Chapter 1.2
The genomics revolution: will canine atopic dermatitis be predictable and preventable?
Background – Heritability studies suggest that atopic dermatitis (AD) involves multiple genes and interactions with environmental factors. Advances in genomics have given us powerful techniques to study the genetics of AD.
Objective – To review the application of these techniques to canine AD.
Results – Candidate genes can be studied using quantitative PCR and genomic techniques, but these are hypothesis-dependent techniques and may miss novel genes. Hypothesis-free techniques avoid this limitation. Microarrays quantify expression of large numbers of genes, although false-positive associations are common. In the future, expression profiling could be used to produce a complete tissue transcriptome. Genome-wide linkage studies can detect AD-associated loci if enough affected dogs and unaffected relatives are recruited. Genome-wide association studies can be used to discover AD-associated single nucleotide polymorphisms without relying on related dogs. Genomic studies in dogs have implicated numerous genes in the pathogenesis of AD, including those involved in innate and adaptive immunity, inflammation, cell cycle, apoptosis, skin barrier formation and transcription regulation. These findings, however, have been inconsistent, and problems include low case numbers, inappropriate controls, inconsistent diagnosis, incomplete genome coverage, low-penetrance mutations and environmental factors.
Conclusions – Canine AD has a complex genotype that varies between breeds and gene pools. Breeding programmes to eliminate AD are therefore unlikely to succeed, but this complexity could explain variations in clinical phenotype and response to treatment. Genotyping of affected dogs will identify novel target molecules and enable better targeting of treatment and management options. However, we must avoid misuse of genomic data.
Introduction
Human and canine AD
Canine atopic dermatitis (AD) is very similar to human AD;1–4 both are inflammatory dermatoses with characteristic clinical features. They are complex diseases involving immune dysregulation, allergic sensitization, skin barrier defects, microbial colonization and environmental factors. Human and canine AD are very common conditions, affecting up to one-third of children in Western societies and 10% of all dogs.1,5–8
It’s in the genes
Both the human and canine conditions have a genetic component. Family history is a major risk factor for human AD.1–3 Strong breed predispositions, with high prevalences in some dog breeds (e.g. up to 25% in West Highland white terrier dogs), suggest that this is also true in canine AD.9–11 In British guide dogs (mostly Labrador and golden retriever cross-bred dogs) the mean heritability is 0.47 (range 0.13–0.81), suggesting that the genetic background accounts for almost 50% of the risk of developing AD.12 Experimental laboratory colonies of dogs with conditions that mimic clinical AD have also been established.4,13–15
It’s not all genetic – environmental factors in AD
The risk of developing AD, the severity and the response to treatment are highly variable. This may be explained by complex genotypes, but it is also likely that environmental influences are important.8 Environmental factors that influence the development of canine AD are listed in Table 1.10,16,17 These may be important in immunity, tolerance and skin barrier function. The environmental influence, however, appears to vary with breed; for example, these factors do not affect the prevalence of AD in West Highland white terrier dogs.
Table 1. Environmental factors that influence the development of canine atopic dermatitis
Risk of developing atopic dermatitis | Environmental factor |
Increased | Urban life |
High human population density | |
Increased average annual rainfall | |
Living in southern Sweden | |
Adoption at the age of 8–12 weeks | |
Regular bathing | |
Reduced | Rurallife |
Living with other animals | |
Walking in forests | |
Feeding noncommercialfoods to lactating bitches | |
No effect | Sex |
Season of birth | |
Home environment | |
Vaccination | |
Deworming |
Genetic studies in AD
Historically, studies have been limited to observations of breed predispositions, as well as heritability and linkage studies. Advances in genomics now allow us to study the genetics of AD in more detail. The aim of this paper is to review these techniques, the current evidence for the genetic basis of canine AD and future opportunities.
Investigating AD-associated genotypes
Genome-wide linkage studies
Genome-wide linkage studies have been widely used to investigate human AD.8 These are family-based approaches using affected individuals, their parents and nonaffected family members. The inheritance of the disease is compared with the inheritance of microsatellite markers.
This technique can evaluate the whole genome, avoiding the limitations of candidate gene approaches. Microsatellite markers have been used to identify chromosomal loci associated with human AD.8 However, the usefulness of this approach has been questioned, because there has been little overlap in the results from these studies. The linked loci extend over large areas of each chromosome, spanning several genes and making it difficult to identify a candidate gene without either extensive chromosomal sequencing or further genotyping of additional microsatellite markers clustered around the loci of interest. It is difficult, furthermore, to amass enough affected individuals and unaffected relatives to perform genome-wide linkage studies in dogs.
Candidate gene association studies
It is possible to genotype markers specifically associated with candidate genes of interest. This allows the use of relatively simple markers, such as single nucleotide polymorphisms (SNPs), insertions, deletions and repeats. In addition, candidate gene approaches are not limited to families, making it easier to recruit large numbers of affected individuals and control subjects.8 However, case-control studies can be confounded by population stratification effects, such as ethnicity or breed and geography. A major disadvantage of this approach is that it is hypothesis dependent in that the genetic analysis is restricted to genes that have been previously implicated in the pathogenesis of AD. Novel genes may therefore be missed.
Genome-wide association studies
Genome-wide association studies (GWASs) are a hypothesis-free way to discover disease-associated SNPs.8 This avoids the limitations of using candidate genes, while retaining the advantages of the case-control approach. Genome sequencing has identified large numbers of genetic variants that can be read on SNP arrays. This allows identification of SNPs that are more frequent in affected individuals than control subjects. These disease-associated SNPs mark regions of the genome that may be involved in the pathogenesis of AD (Figure 1). Depending on the number and distribution of SNPs, GWASs can interrogate the entire genome. However, fine mapping relies on having many SNPs evenly distributed throughout the genome. In addition, disease-associated SNPs may be located in unknown areas of the genome. Further sequencing and functional studies are therefore required to confirm whether the disease association is causal. The functional effects, moreover, may depend on the specific combinations of SNPs within a gene and/or interactions with SNPs in other genes, or both. Another weakness of this approach is that GWASs are limited to identifying common SNPs with small effects. They cannot identify rare SNPs with large effects, untyped SNPs and some structural variations, e.g. microsatellites, variable number tandem repeats, insertions, deletions and duplications.
Figure 1. Whole-genome association plot of significance for canine atopic dermatitis (AD) (after Wood et al. with permission of Springer Science+Business Media).22 This study used a chip with approximately 22,000 single nucleotide polymorphisms (SNPs) to study SNP frequency in atopic dogs and healthy control dogs. The SNPs are plotted on the x-axis according to their position on each chromosome, with their association with AD on the y-axis shown as log10 P-value. The circles represent individual SNPs, which are grouped by chromosome. The SNPs that are statistically more frequent in the atopic population identify potentially AD-associated genes or loci for further studies.

Genome-wide association studies in dogs can take advantage of their strong linkage disequilibrium (LD). In humans, LD is relatively weak (extending over about 10–100 kbp), necessitating high-density arrays (up to 1.6 × 106 SNPs) and large cohorts (at least 1000 cases and controls).18 Dog breeds are of recent origin and are highly inbred, with LD over long distances (0.8–5 Mbp),19,20 meaning that fewer genetic markers and smaller sample sizes can be used. For example, complete coverage of the canine genome requires only 5000 to 30,000 SNPs.21 However, low numbers of SNPs can result in incomplete coverage that could miss important genes. For example, the first GWAS in canine AD22 used the Illumina Canine SNP20 chip (San Diego, CA, USA). This includes 22,362 canine SNPs from the CanFam2.0 assembly based on the boxer dog, with the partial sequence of a standard poodle dog and 100,000 sequence reads from nine other breeds. Despite this, many genes of interest (e.g. filaggrin) were not included. These gaps should be covered by continued development of the Dog Genome Project.
Quantitative RT-PCR
Quantification of mRNA can be performed in any tissue to identify genes that are differentially regulated in affected individuals compared with control subjects. Microarrays can be used for hypothesis-free assessment of very large numbers of genes on single chips.23 Gene transcription, however, does not necessarily imply causality; the change may be secondary to the disease process. Despite this, gene expression studies are useful to identify candidate genes and to confirm involvement of genes associated with AD in genomic studies. Genome-wide microarrays can produce a hypothesis-free transcriptome of all known genes in any tissue, but the high cost and complex results are barriers to widespread use at present.8
Bias and false results
Genomic studies are prone to bias and error that reduce the power of the analyses and affect replication of the results. Accurate phenotyping is critical, because any variation will have a profound impact on determining associations with AD. Other issues include failing to account for population stratification in case-control studies. Studies with relatively low numbers of cases are vulnerable to type II (i.e. false-negative) errors. Type I (i.e. false-positive) errors can occur following multiple testing unless corrections are used to reduce the false discovery rate. In addition, the effects of nonrandom mating, mutations, selection, small population effects, genetic drift etc. mean that canine populations may not be in be Hardy-Weinberg equilibrium (i.e. allele and genotype frequencies in a population remain in equilibrium).
Genomic studies in canine AD
Atopic dermatitis is a complex disease
Observations of atopic West Highland white terriers indicated that the inheritance patterns were consistent with a common fully penetrant dominant or recessive major locus.24–26 However, studies in other breeds suggest that canine AD is a multifactorial and polygenic condition with a complex mode of inheritance.4,12,15,27 Genomic studies in dogs have now implicated numerous genes in the pathogenesis of canine AD (Table 2), although whether these are associated with cause or effect is not always clear. These include genes involved in innate and adaptive immunity, inflammation, cell cycle, apoptosis, skin barrier formation and transcription regulation, many of which have also been implicated in human AD.
Table 2. Genes that have been implicated in canine atopic dermatitis by candidate gene quantitative PCR (qPCR), microarray, genome-wide linkage studies (GWLS), candidate gene single nucleotide polymorphism (SNP) studies or genome-wide association studies (GWAS)
Gene | Study | Potential relevance to canine atopic dermatitis |
Inflammation or immunology | ||
S100A8 | Microarray23 GWLS26 qPCR35 | Pro-inflammatory; correlated with clinical severity |
INPPL1 | Microarray23 qPCR35 SNP38 | Affects allergen-specific IgE responses, allergen presentation and uptake, T-cell activation and inflammatory responses |
SCCA-2 | Microarray23 | Serine protease inhibitor; marker for squamous cell carcinoma |
SAA | Microarray23 | Expressed in response to inflammatory stimuli |
SAA3 | Microarray23 | |
Serum amyloid A protein (Canis familiaris) | Microarray23 | |
TIMP1 | Microarray23 qPCR35 | Tissue inhibitor of metalloproteinase 1; promotes cell proliferation; anti-apoptosis |
ARTS-1 | Microarray23 | Cytokine receptor regulation; antigen presentation |
C10orf118 | Microarray23 | Unknown |
IL1RAPL1 | Microarray23 | Synthesis of pro-inflammatory proteins |
DPP4 | qPCR35 SNP38 | Regulation of inflammation; interacts with tumour necrosis factor-α and tissue inhibitor of metalloproteases 1 (TIMP1) |
PKP2 | qPCR35 | Upregulates T-cell activity and survival |
TNF | qPCR35 | Pro-inflammatory; tumour necrosis factor-α correlates with clinical severity |
PTPN22 | GWAS25 | Regulation of B- and T-cell receptor signalling; regulation of innate immune response; regulation of natural killer cell proliferation; associated with immune-mediated disorders |
TSLP-receptor | SNP38 | Cytokine gene transcription; implicated in allergic inflammation |
MS4A2 | SNP38 | High-affinity IgE receptor β subunit |
CD83 | GWAS40 | Antigen presentation and humoral immunity |
CMA1 | GWAS22 | Mast cell chymase |
NOD1 | GWAS22 | Intracellular microbial pattern recognition receptor; innate and adaptive immunity |
SELP (P-selectin) | GWAS22 | Leukocyte recruitment |
Cell cycle, apoptosis, repair or lesion formation | ||
RAD50 homologue isoform 1 | Microarray23 | Unknown |
POSTN | Microarray23 | Epithelial cell adhesion and migration |
POSTN | qPCR35 | |
CIDE-3 | Microarray23 | Apoptosis and cell proliferation |
SDC1 (syndecan 1) | Microarray23 | Integral membrane protein; involved in cell binding, cell signalling, cytoskeleton organization, cell proliferation, cell migration and cell-matrix interaction |
Cadherin-13 | Microarray23 | Cell signalling; cell migration and differentiation |
Cullin 4A | Microarray23 | Cell cycle regulation and development; DNA repair |
qPCR35 | ||
DCLRE1B | GWAS25 | Unknown |
BCL2L15 (BCL2-like 15) | GWAS25 | Regulation of apoptosis and tolerance |
ANGPTL4 | GWAS22 | Skin wound healing; keratinocyte migration, endothelial apoptosis survival factor |
Transport or regulation | ||
Kinectin 1 | Microarray23 | Unknown |
Myosin Va | Microarray23 | Unknown |
A-kinase anchor protein 9 isoform 2 | Microarray23 | Unknown |
Sperm-associated antigen 5 | Microarray23 | Unknown |
Canis familiaris ret proto-oncogene | Microarray23 | Unknown |
Nucleoprotein TPR | Microarray23 | Unknown |
Phospholipase C, zeta 1 | Microarray23 | Unknown |
Potassium channel tetramerization | Microarray23 | Unknown |
FERM, RhoGEF, pleckstrin domain protein 2 | Microarray23 | Unknown |
ATP-binding cassette C12e | Microarray23 | Unknown |
SFXN5 sideroflexin 5 | GWAS25 | Unknown |
EXOC6B exocyst complex component 6B | GWAS25 | Exocytosis |
AP4B1 | GWAS25 | Endocytosis and secretion |
ABCC3 | GWAS22 | Protein transport; drug efflux |
Barrier formation | ||
GOLGA4 subfamily a5 GOLGA4 subfamily a4 | Microarray23 | Golgi body function; glycosylation and transport of proteins and lipids |
SPINK5 | qPCR35 | Regulates proteolysis and keratinocyte differentiation; filaggrin function; T helper-2 polarization |
SGPL1 | qPCR35 | Skin barrier regulation; antimicrobial activity; keratinocyte proliferation and differentiation |
Mucin-15 | Microarray23 | Cell adhesion to matrix |
Mucin-2 | Microarray23 | Mucosal mucin protection |
Tight junction protein 3 | Microarray23 | Signal transduction; cell adhesion |
PPAR gamma | qPCR35 | Reduced lamellar body formation and lipid processing; anti-inflammatory |
FLG (Filaggrin) | SNP38 | Alterations in filaggrin expression and function associated with human and canine atopic dermatitis |
RAB3C | GWAS22 | Implicated in epidermal lipid layer and skin barrier formation |
Transcription and translation regulation | ||
eIF-5B | Microarray23 | Unknown |
STAT2 | Microarray23 | Cell growth, survival and differentiation; inflammation and immunity; interferon-γ regulation; tolerance |
CGGBP1 (CGG repeat binding protein 1) | Microarray23 | Unknown |
FUSE binding protein 2 | Microarray23 | Unknown |
FOXO4 (foxhead box) | Microarray23 | Cell metabolism and proliferation; tolerance |
Empty spiracles homologue 1 | GWAS25 | Unknown |
ZNF638 zinc finger protein 638 | GWAS25 | Unknown |
PAIP2B | GWAS25 | Unknown |
PHTF1 | GWAS25 | Unknown |
HIPK1 | GWAS25 | Unknown |
TRIM33 | GWAS25 | Regulation of transforming growth factor-β receptor signalling |
Miscellaneous | ||
EEA1 | Microarray23 | Unknown |
CG15747-PA | Microarray23 | Unknown |
HIF1a (Hypoxia-induced gene 1a) | Microarray23 | Unknown |
C6orf142 | Microarray23 | Unknown |
Sushi-repeat-containing protein SRPX | Microarray23 | Antioxidant; cytokine regulation |
RING-H2 protein | Microarray23 | Unknown |
ATRX1 | Microarray23 | Unknown |
C1orf163 | Microarray23 | Unknown |
FBXL10 | Microarray23 | Unknown |
FOLH1 | Microarray23 | Unknown |
S6K-alpha 6 | Microarray23 | Serine kinase; cell growth and differentiation |
mSin3A-associated protein 130 | Microarray23 | Unknown |
PH domain leucine-rich repeat protein phosphatase-like | Microarray23 | Inhibitor of kinases and growth factor-induced signalling |
Spag6 | Microarray23 | Unknown |
Ecotropic viral integration site 1 | Microarray23 | Unknown |
SPR sepiapterin reductase | GWAS25 | Unknown |
Cytochrome P450 26B1 | GWAS25 | Lipid metabolism and barrier function; cytochrome P450 activity |
DYSF dysferlin | GWAS25 | Unknown |
NAGK N-acetylglucosamine kinase | GWAS25 | Unknown |
RSBN1L | GWAS25 | Unknown |
OLFML3 | GWAS25 | Unknown |
SYT6 synaptotagmin VI | GWAS25 | Endocytosis; cell adhesion |
PROM1 | GWAS22 | Unknown |
RAB7A | GWAS22 | Melanocyte function and melanogenesis |
SORCS2 | GWAS22 | Neuropeptide receptor activity |
The bold and italic refers to genes that were differentially expressed in atopic skin compared with healthy control dogs; bold, upregulated and italic, downregulated.
Microarray studies
An initial paper reported that 54 of 22,000 genes (Agilent 22K oligonucleotide canine array; Agilent Technologies, Palo Alto, CA, USA) showed significantly different transcription in canine AD compared with control dogs (Table 2).23 The genes were broadly grouped into inflammation/immunity, cell cycle/apoptosis/repair, barrier formation, transport/regulation and transcription pathways. Most of the inflammation or immunology markers were upregulated in atopic, particularly lesional, skin. Other genes were generally underexpressed, especially in nonlesional atopic skin. A large number of these downregulated genes are involved in the transport of calcium, potassium and other ions which may affect expression and function of other mediators involved in inflammation and barrier function. The most dysregulated gene was S100 calcium binding protein A8 (S100A8). This is an important pro-inflammatory molecule located on the epidermal differentiation complex. Interestingly, a recent paper correlated S100A8 expression with the clinical severity of canine AD.28 Release is stimulated by tumour necrosis factor-α, the levels of which also correlate with clinical severity.29 The epidermal differentiation complex comprises genes for proteins such as profilaggrin, loricrin, involucrin and S100, which are essential for keratinocyte and epidermal barrier differentiation.30 These genes were not available on the canine microarray and GWAS chips used in earlier studies,22,23 and since these studies canine and human AD have been associated with loss-of-function filaggrin mutations and altered filaggrin expression.31–34
This microarray study2 provided a rapid, wide-ranging assessment of many genes and identified novel targets and pathways for further investigation; however, it was limited by the omission of key epidermal barrier and inflammatory cytokine genes. In addition, the study used a relative low number of dogs, which reduced its power to detect small changes. Using multiple breeds may have also confounded the results and reduced the power of the study.
Candidate gene studies
One study using atopic and healthy skin quantified mRNA for 20 genes identified from the microarray study23 and/or earlier literature.35 Significant differences were seen for 11 genes, involving immune responses, regulation and skin barrier function. Seven of these have been associated with human AD. S100A8 was again the most dysregulated gene. Three genes correlated with Canine Atopic Dermatitis Extent and Severity Index (CADESI)-03 scores (S100A8, SAA-1 and PKP2), and four genes correlated with intradermal test results (CMA1, SAA-1, SPINK5 and S100A8); these have been associated with inflammation, T-cell survival and skin barrier function.28,36,37 Weaknesses of this study included the relatively small sample size, use of multiple dog breeds and the variable time scale of the lesions at presentation.
Analysis of 97 SNPs in 25 candidate genes in 659 dogs of eight breeds from the UK, USA and Japan found that six were significantly associated with canine AD.38 A SNP in the thymic stromal lymphopoietin (TSLP)-receptor, which has been implicated in allergic inflammation,39 was seen in all eight breeds. Other canine AD-associated SNPs, however, were restricted to certain dog breeds and locations (e.g. filaggrin with UK Labrador retriever, and INPPL1 and MS4A2 with Japanese shiba inu).
A linkage study in West Highland white terrier dogs24 used specific microsatellite markers for fine mapping of the FLG locus. There were, however, no haplotypes that associated significantly with canine AD. This makes a primary role for filaggrin defects in AD in these dogs unlikely, although this has been implicated in other breeds.31–33
Genome-wide linkage studies
A genome-wide family-based linkage approach using microsatellites [256 markers from the Minimal Screening Set 2 (MSS-2) that covered the genome with an average intermarker distance of 8.59 cM] in 90 West Highland white terrier dogs from families with AD did not detect any chromosomal regions that were significantly linked to canine AD.26 However, some chromosomal regions were not covered at high density, reducing the power of this study. The highest linkage score was for a region on CFA7 that contains the S100A8 gene, which has been associated with canine AD.23,28,35 This study excluded linkage to the FLG locus, corroborating the results of other reports.24,38,40 This suggests that primary defects in filaggrin expression are not important in the pathogenesis of canine AD in West Highland white terrier dogs.
Genome-wide association studies
The first GWAS in canine AD22 evaluated DNA from 242 atopic and 417 control dogs of eight breeds from the UK, US and Japan. The top 40 SNPs were selected for validation (Figure 2). Nine chromosomes expressed multiple SNPs, suggesting a haplotype effect. Subsequent quantitative PCR with quality assurance testing and corrections for the false discovery rate and population structure revealed that 13 SNPs had a significant association with canine AD; however, a number were intergenic, with unknown functions and interactions.
Figure 2. Network pathway of genes associated with canine atopic dermatitis supported by at least one reference from the literature or from canonical information stored in the Ingenuity® Knowledge Base (IPA; Ingenuity Systems, http://www.ingenuity.com). Nodes are displayed using various shapes that represent the functional class of the gene product.

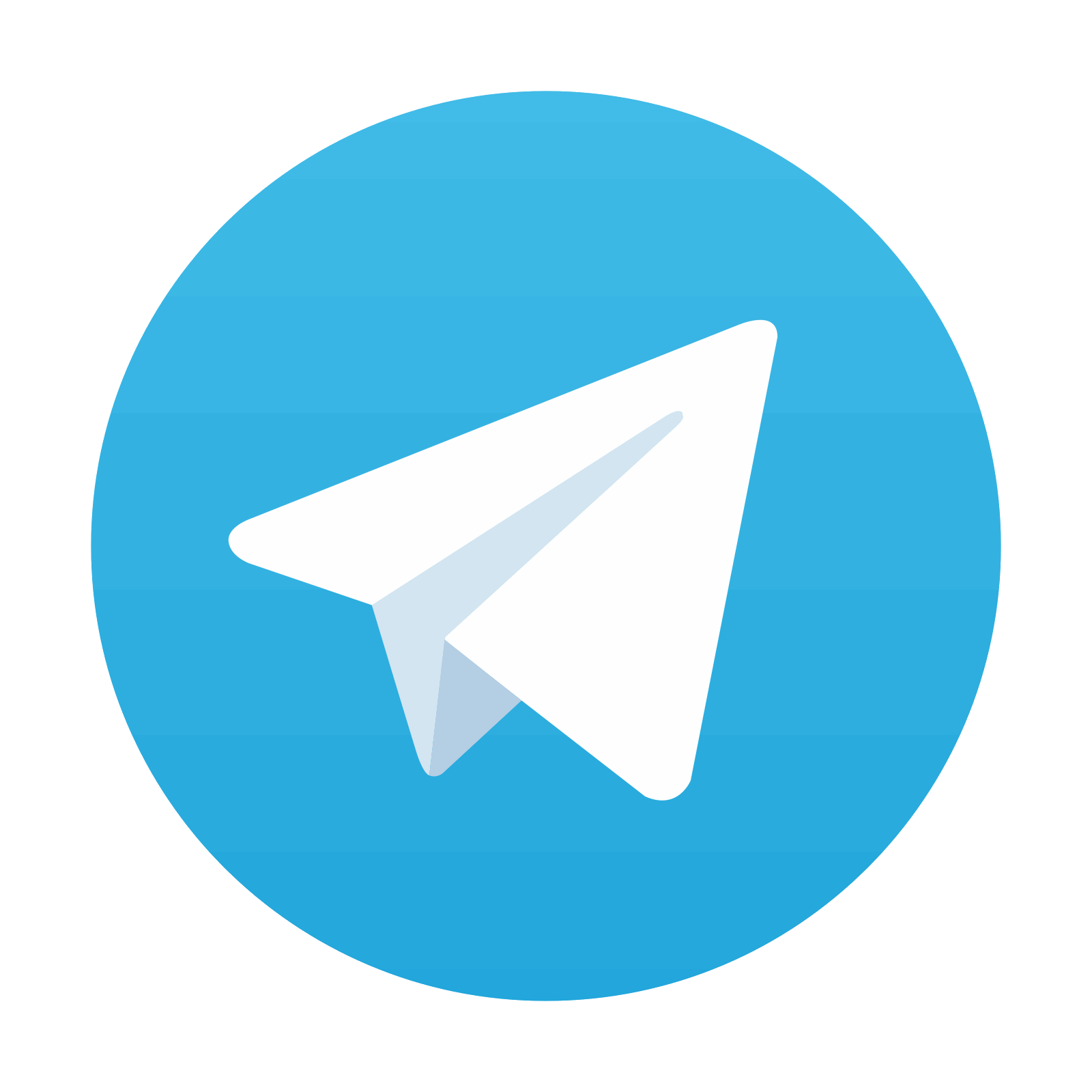
Stay updated, free articles. Join our Telegram channel

Full access? Get Clinical Tree
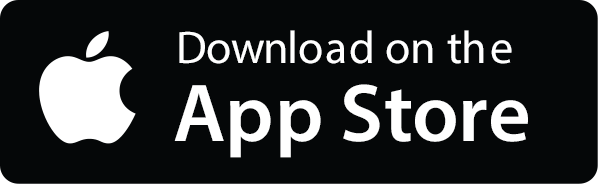
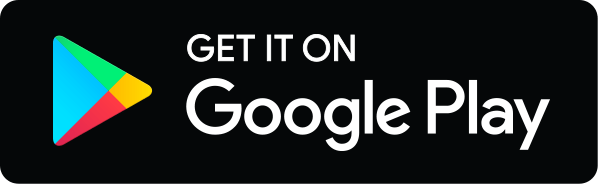