product is never 100% efficient, the excess energy is given off as heat. The total heat production rate of a bird can therefore be estimated from its body weight, metabolisable energy intake and feed conversion efficiency. Calculations for ventilation rates of closed poultry houses are largely determined from estimates of the birds’ total heat production rates. By using, the number of birds in a house, their body weights and their estimated caloric intakes we can estimate how many calories of heat they produce, and therefore how much warm air needs to be exhausted from a building in order to maintain the building within an acceptable temperature range.
Since living tissues constantly produce heat, it is essential that some of that heat be transferred to the environment. The modes of heat flow between the bird’s body and the environment are divided into the sensible modes, in which heat energy is transferred directly along a thermal gradient and the latent mode, evaporation, in which heat energy is absorbed during the conversion of water from liquid to vapour. The sensible modes – radiation, conduction and convection – depend on a measurable temperature difference between the surface of the body that is involved in the exchange of heat and temperatures of the bird’s surroundings. Evaporation, on the other hand, depends on a vapour pressure gradient between the evaporative surface of the body and the air and is therefore affected by humidity, which reflects the water holding capacity of air.
Radiation involves the transfer of heat energy without a medium, such that warmer surfaces will radiate heat energy to cooler surfaces. For poultry housed indoors, heat from the warm surfaces of the bird’s body will radiate to any cooler surfaces that they are exposed to such as the walls or ceiling of the barn. In the case of radiant supplemental heaters or uninsulated roofs that are heated by the sun, the heat energy from lamps or hot surfaces will be directly transferred to and absorbed by the bird’s body. In outdoor conditions, birds will radiate heat to the sky and any cooler objects in the environment and will absorb radiation from the sun and warmer surroundings such as the ground. These radiant heat exchanges occur independently of air temperature.
Conduction involves the transfer of heat energy through a medium. It too depends on a thermal gradient but unlike radiation, conductive heat exchange requires physical contact between the surfaces involved in the heat exchange. The rate of conductive heat flow depends not only on the temperature gradient, but also on the thermal conductivity of the contact surface. The reciprocal of thermal conductivity is the insulation value of substance, which is described by its r-value. Substances such as water, metal or concrete have high thermal conductivities and readily transfer heat. Air has an extremely low thermal conductivity, and materials that are porous and effectively trap air such as polystyrene, straw or feathers have the lowest rates of heat transfer.
Convection involves the transfer of heat through moving streams of air (or water). Natural convection occurs because of thermal buoyancy so that even in still air, some heat is transferred from the surface of the body to the surrounding air, which then warms, rises and carries away the heat. A stationary layer of air, the boundary layer, surrounds the body and provides insulation. In drafts or windy conditions (forced convection) heat loss from the body increases significantly as this boundary layer is disrupted and the air surrounding the body is warmed and carried away much more rapidly. The rate of heat transfer by convection is determined by the combination of air temperature and air velocity. The combination of the two factors on heat loss is described by what is commonly referred to as the wind chill factor.
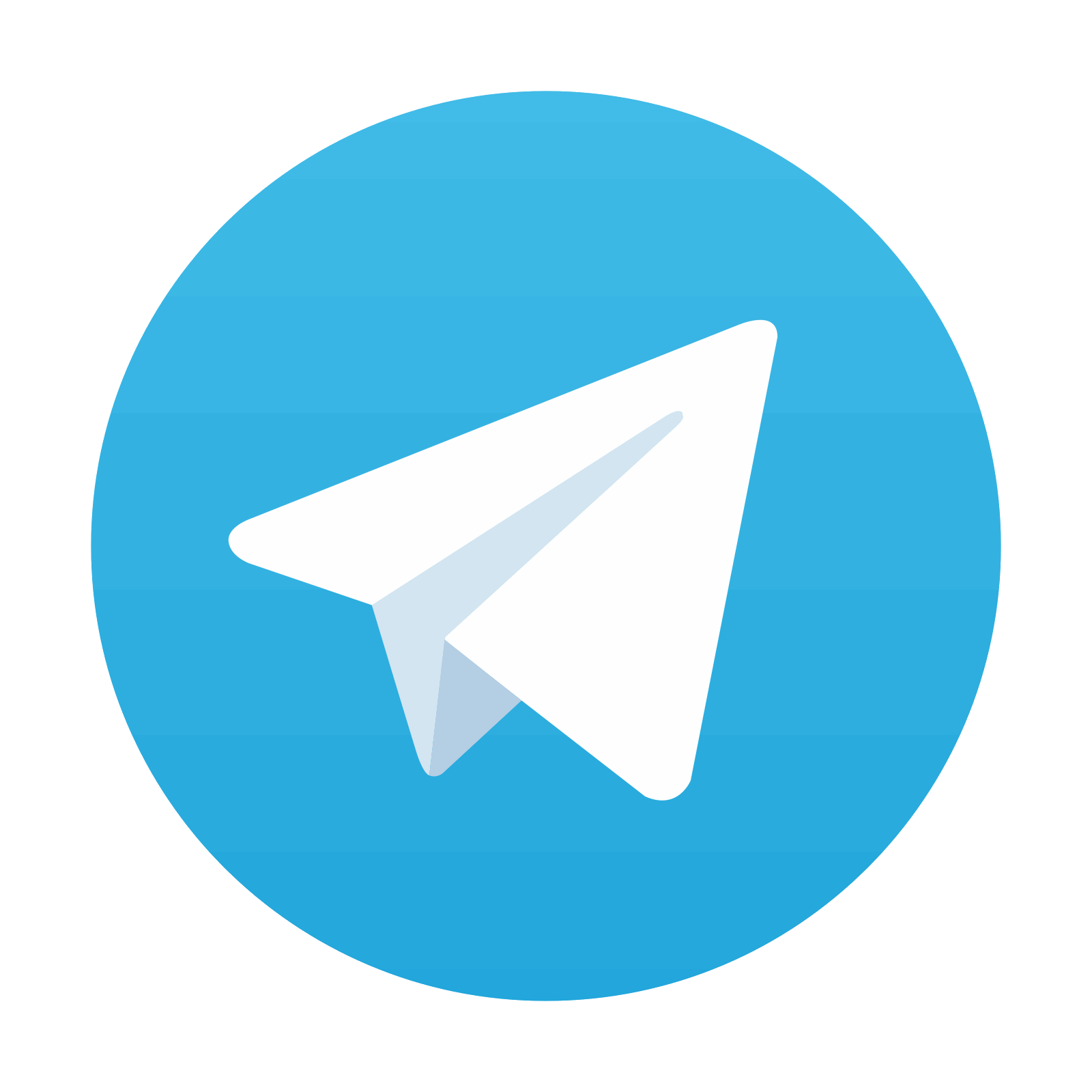
Stay updated, free articles. Join our Telegram channel

Full access? Get Clinical Tree
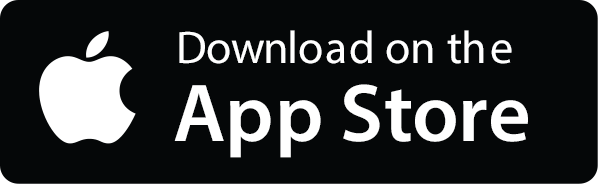
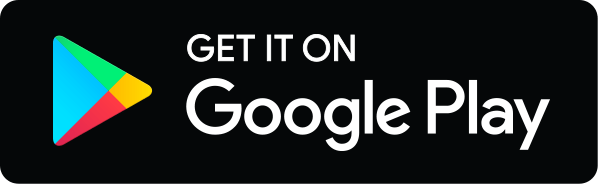