Chapter 24 The Mechanics of Aquarium Water Conditioning
The artificial filtration methods used in zoos and aquariums are similar to the ones used in swimming pool, municipal waste water, and drinking water treatment. Better understanding and consideration of natural stabilizing processes may provide more efficient and cost-effective life support for aquatic animals. First, understanding aquatic animal housing water flow systems is critical. Open systems have direct circulation with a natural body of water and do not usually require filtration. Semiclosed systems have basic filtration systems but rely on partial exchanges with an external water body. The third and most complex type of system is the closed system. Because this includes all types of filtration, this chapter will concentrate on this system. Basic marine mammal or bird pools are the simplest closed systems. These afford much more freedom from a water conditioning standpoint because the animals are not dependent on ultraclean water for respiratory needs, as in fish systems (Fig. 24-1). The most complex is the mixed exhibit, in which the fastidious needs of fish and the federal regulations for marine mammals must be addressed. To complicate issues, many exhibits now have people swimming in these systems and thus must meet public health regulations.
Once fish are added to systems, general oxidant sterilization cannot be used. These systems operate without chlorine and bromine and residual ozone oxidants should maintain an oxidation-reduction potential (ORP) below 350 mV. Systems in which water exchanges are nonexistent or limited need to consider denitrification systems to convert nitrates to nitrogen gas, which is off-gassed. With fish exhibits or mixed taxa exhibits, gas exchange partial pressures need to be considered. Once invertebrates are added, detailed chemistry control is needed but is beyond the scope of this chapter. It is important to recognize that conflicting information is based on varying philosophies between older traditional thoughts verses new ideas of ecologic approaches to water conditioning. For further reading, see studies by Carlson,3 Overby,5 Spotte,6 Van der Toorn,7 and Watson and Hill,8 and the website of the Aquatic Animal Life Support Operators (http://aalso.org).
Filtration Methods
Biologic Filtration
This makes biologic filters very sensitive and fickle to environmental changes. Filter bacteria are simple cells that are not capable of carrying out the complex internal digestive and excretory processes of higher organisms. In the presence of much accumulated organic matter, the bacterial environment becomes acidic, high in CO2, methane, and ammonia and low in dissolved oxygen (DO), with many bacterial enzymes for organic breakdown. Bacteria use complex organic foods, but have simple nutritional needs (e.g., simple sugars; small amounts of vitamins, amino acids, and micronutrients). Only a small portion of the nitrogen, phosphorus, and sulfur in these foods is used. (The majority of) these become organic wastes and tend to accumulate in the aquarium and become toxic or stressful to other aquatic organisms.1
The whole organic cycle is usually represented to the veterinarian in only partial form. It consists of the aerobic conversion of ammonia to nitrate and usually does not consider other organic processes, such as denitrification, which is usually incorporated in plants in natural systems. Organic biodegradation is an aerobic process in which reactions catalyzed by heterotrophic bacteria convert organic waste to inorganic chemical byproducts and reduced organics. Nitrification is an aerobic two-step process in which catalyzed reactions by highly specific genera of nitrifying bacteria convert NH4+ to NO3−2. Denitrification is an anaerobic two-step process whereby reactions catalyzed by specific bacteria reduce NO3−2 and release N2 as gas from the system. For further details of the denitrification process, see Chapter 25.
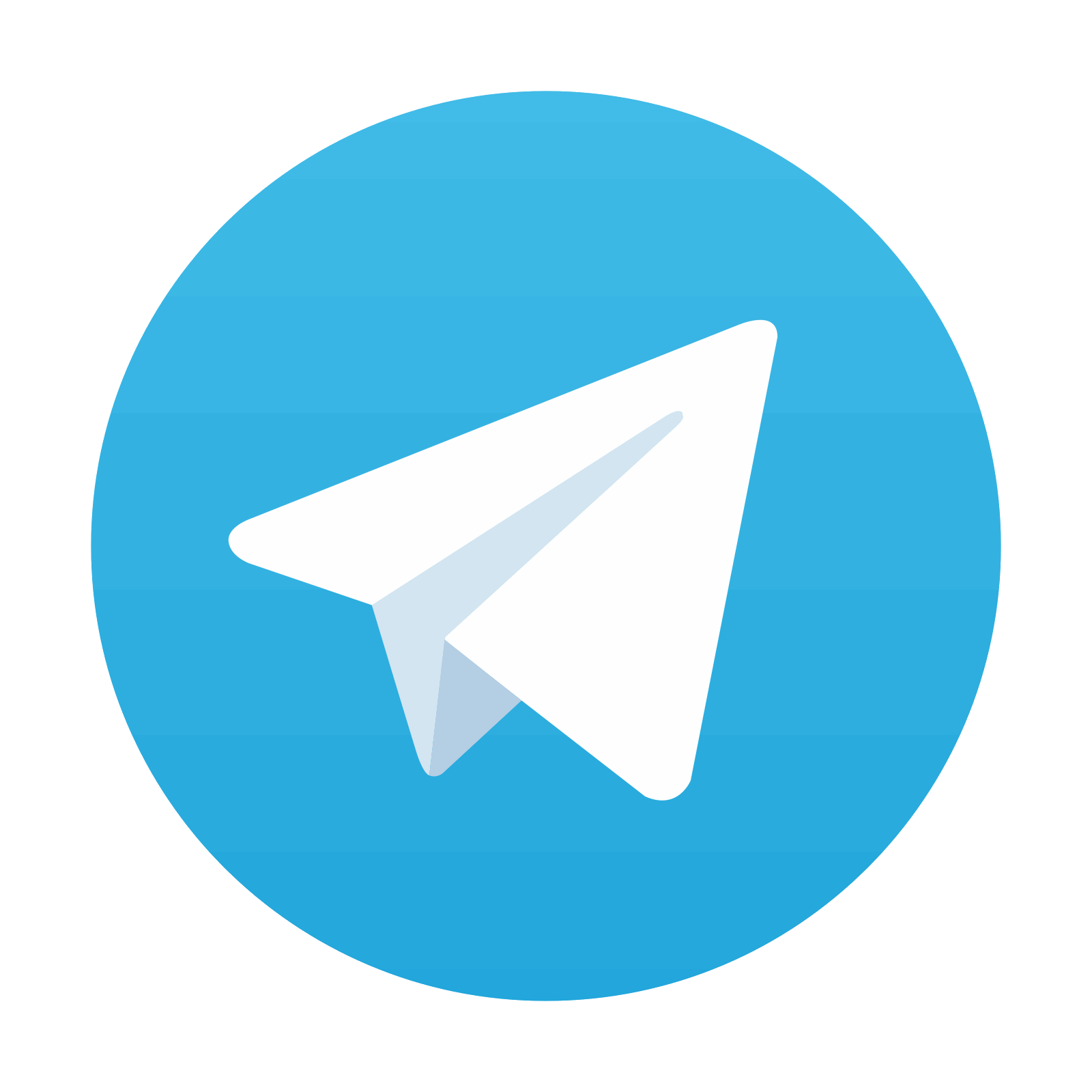
Stay updated, free articles. Join our Telegram channel

Full access? Get Clinical Tree
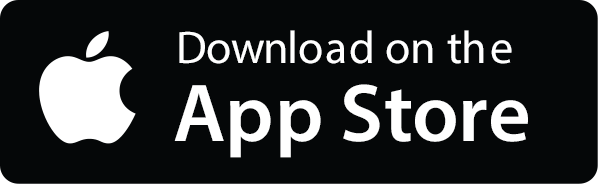
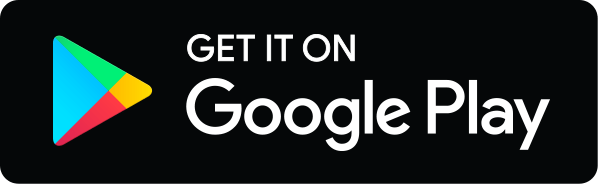