Chapter 5
Target Organ Pathology
Elizabeth McInnes
Cerberus Sciences, Thebarton, SA, Australia
In theory, all tissues and organs in the body are potential targets for the toxic effects of xenobiotics. In practice, however, certain organs are affected more than others. The skin, eyes, respiratory tract and gastrointestinal tract are often target organs as a result of exposure due to the route of administration (intradermal, inhalation, oral gavage, etc.). In addition, tissues associated with metabolism and excretion, such as the liver and kidney, often demonstrate test article-related findings. Generally, the responses to xenobiotic toxicity in all tissues follow the same form as those induced by other causes: degeneration, necrosis, acute and chronic inflammation, proliferation and neoplasia, as discussed in Chapter 3. The assessment of the toxicity of a xenobiotic is determined by establishing whether it elicits an effect in more than one animal species, whether there is a clear dose response between the low- and high-dose animal groups and, in the case of tumours, whether they are benign or malignant, or both. In this chapter, emphasis is placed on lesions that may be observed by study personnel at necropsy, rather than complex histopathological lesions only visible under the light microscope.
5.1 Skin
The skin is the external covering of the body. As one of the largest organs of the body, it is vulnerable and often injured. Changes to the skin and fur may be the most obvious changes seen in laboratory animals on study. The presence of abundant fur in most species (with the exception of the minipig), and the associated difference in skin physiology, means that any changes seen must be carefully evaluated for their potential relevance to humans. A fur covering may also mask changes to the underlying skin, which can only be visualised by microscopic examination. Changes in the skin may be seen both following systemic administration of drugs (although this is quite rare) and as a result of topical drug application (more common). In addition, the skin is capable of biotransformation and may produce toxic metabolites from a harmless compound (Oesch et al., 2007).
Fur/hair loss or thinning (Figure 5.1) may occur as a result of systemic administration of drugs, and reflects changes in hair growth or follicular destruction. Loss of hair (alopecia), reduced hair production (hypotrichosis) and excessive hair production (hypertrichosis) may all be observed after topical or systemic administration of xenobiotics. Alopecia is caused by a large number of drugs, including cytotoxic drugs and retinoids (Haschek et al., 2010). Topical xenobiotics may cause systemic effects, or a xenobiotic administered systemically may have effects on the skin (e.g. corticosteroids administered orally may cause skin thinning (atrophy) in dogs).

Figure 5.1 Fur/hair loss or thinning (alopecia) in a mouse.
Fur/hair colour may change with the administration of xenobiotics. Melanocytes (which are black) occur in the skin of pigmented animals. Oestrogens tend to cause an increase in skin pigmentation (i.e. a dark brown black colour) (hyperpigmentation), whilst androgens cause a decrease in pigmentation (hypopigmentation) (Tadokoro et al., 2003). Some nanoparticles can cross the skin and enter the lung and nerves, but nanocrystalline silver in wound dressings localises only in the superficial layers of the stratum corneum, causing grey discolouration (Samberg et al., 2010).
Skin reddening (erythema) and scaling may be most obvious where areas of skin have been exposed (e.g. via hair clipping) to allow topical administration of drugs. This represents the early inflammatory response to irritation (i.e. vasodilatation of blood vessels and skin thickening). It can be induced by the method used to remove the fur (e.g. shaving or chemical depilation), and it is important that this factor is controlled for by preparing sites in the same way and then applying vehicle at a control site. Vasodilation causing erythema and blister or vesicle formation (accumulation of oedematous fluid) may also be observed at necropsy, after exposure to irritant xenobiotics such as toluene. Fibrosis and scarring will result after initial erosion and ulceration of the skin.
Skin thickening (epidermal thickening is called ‘acanthosis’) (Figure 5.2) and scaling may be hard to appreciate if the fur is intact, but may be noted in life as an increase in loose scale (‘dandruff’). It is often associated with modification of sebaceous gland activity. Skin erosion (partial loss of the epidermis) and ulceration (complete loss of the epidermis) may be easily recognised as red craters lined by yellowish crusts visible in the overlying skin (Figure 5.3). Ulceration occurs as a result of direct necrosis of the skin tissue caused by corrosive agents or as a secondary effect of vasculitis, leading to local ischaemia of the skin. Compounds that cause ulceration include irritant substances such as acids and alkalis, as well as drugs that cause immune-mediated responses, vasculitis and/or photosensitivity reactions (including sulphonamides, penicillins and anticonvulsant drugs) (Haschek et al., 2010). Photosensitivity is noted in the skin when ultraviolet (UV) light activates photosensitive compounds (e.g. fluoroquinolones, tetracyclines and retinoids) (Ferguson, 2002) present either on its surface (topical administration) or within it (systemic administration), causing erythrema, blistering and eventual sloughing of the skin.

Figure 5.2 Acanthosis (epidermal thickening) in a young rat pup.

Figure 5.3 Necrosis, erosion and ulceration of the skin in the preputial gland area of a male mouse.
Pustule and abscess formation generally occurs as a result of inflammation of the hair follicle. Pustules are easy to recognise, generally consisting of a ‘pimple-like’ structure with a central mass of pus (yellow) surrounded by a layer of fibrosis or scar tissue. Inflammation of the hair follicle may cause the formation of pustules, and later micro- and macroabscesses. Acne can be caused by chlorinated hydrocarbons and is characterised by dilated sebaceous glands filled with keratin.
Petechiae are small (~1–2 mm diameter) red haemorrhages in the skin or subcutis (Figure 5.4). They are easy to see in nude mice. They may be caused by systemic compounds (e.g. sulphonamides), eliciting widespread vasculitis (inflammation of blood vessels) or disseminated intravascular coagulation (widespread microthrombi lodging in vessels, causing macroscopic petechiae) (Wojcinski et al., 2013).

Figure 5.4 Petechiae on the skin of a nude mice.
Granulomas are a distinct reaction in various tissues (e.g. lungs and skin), causing focal, raised masses. They typically contain a centre made up of foreign material or microorganisms, surrounded by large multinucleate macrophages, neutrophils and a layer of fibrosis. Lesions caused by drugs in the dermis include granulomatous inflammation due to modified hyaluronan (Westwood et al., 1995) or a foreign body, such as a medical device. Calcification or mineralisation of the subcutis may be observed on treatment with substances such as dihydrotachysterol (Wojcinski et al., 2013), causing the skin to become hard and gritty when cut.
Skin tumours may involve the epidermis (e.g. squamous cell papilloma, squamous cell carcinoma; Figure 5.5) or may be derived from mesenchymal cells (e.g. fibrosarcoma, lipoma, mast cell tumour, haemangiosarcoma). Tumours of the hair follicles include trichofolliculoma and trichoepithelioma. Melanocytic tumours (generally brown or black in colour) of the skin include benign and malignant melanoma; they may be induced by UV light (Ingram, 1998). Chronic administration of an irritant xenobiotic to the skin can cause the development of tumours, such as squamous cell carcinoma (generally, a large, ulcerated, raised skin mass that does not heal). Biomaterials such as microchips may also be carcinogenic, and may cause mesenchymal tumours in the skin due to chronic irritation (Elcock et al., 2001).

Figure 5.5 Squamous cell carcinoma in the dorsal skin above the scapula in a rat.
5.2 Eye
Injury to the eye occurs in all species of laboratory animals, and ranges from minimal (e.g. conjunctivitis (Figure 5.6), where only the skin round the eye is affected) to severe (i.e. panophthalmitis, where all are components are inflammed). Bulging eyes are easy to recognise in all laboratory animal species; this phenomenon is termed ‘exophthalmos’ and is generally secondary to inflammation of the orbit or the presence of tumours (e.g. a Harderian gland adenoma or adenoacarcinoma behind the eye). Excessive tear production (lacrimation) and brown discolouration (red tears) of the periorbital skin may be observed, particularly in rodents. Excessive lacrimation can be seen around the eyes and is caused by stress or cholinergic drugs (Harkness and Ridgeway, 1980).

Figure 5.6 Conjunctivitis in a mouse.
Dry eyes with a reduction in tear production leads to redness and scaling in the conjunctiva; this is known as ‘keratoconjunctivits sicca’. Radiation that causes degeneration of the lacrimal gland can lead to a reduction in tears and as well as local anaesthetics. Cloudiness of the cornea tends to indicate keratitis (inflammation of the cornea) and is caused by dust, irritant substances and topical administration of prostaglandin agonists (Aguirre et al., 2009). Initially, keratitis presents as a cloudiness to the cornea (oedema); later, ulceration can occur. An injured eye may heal with fibrosis (scarring), causing a white cloudiness of the cornea (pannus).
Inflammation of the skin around the eye is called ‘conjunctivitis’, and the periorbital skin will be red and swollen (oedema), and may be ulcerated and weeping. Causes include infectious agents and compounds such as ricin (Strocchi et al., 2005). The accumulation of blood in the anterior chamber is called ‘hyphema’, whilst the accumulation of pus in the anterior chamber is called ‘hypopyon’; this is seen in cases of bacterial infection (e.g. Staphylococcus aureus). Inflammation of the iris (iriditis) may resolve with fibrosis and can be caused by cyclophosphamide. Impaired drainage of the aqueous humour is referred to as ‘glaucoma’.
The lens of the eye is normally transparent. ‘Cataract’ is the degeneration of the lens, causing whitish opacification of the eye (Figure 5.7) and a white-appearing lens. Cataract may be caused by age, diabetes, UV light or compounds such as glucocorticoids. Retinal atrophy is common in older mice and may be caused by ageing or continuous lighting. It is not possible to see retinal atrophy without performing an ophthalmoscopic examination (fundoscopy) or looking at the retina under the light microscope.

Figure 5.7 Whitish opacification of the eye due to the presence of a cataract.
5.3 Gastrointestinal Tract
The gastrointestinal tract is one of the largest organs of the body. It is a prime target for test article-related lesions because it is the first organ in contact with the test substance following ingestion. Gastric lavage and oral ingestion are two of the most common routes of chemical administration, with a high likelihood of intestine-related toxicity. Clinical signs relating to the gastrointestinal tract are obvious and easily recognised in laboratory animals on study; these include diarrhoea, salivation, blood in the faeces and vomiting in some animals (but not rats or mice). The critical balance between constant cell division and cell loss in the stomach and small and large intestines means that ulceration and proliferation occur commonly. In general, the mechanism of injury in the gastrointestinal tract is irritation, and the response is degeneration/necrosis, inflammation and proliferation.
Thickened gums and tooth abnormalities may be observed in the mouths of laboratory animals on study. Calcium channel blockers such as cyclosporine A cause gingival hyperplasia (excessive thickening of gums) in dogs, whilst immunosuppressants such as corticosteroids may cause ulceration with subsequent Candida overgrowth due to the inability of the immune system to defend against the invasion of yeasts. Tetracyclines cause tooth discolouration, and dental abnormalities are noted with cyclophosphamide treatment. Vascular endothelial growth factor (VEGF) is an angiogenesis inhibitor and may cause dental dysplasia (disordered growth) and degeneration resulting in tooth fractures, which may be visible at necropsy as broken and extremely white teeth. Sunitinib, a broad-spectrum tyrosine kinase inhibitor, causes gingival necrosis in non-human primates (Patyna et al., 2008), which is visible as focal areas of ulceration on the gums. Tumours of the mouth include squamous papilloma (Figure 5.8) and squamous cell carcinoma of the oral mucosa. Tumours of the teeth include odontoma, fibroma and ameloblastoma; these may occasionally be induced by compounds such as 3-methylcholanthrene (Greene et al., 1960).

Figure 5.8 Squamous papilloma in the mouth of a rat.
Excessive salivation is characterised by increased amounts of saliva. It is easy to recognise in dogs and non-human primates, with strands of saliva extending from the mouth over the chin. Beta adrenergic agonists may cause salivary-gland enlargement (Ten Hagen et al., 2002), and excessive salivation is seen in organophosphate toxicity (Betton, 1998a). Salivary gland inflammation and atrophy due to reduced food consumption is a common test article-related finding. Tumour development in the salivary glands includes adenoma, adenocarcinoma, myoepithelioma and mesenchymal tumours and is rarely caused by administration of xenobiotics.
Difficulty in swallowing can be observed in rodents, dogs and non-human primates, and may be related to changes in the oesophagus. In these cases, undigested food will be present either in the mouth or on the floor in front of the affected animal. Lesions of the oesophagus include oesophagitis and gavage injury (where the gavage tube goes through the oesophageal tissue into the thoracic cavity and causes inflammation). Oesophagitis generally heals with scar formation and fibrosis, and obstruction may result (Betton, 1998a). Megaoesophagus (enlarged dilated oesophagus) causes difficulty in swallowing and is generally congenital. Zinc and vitamin A deficiencies can cause thickening of the oesophagus (hyperkeratosis), which is visible as a thickened white internal oesophageal lining. Oesophageal tumours include papilloma and carcinoma and may be induced by nitrosaniline and nitrosourea compounds in rats (Markovits et al., 2013).
Stomach ulceration and vomiting are often observed when the lining of the stomach is damaged. During life, the animal may show a hunched position, indicating abdominal pain. Stomach ulcerations can be easily recognised at necropsy, and consist of small reddened craters (full- or partial-thickness ulceration of the mucosa) present in the glandular and nonglandular stomach of rodents or in the glandular stomach of dogs. Ulcers in the stomach may lead to gastric haemorrhage, which is characterised by dark black gastric contents as the blood is digested by the gastric enzymes. Irritant compounds, such as ethanol, acids and alkalis (Betton, 1998a), cause initial erosion and ulceration, and later can also cause proliferative changes and thickening of the mucosa of the stomach. Mineralisation of the stomach is unlikely to be seen at necropsy, although it may manifest as a grittiness during cutting of the stomach with a knife or scalpel at necropsy. Mineralisation of the mucosa and muscle layers of the stomach may be observed with MEK inhibitors (Diaz et al., 2012), but this is a rare lesion.
Thickening of the stomach wall can be recognised at necropsy, especially when treated stomachs are compared to the stomachs of control animals. Hyperplasia of the glandular stomach (particularly the neuroendocrine cells of the stomach) progressing to neoplasia may be observed with antisecretory drug treatment, such as omeprazole (neuroendocrine cell proliferation), as well as with irradiation. Antisecretory drugs such as cimetidine restrict the production of hydrochloric acid in the stomach, stimulating the neuroendocrine cells to increase and enlarge. Stomach tumours are easy to recognise, generally consisting of large, raised, red, ulcerated masses. They may be induced by xenobiotics such as nitosamines. Tumours in the stomach include papilloma, squamous cell carcinoma, adenoma, adenocarcinoma, neuroendocrine tumours and mesenchymal tumours. Squamous cell carcinoma is commonly induced by irritants, promoters and genotoxins (Figure 5.9) (Chandra et al., 2010).

Figure 5.9 Squamous cell carcinoma in the stomach of a rodent.
Diarrhoea is common in laboratory animals, and pools of liquid (often mucoid or bloody faeces) are generally visible in the caging. At necropsy, the small and large intestines will be moderately dilated and filled with foul-smelling green, brown or red liquid contents. In severe cases, diarrhoea can result in the telescoping of the small intestine into a downstream section, causing a lesion known as ‘intussusception’; this may be caused by compounds such as alpha adrenergic agonists, which increase intestinal motility and thus cause diarrhoea. The enterocytes of the intestinal surface have a high mitotic rate and are thus very susceptible to cytotoxic drugs such as cyclosporine, as well as radiation. Irritants compounds (such as heavy metals) cause mucosal ulceration, haemorrhage and inflammation of the intestine, with consequent diarrhoea. Thickening of the intestine may be related to proliferative lesions in the intestine, caused by drugs such as VEGF receptor inhibitors, which lead to proliferation of Brunner’s glands in the duodenum (Ettlin et al., 2010).
The large intestine may show evidence of diarrhoea or constipation. Occasionally, the intestine may display a different colour at necropsy. Some macromolecules derived from drug compounds may accumulate in macrophages within the lamina propria of the small or large intestine or the regional lymph nodes, causing discolouration. Xenobiotics can interfere with motility in the large intestine, causing either diarrhoea or constipation (morphine derivatives), and antibiotics such as tetracyclines change the intestinal microflora, resulting in diarrhoea. Caecal enlargement is associated with increased calcium absorption or ingestion of dietary starches and sugar alcohols, as well as nonabsorbable or high-molecular-weight compounds (Betton, 1998a). Thickening of the small or large intestine may indicate underlying inflammation (i.e. enteritis). Chronic colitis in the mouse is characterised by thickening of the colon and rectum, which is noticeable at necropsy (Figure 5.10). Intestinal tumours are rare, but include polyps (Figure 5.11), adenoma, adenocarcinoma and mesenchymal tumours, such as leiomyoma and leiomyosarcoma (Figure 5.12). Peritonitis is inflammation of the lining of the abdominal cavity and is characterised by yellow strands of fibrin and pus and by turbid fluid in the abdominal cavity at necropsy. Peritonitis is rare in toxicological studies, but may occur if a treatment-related ulcer in the stomach or the small or large intestine perforates and faeces enters the abdominal cavity.

Figure 5.10 Chronic colitis (*) in a mouse.

Figure 5.11 Polyp in the colon of a rat.

Figure 5.12 Leiomyosarcoma extending from the ileum outer surface in a rat.
The pancreas has two elements: the exocrine and the endocrine pancreas (see Section 5.10). Pancreatitis (inflammation of the exocrine pancreas) is an acute and severe condition, and animals will display severe pain (e.g. hunched posture in rodents, the prayer position in dogs). At necropsy, the pancreas will display severe haemorrhage, necrosis (yellow areas) and oedema. Pancreatitis may be caused by drugs such as cyclosporine A (Hirano et al., 1992), but not many compounds are known to cause it. Increased or decreased zymogen granules in the pancreas (only visible under the light microscope) may be caused by reduced feed consumption or sunitinib, an inhibitor of receptor tyrosine kinase (Patyna et al., 2008); this is a frequent finding in short- and long-term safety studies.
Proliferative lesions are visible as small to large masses in the pancreas at necropsy. Proliferative lesions in the pancreas include acinar hyperplasia, adenomas and carcinomas; these may be observed with peroxisome proliferator-activated receptor (PPAR) alpha activators, fibrates (Cattley et al., 2013), as well as heat-labile, soya-bean, trypsin inhibitors (Betton, 1998a).
5.4 Liver
The liver is a common target organ in toxicity studies in animals because oral compounds reach it first via the hepatic portal vein from the gastrointestinal tract. Furthermore, the liver is often responsible for removing all of a compound from the blood, and is thus exposed to the compound in high concentrations. Toxic liver injury is one of the most frequent reasons for terminating the development or therapeutic use of a drug. Test article-related findings in the liver include degeneration, necrosis and regeneration. Liver failure is characterised by jaundice, encephalopathy (neurologic signs such as dullness), bleeding tendency (due to deficiency of clotting factors), odema and hypoproteinaemia.
Liver necrosis and inflammation are common, and are recognised at necropsy by the presence of small to large, nonraised yellow areas present on the reddish-brown surface of the liver (Figure 5.13). Hepatocyte degeneration or necrosis may range from a single cell (only observed under microscope) to multifocal, lobar and massive necrosis. Test article-related liver necrosis is a significant lesion in toxicology, and its presence may mean that a compound cannot proceed to clinical trials (Cattley et al., 2013). Common mechanisms of liver necrosis include depletion of glutathione, interference with mitochondrial energy production and cytoskeleton damage (Grattagliano et al., 2009). Liver necrosis is generally either centrilobular (the most common form, due to the concentration of CYP enzymes in this area and the anoxia that occurs here, at the furthest point from the arteriole), midzonal or periportal. These distributions can only be observed under light microscope, but will often be mentioned in pathology reports as they describe the injury in terms of major liver landmarks (i.e. the portal tract and central vein). If the necrotic injury involves the mesenchymal tissue in the liver, scarring or fibrosis will occur; cirrhosis is defined as a ‘fibrosing nodular liver’.

Figure 5.13 Yellow areas (*) on the reddish-brown surface of the liver, indicating liver necrosis and inflammation.
Hypertrophy or enlargement of the hepatocytes (increase in size, not in number) is a common toxicological liver lesion. It may be observed at necropsy by the presence of a large liver (with increased weight compared to controls) with rounded edges that bulge upon cutting. Hypertrophy of hepatocytes is generally associated with an increased cytoplasmic endoplasmic reticulum due to P450 enzyme induction or peroxisome proliferation. Noted peroxisome proliferators are the hypolipidaemic agents based on clofibric acid and the PPARs. Xenobiotic enzyme inducers (e.g. phenobarbitone) produce enlarged hepatocytes capable of rapidly degrading endogenous hormones such as sex hormones and thyroid-stimulating hormone (TSH). This may result in thyroid hypertrophy, as the thyroid responds to the greater TSH breakdown by producing more TSH. It is thus a good idea to examine the thyroids carefully in animals that display an enlarged liver, as they may be increased in size at necropsy.
A diffuse pale yellow is often seen at necropsy. Lipid accumulation is one of the most common treatment-related findings in the liver, and the liver will generally be increased in weight and may assume a yellow colour and greasy texture. Oil Red O and Sudan black are special stains that can be used on histological sections of the liver (nonfixed) to identify lipid in the liver tissue. Accumulation of pigments (lipofuscin, glycogen) or nonpigments (drug metabolites) may also be a test article-related finding, and may cause a colour change in the liver, which will be visible at necropsy. For instance, hypolipidaemic agents cause an increase in lipofuscin (golden-brown pigment) in rodent liver cells following prolonged treatment (increased lipid peroxidation).
Hepatitis is inflammation of the liver. At necropsy, a swollen, enlarged, often pale yellow or mottled liver can be seen, frequently with thin yellow fibrin strands on the surface. This change is not common as a test article-related finding. Inflammatory cell infiltration into the liver is a common background lesion and is generally only visible under light microscope, but it may rarely be a test article-related finding (e.g. atorvastatin in beagle dogs in chronic toxicity studies causes hepatic microgranulomas). The inflammatory-cell aggregations may be visible macroscopically as tan, white foci in the liver, if severe. Immunosuppressive drugs can cause a decrease in inflammatory cell foci in the liver. Certain xenobiotics may be phagocytosed by Kupffer cells (macrophages of the liver), including nanoparticles (Xiao et al., 2011); occasionally, this may be visible as a colour change in the liver at necropsy.
A generalised yellow colour of the fat tissues and carcase at necropsy is referred to as ‘jaundice’ or ‘icterus’ (Figure 5.14); this may have prehepatic (generally, red blood cell breakdown), heaptic (such as liver inflammation) or posthepatic (obstruction of the large bile duct, preventing the bile from moving to the gall bladder) causes. Examination of the sclera of the eye is a good method for establishing that jaundice is present. Cholestasis is a decreased volume of bile or impaired secretion of specific solutes into the bile, with a resultant increase in the serum levels of bile salts and bilirubin. Icterus may also be caused by the drug chlorpromazine or phalloidin (Betton, 1998b). Test article-related findings in the gall bladder and bile duct include inflammation (cholecystitis), bile-duct necrosis (caused by the compound trabectidin) (Donald et al., 2002) and bile-duct proliferation.

Figure 5.14 Jaundice/icterus in a mouse, visible as a generalised yellow colour of the abdominal muscles.
Liver tumours are generally visible at necropsy as multiple or single masses, often raised above the liver surface, and sometimes round. Hepatic proliferative lesions caused by xenobiotics range from hypertrophy and hyperplasia to hepatocellular adenomas (Figure 5.15) and hepatocellular carcinomas. Foci of cellular alteration (esoinophilic, basophilic, vacuolated and mixed) are not visible macroscopically, but may be test article-related; controversy remains over whether or not these lesions are preneoplastic (Thoolen et al., 2013). Tumours of the bile duct include cholangioma (benign) and cholangiocarcinoma and hepatocholangiocarcinoma (malignant); these generally cause jaundice, because the bile is prevented from leaving the gall bladder by the obstruction of the tumour in the bile duct.

Figure 5.15 Hepatocellular adenoma (*) in the liver of a mouse.
5.5 Respiratory System
It is difficult to visualise the respiratory system whilst animals are living, although the respiration rate will give you lots of information about what is happening in the lungs (an increased respiration rate is seen in cases of pneumonia or lung tumours). A nasal discharge (clear, mucoid or pus) gives an indication of inflammation in the upper respiratory tract. The respiratory system is analysed in detail in inhalation studies, although compounds administered by other routes (such as oral gavage) may also effect the respiratory epithelia via the bloodstream or through inhalation of refluxed material (Lewis and McKevitt, 2013). Generally, in inhalation studies, four sections of the nasal cavity are examined, and three or four standard sections of the rodent nasal turbinates may be cut with reference to the upper palate (Young, 1981). Local metabolism of inhaled or administrated compounds occurs in the nasal epithelia and Bowman’s glands, due to the presence of xenobiotic metabolising enzymes such as P450 (Harkema, 1991). Mice and rats are obligate nose-breathers, and so obstruction to airflow due to inflammation or a tumour may result in swallowing of air, leading to a severely distended, air-filled stomach and intestines, which eventually compresses the diaphragm, causing death by asphyxiation (Figure 5.16).

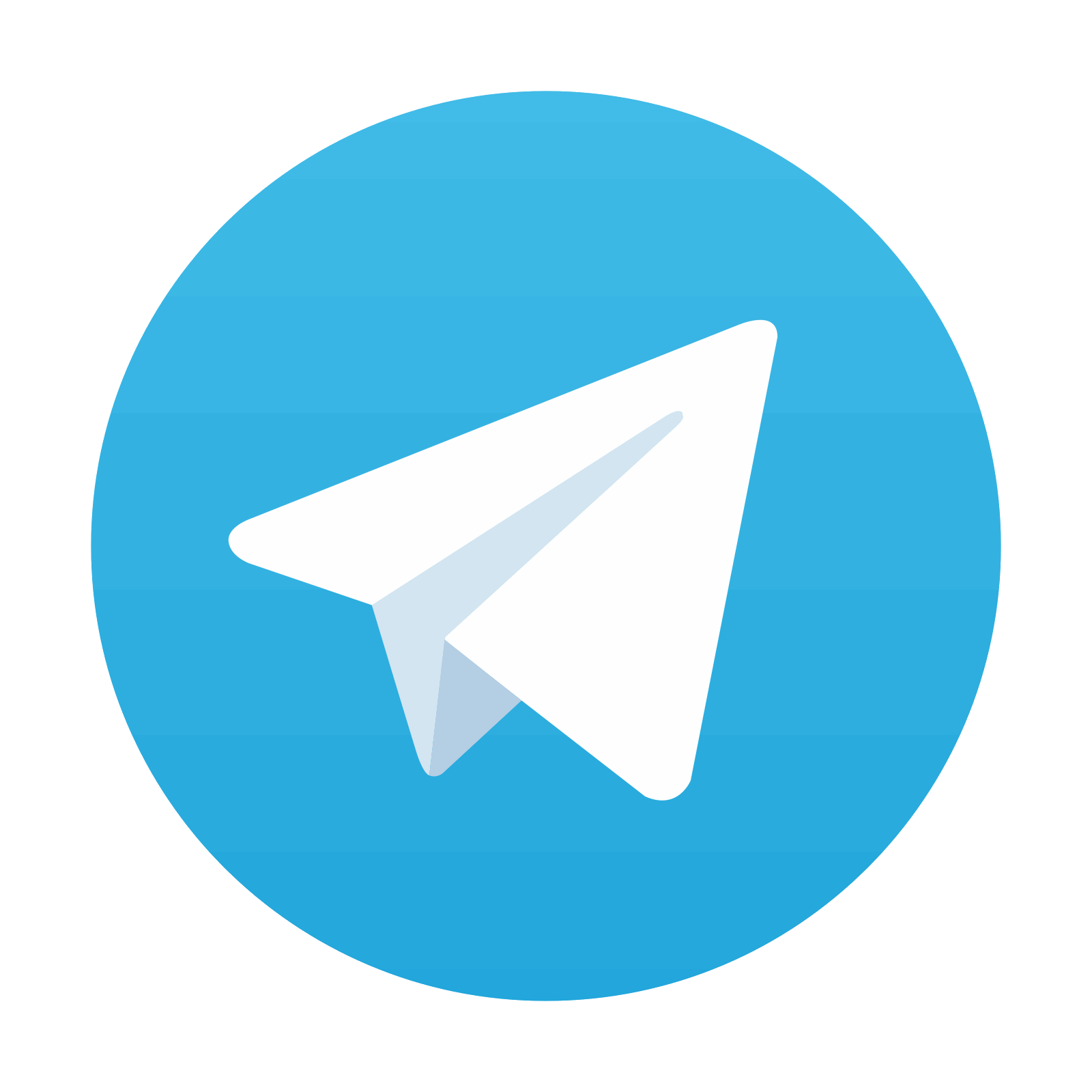
Stay updated, free articles. Join our Telegram channel

Full access? Get Clinical Tree
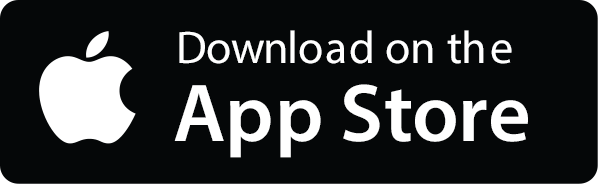
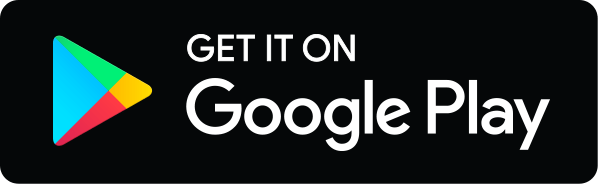