Fig. 1.
Hyperflexion injury is typically associated with an anterior spinal cord syndrome, with spastic paraplegia and hypalgesia and sparing of posterior column sensation (LCST lateral corticospinal tract, LST lateral spinothalamic tract, AST anterior spinothalamic tract).
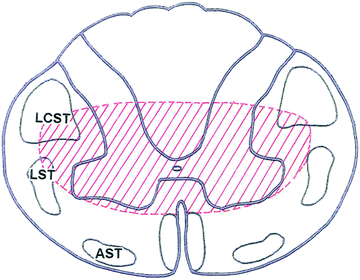
Fig. 2.
Hyperextension injury leads to a central spinal cord syndrome, which is characterized, in cervical injuries, by spastic weakness that is more prominent in the upper than in the lower extremities and by hypalgesia with variable loss of posterior column sensation.
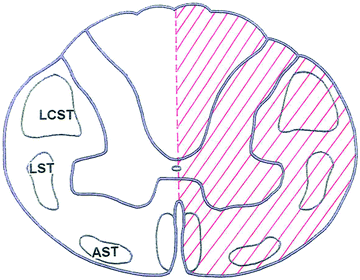
Fig. 3.
The Brown-Séquard syndrome, i.e., ipsilateral weakness and loss of posterior column sensation with contralateral hypalgesia, is usually the result of a penetrating injury such as a stab wound.
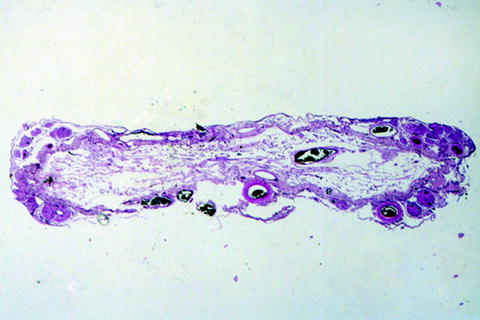
Fig. 4.
Spinal cord at T10 in a man with a complete spinal cord syndrome, 39 years after a shell-fragment injury to the spine. Only the leptomeninges and leptomeningeal blood vessels are visible; no spinal cord parenchyma remains (hematoxylin and eosin stain).
2.1 Penetrating Injuries
Penetrating injuries are those in which the integrity of the dura and leptomeninges are breached by the injuring agent which, in peacetime civilian life within the United States, is most frequently a bullet or a sharp object such as a knife blade. The resulting neurological deficits will depend heavily upon the extent and level of the injury. Missiles may penetrate the spinal cord directly or injure it by driving bone fragments into it. Closed injuries may also breach the dura and leptomeninges by producing vertebral fractures and driving bone directly into the spinal cord parenchyma.
2.2 Nonpenetrating Injuries
Acutely fatal injuries tend to occur at high cervical spinal cord levels and may show very little in the way of dramatic pathological findings, as the continuity of the vertebral column and spinal cord is typically restored immediately following the injury (6). Damage to the vertebral column should alert the examiner to the possibility of structural damage to the spinal cord proper. Surprisingly little may be seen (7). Epidural, subdural, or subarachnoid hemorrhage or damage to external blood vessels such as the vertebral or anterior spinal arteries is seldom observed. The main finding may only be extravasation of erythrocytes into the spinal cord parenchyma, which may evolve, on rare occasion, into frank hemorrhage (hematomyelia). Histologically, recognition of the presence of structural damage to the tissue itself requires that the victim survive for at least several hours after the injury.
3 Primary Spinal Cord Lesions
In those instances in which the injury is not immediately lethal and in which the afflicted individual survives for more than a few hours, histological abnormalities are more readily observed. For purposes of this discussion it is convenient to separate lesions into two categories, namely, those that develop at the time of impact (primary lesions) and those that develop afterward (secondary lesions).
For primary lesions, some investigators use the same terms as those used for traumatic brain injuries, i.e., concussion, contusion, laceration, and compression (8). Not all of these terms are in common usage, however, because of differences among investigators in the way they are defined. The word concussion, for example, is applied by some to transitory spinal cord dysfunction in the absence of structural damage and by others to injuries in which damage may be moderately severe (8, 9). Given the limited opportunity to examine human material in such circumstances, it would probably wiser to restrict its usage to those clinical situations in which, following traumatically induced spinal cord dysfunction, there is rapid and complete neurological recovery (10).
The word contusion, by analogy with its use in association with craniocerebral injuries, implies that the spinal cord has impacted against the bony wall of the spinal canal, a situation that seldom if ever prevails in actuality. Injury following upon contact between the spinal cord and its bony encasement is virtually always due to bony displacement (e.g., fracture or dislocation), in which case the term compression or crush injury is more appropriate.
Spinal cord laceration is seldom observed in the absence of a penetrating injury and, when it occurs, is most often the result of bone fragments being driven into the spinal cord following a vertebral fracture.
Spinal cord compression is generally due to fracture/dislocation or subluxation within the bony vertebral column. Vertebral body continuity may be restored completely immediately following the injury. When acute it may lead to the development of a cylindrical core of necrosis within the ventralmost portion of the posterior column that tapers, in conical fashion, above and below the level at which compression has been maximal (9, 11–13), much in the manner in which a tube of toothpaste has been squeezed (14) (Fig. 5). This type of lesion has sometimes been referred to as “pencil necrosis” (15, 16). With very severe injuries the spinal cord may be transected or completely crushed, although this is rare. Usually, some residual parenchyma can be observed traversing the site of maximal injury (3, 17).
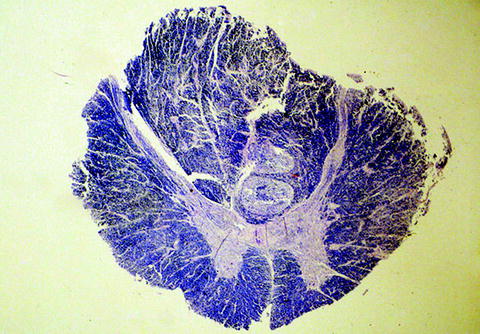
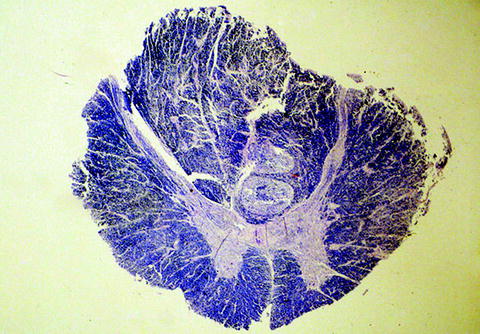
Fig. 5.
Acute spinal cord compression. Note the “cores” of displaced tissue within the ventral portions of the posterior columns (Klüver–Barrera stain).
4 Secondary Spinal Cord Lesions
Provided that the spinal cord is not transected or completely crushed, the earliest change that is visible by light microscopy is the presence of pericapillary extravasation of erythrocytes and serum constituents (6). These hemorrhages may become more numerous over the next few hours, but frank hematomyelia is uncommon. Swollen, injured axons may become visible as early as 30 min after the injury, as evidenced by immunoreactivity for β-amyloid precursor protein, owing either to axonal retraction bulb formation after transaction or to impairment of axoplasmic flow through otherwise anatomically intact axons (18, 19). Between 8 and 24 h post-injury small numbers of neutrophils begin to appear and the white matter becomes progressively more edematous, as evidenced by pallor of myelin staining. Macrophages appear at 24–48 h and steadily increase in numbers thereafter, and GFAP-positive reactive astrocytes appear at 48–72 h. At 24–48 h there is extensive coagulative necrosis with prominent neuronal ischemic cell change. Following this there is tissue breakdown progressing to cavitation. Neovascularization begins at approximately 1 week. In both human and experimental material there is evidence to suggest that, in the brain (and, presumably, also in the spinal cord), there is prolonged activation of resident microglial cells that may persist for months to years after injury (20, 21).
Although experimental studies have demonstrated a number of physiological and biochemical abnormalities, including impairment of microcirculatory perfusion (22, 23) and of autoregulation of blood flow (24), release of vasoactive amines and of excitotoxic neurotransmitters (25–27), Ca++ and K+ ion shifts (28, 29), and generation of oxygen-free radicals (30, 31), such abnormalities have not, for obvious reasons, been documented in human spinal cord-injured subjects.
Traumatic demyelination, i.e., loss of myelin with relative axonal sparing, though well studied in experimental models of spinal cord injury (3–34), does not appear to be as prominent in human material (3, 18). When it occurs, it typically does so in close relation to the site of primary impact, and then only around isolated axons. Evidence of oligodendroglial apoptosis has been described in human material (35). Remyelination of central axons, when observed, appears to be achieved by Schwann cells rather than by oligodendrocytes, a process sometimes referred to as “schwannosis” (3, 18, 36).
Collagenous scar formation occurs whenever the pia-arachnoid is breached. If the damage to the spinal cord parenchyma is extensive, the collagen may encroach upon what remains of the parenchyma to such an extent that it appears to replace it completely (Fig. 6). The collagen is presumably elaborated by arachnoidal cells and is limited to the subarachnoid compartment, as evidenced by the presence of a layer of arachnoidal cells between the mass of collagen and the dura (37) (Fig. 7). A recent study in experimental animals suggests that pericytes may also contribute to the appearance of collagen (38). The presence of scar tissue tethers the spinal cord and renders it less mobile within the spinal canal, making it more vulnerable to complications such as posttraumatic syringomyelia (see below).
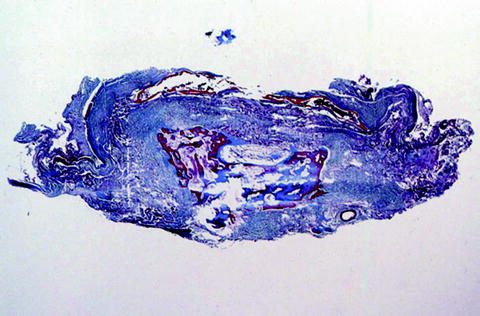
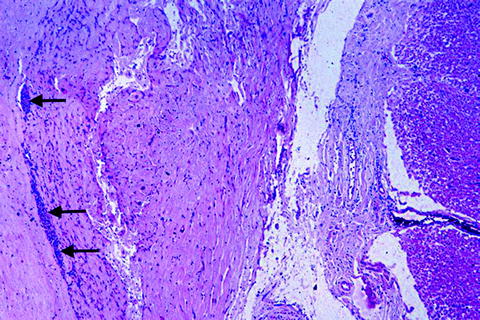
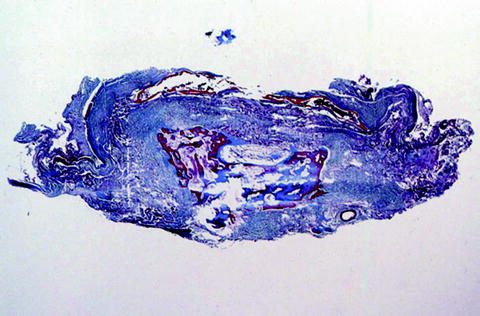
Fig. 6.
Spinal cord at T10 ten years after a fall, showing complete replacement of the spinal cord by collagenous connective tissue (blue). Heterotopic bone formation is present within the central portion of the scar (Masson trichrome stain).
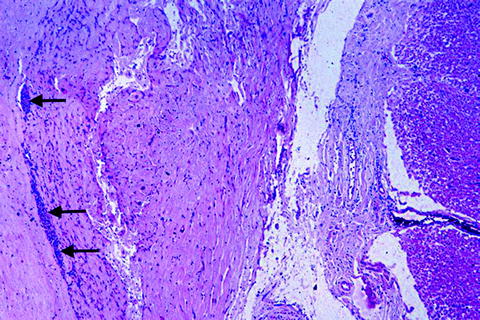
Fig. 7.
Spinal cord at C6 in a patient rendered quadriplegic 9 years previously in a motor vehicle accident. Dura is on the left and spinal cord on the right, with collagenous connective scar tissue in between. Note the layer of arachnoidal cells (arrows) between the scar tissue and the dura, thereby localizing the collagen within the subarachnoid compartment (hematoxylin and eosin stain).
Traumatic neuromata are present in great abundance where damage to spinal cord parenchyma is maximal (Fig. 8). These neuromata are presumed to represent regenerating sprouts, mainly from the centrally directed neurites of dorsal root ganglion cells (39–41).
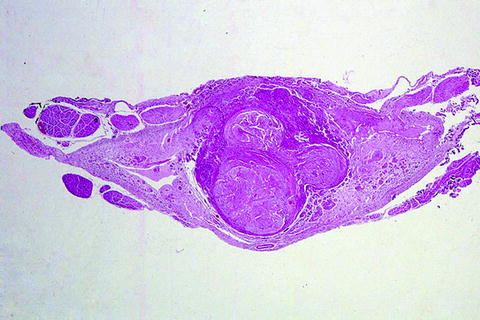
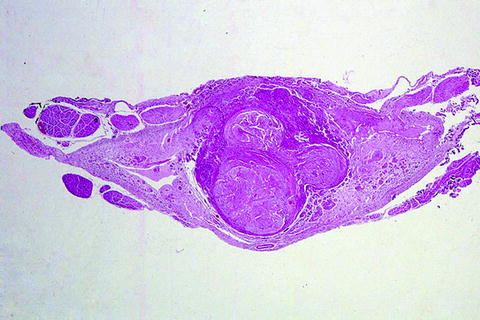
Fig. 8.
Spinal cord at C6 in a patient rendered quadriplegic 45 years earlier in a motor vehicle accident. Note the presence of numerous traumatic neuromas (hematoxylin and eosin stain).
Wallerian degeneration of ascending tracts above and descending tracts below the level of injury represents degeneration of those axonal segments distal to the site of transaction. The evolution of this process, which may take place over a period of years, is much more attenuated than it is in the peripheral nervous system. Marchi staining permits detection as early as 10 days after injury, but this is not commonly done nowadays. CD68-immunoreactive macrophages are seen 1–4 months after injury (42, 43) and are accompanied by reactive astrocytosis (44). Pallor of myelin staining of affected tracts may not become pronounced until 6 weeks to 2 months have elapsed.
Delayed posttraumatic syringomyelia is of singular clinical importance, as it may increase significantly the extent of neurologic impairment. The condition is characterized by the development, after an interval of several years, of one or more syringomyelic cavities extending either upward or downward for variable distances from the original site of injury (45) (Fig. 9). The consequences can be dire. If, for example, the cavity extends upward from a thoracolumbar lesion, a paraplegic patient may be rendered quadriplegic. The frequency with which this complication develops is not known but is probably over 20% (46). The syringomyelic cavity, which contains no lining epithelium and which is bordered by a zone of isomorphic gliosis (Fig. 10), has no direct communication with the central canal, fourth ventricle, subarachnoid compartment, or, necessarily, cavities formed at the original site of injury. The mechanism by which posttraumatic syringomyelia develops is not known, but the view has been expressed that progressive tearing may follow upon the effects of episodic elevation of venous back-pressure (such as that induced by coughing, sneezing, or Valsalva maneuvers) upon a spinal cord that is tethered by adhesive arachnoidopathy (37, 45).
< div class='tao-gold-member'>
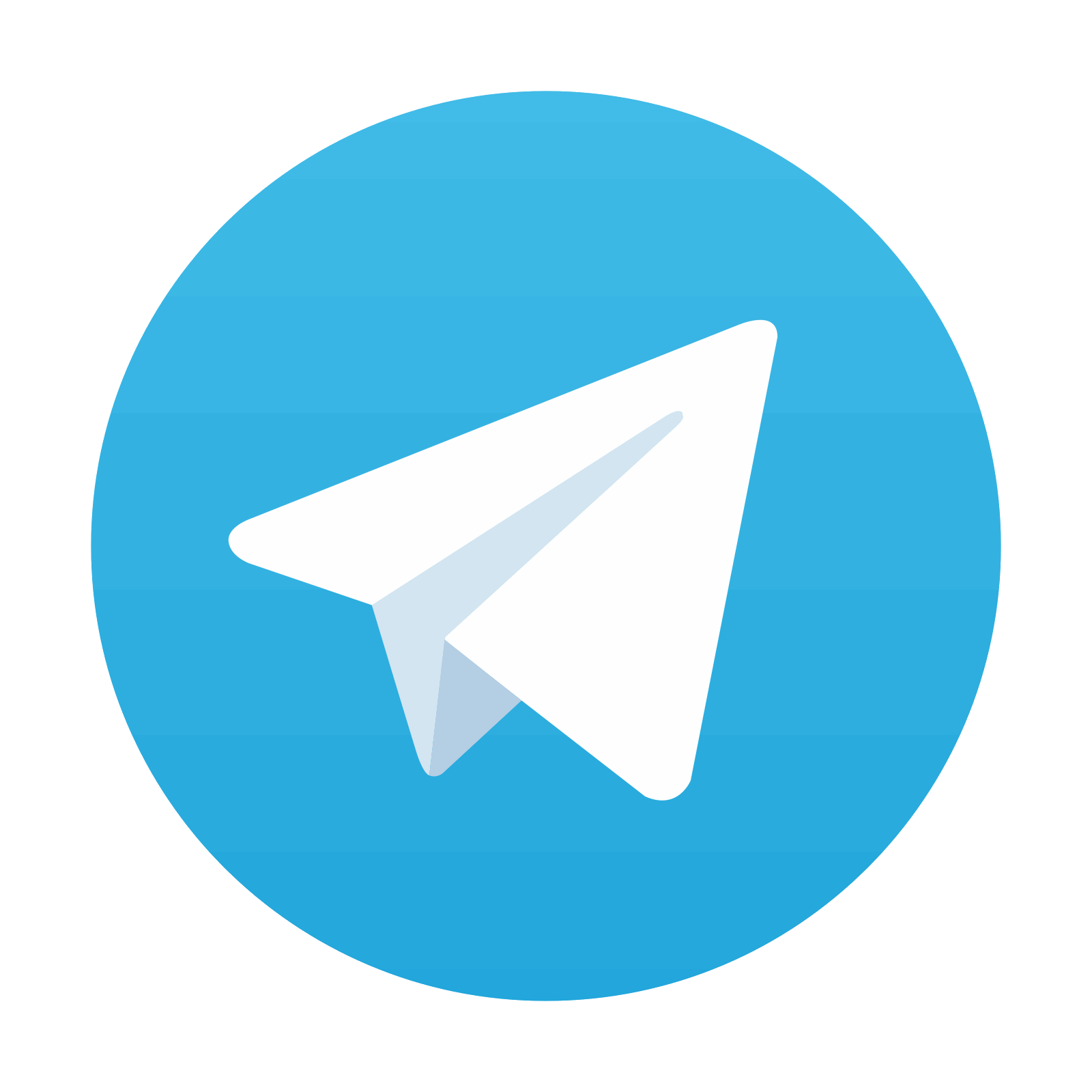
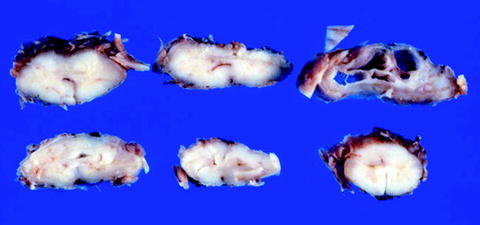
Only gold members can continue reading. Log In or Register a > to continue
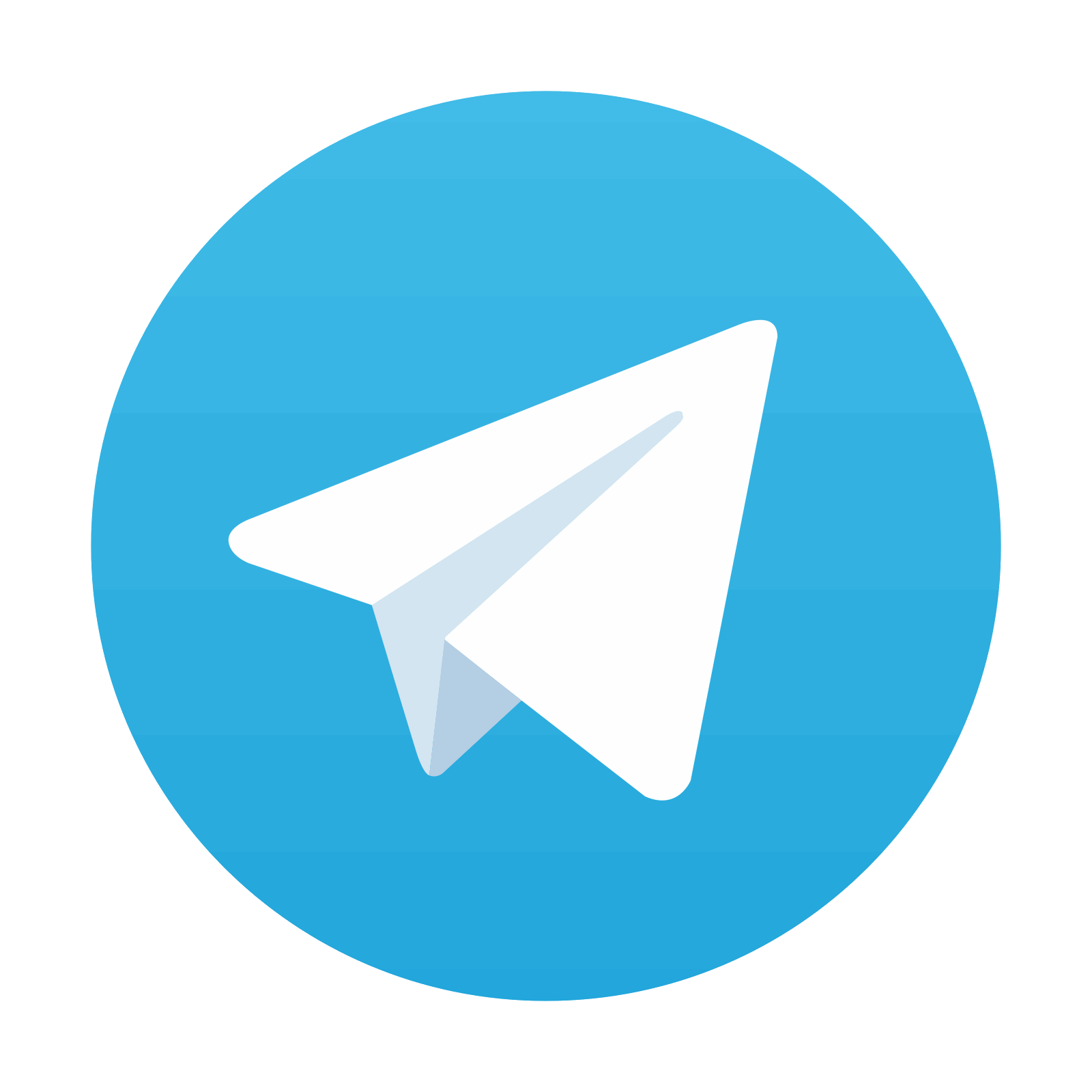
Stay updated, free articles. Join our Telegram channel

Full access? Get Clinical Tree
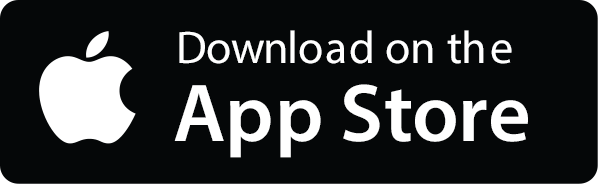
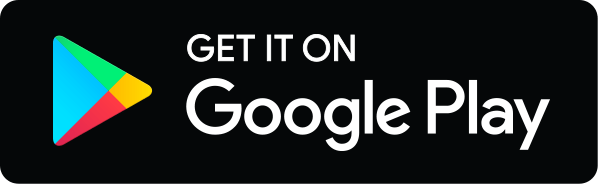