Fig. 6.1.
Development of PV-synaptic contacts in primary neurons cultured from cortical tissue. Cultured cortical neurons were immunostained for simultaneous detection of αCaMKII (left panel: glutamatergic neuron) and parvalbumin (right panel: PV interneuron processes) during their fourth week of development in vitro. Note that PV processes surround the soma and primary dendrites of the glutamatergic neuron, making “en passant” synaptic contacts (beaded structures in right panel ).
1.1 Why Studying PV Interneurons in Culture May Shed Light to Schizophrenia Pathophysiology
The strikingly similar pattern of postnatal development of PV interneurons in vivo and in culture (42) led us to test whether these interneurons were a specific target of prolonged exposure to NMDA-R antagonists. Should this be the case, it would permit the study of the specific effects that blockade of NMDA-Rs have in the development and function of this inhibitory neurons in a simplified system, where only cortical glutamatergic and GABAergic neurons are present.
1.1.1 PV Interneurons in Culture Follow a Preprogrammed Pattern of Development and Respond to NMDA Receptor Blockade Similar to What Is Observed In Vivo
Cortical neuronal cultures give rise to synaptic activity when developed in vitro (43–46). Spontaneous inward currents driven by AMPA-type glutamate receptors have been observed in these cultures during the first week in vitro (47), and expression of inhibitory GABA(A) receptors was observed after 2 weeks in culture (48, 49). This type of cortical culture is widely used for studies of neurodegeneration, excitotoxicity, regulation of ion-channel activity, and neurotrophin or neurotransmitter intracellular signaling (48, 50–55).
The primary culture system offers many advantages when studying mechanisms triggered by NMDA-R antagonists, since in this system, all inputs from outside the cortex are severed during the dissection process and the only relevant neurotransmitters are glutamate and GABA. Over the past 5 years we have accumulated evidence demonstrating the advantages of the primary neuronal culture system in studies of PV-inhibitory neuron development and function, as well as in studies of their dysfunction as a possible cause of schizophrenia (28, 42, 56). These interneurons develop in culture in a strikingly similar manner to what has been shown in vivo, with parvalbumin expression appearing at the end of the first week in vitro and the number of PV-positive neurons increasing steadily to reach 50% of the GABAergic population by the fourth week (39, 42, 57). When analyzed during the fourth week in vitro, the characteristic fast-spiking current patterns of PV interneurons are indistinguishable from those observed at a similar postnatal age in vivo (42, 57). By analyzing NMDA-mediated activation of intracellular signaling pathways, we showed that NMDA-Rs are functional in the cultured interneurons. More importantly, we showed that the composition of NMDA-R subunits in these interneurons is different than that present in pyramidal neurons at a similar age (42). These results have recently been confirmed in PV interneurons of rat prefrontal cortex (58), strongly supporting the applicability of the results we obtained in the primary culture system to an in vivo situation.
With regard to the effects of NMDA-R antagonists on the GABAergic phenotype of PV interneurons, we showed that prolonged exposure to a non-selective NMDA-R antagonist or to an NR2A-preferring antagonist induced the loss of parvalbumin and GAD67 expression exclusively in PV interneurons in culture, without affecting the viability of the neurons. We also showed that these effects were prevented by increasing intracellular calcium concentrations with a calcium channel opener, or by activation of group 1 metabotropic glutamate receptors (42).
1.1.2 NMDA-R Antagonist Exposures Increase Oxidative Stress-Related Mechanism that Leads to the Dysfunction of PV Interneurons In Vitro and In Vivo
In search for the mechanisms by which exposure of NMDA-R antagonists leads to the loss of GABAergic phenotype of PV interneurons, we demonstrated, again using the culture system, that exposures to NMDA-R antagonists lead to disinhibition of excitatory circuits and activation of the superoxide-producing enzyme NADPH oxidase-2 (Nox2) in 3-week-old primary cortical neurons as well as in adult brain. Furthermore, the inhibition of Nox2 with apocynin or the elimination of superoxide with a brain-penetrant SOD mimetic prevented the loss of phenotype of PV interneurons in vitro and in vivo, and ketamine effects were absent in Nox2-deficient animals (28, 56). Exposure to PCP and more selective NMDA-R antagonists such as MK801 and CPP was shown to produce a rapid increase in reactive oxygen and nitrogen species (ROS) in vitro (59) and in vivo (60, 61), and repetitive exposures in vivo led to a substantial elevation of baseline levels of free radicals, suggesting that this treatment results in a persistent change in the oxidative state of the cortex (61). Interestingly, recent results have shown that NMDA receptor activity is required for the expression of antioxidant enzymes (62), further supporting the idea that prolonged blockade of NMDA receptors produces an increased oxidative state in brain.
1.1.3 Inflammatory Mediators Are Involved in the Activation of Oxidative Pathways that Lead to Loss of Function of PV Interneurons
Alterations in cytokine levels have been a consistent finding in schizophrenia patients (63). In particular, elevated plasma levels of IL-6 have been reported in patients and first-degree relatives (64–66), and increased IL-6 levels were found to correlate with exacerbation of psychotic episodes (64–69). To analyze the possible involvement of proinflammatory molecules in the ketamine-mediated activation of Nox2, we turned again to the primary culture system. Using this system, we showed that prolonged exposure to ketamine increased the levels of IL-6 mRNA, without affecting the levels of IL-1β or TNF-α mRNAs, and that IL-6 released from neurons was directly involved in the induction and activation of Nox2 by ketamine (56). This result translated to the in vivo situation where we showed that brain IL-6 levels remained elevated 24 h after the last exposure to the NMDA-R antagonist, and was necessary and sufficient to produce the induction and activation of Nox2, and the consequent loss of the GABAergic phenotype of PV interneurons (56).
In summary, the use of the primary culture system permitted a detailed study of the mechanisms by which NMDA-R antagonists produce the loss of the GABAergic phenotype of exclusively PV interneurons and led to the discovery of the IL-6/Nox2 pathway as intrinsically involved in the enduring effects of NMDA-R antagonists on the PV-inhibitory circuit in vivo (28, 42, 56).
2 Materials
For the preparation of primary cultures, the following materials are necessary.
2.1 Equipment
Dissection microscope
Horizontal flow clean bench (dissection hood)
70% Ethanol
Sterile dissection tools:
#3c and #4 forceps
Scissors (blunt tips: 9 and 15 cm)
Forceps (serrated tip: 12 and 16 cm; 1×2 teeth: 10 cm)
Stainless steel tray with lid
2.2 Media
1.
Dissection media (DM)
Prepare a 10× DM the following way:
280 mM glucose 25 g
200 mM sucrose 35 g
4.2 mM NaHCO3 1.75 g
10× HBSS 500 ml
Dissolve thoroughly, filter sterilize, and store at 4°C.
2.
Media stock (MS) (1.09 l)
H2O (sterile) 900 ml
10× MEM 100 ml
GB stock (see below) 90 ml
Glucose bicarbonate (GB) stock (for 1 l)
NaHCO3 (35.3 mM) 29.66 g
Glucose (246.6 mM) 44.44 g
H2O to 1 l
Filter, sterilize, and keep at 4°C.
The quality of the sterile water is essential for healthy cultures. If in doubt, purchase it from a trusted supplier.
3.
Astrocyte plating media:
FBS* 100 ml
Horse serum (HS)* 100 ml
Glutamine (200 mM) 10 ml
Pen-Strep (100×) 10 ml
MS to 1 l
4.
Astrocyte growth media:
1× MEM 422 ml
Glutamine (200 mM) 5 ml
Glucose (20%) 18 ml
Pyruvate (100 mM) 5 ml
HS* 50 ml
In general we purchase sterile glutamine and pyruvate already in solution at the indicated concentrations.
5.
Neuron plating media:
1× MEM 90 ml
200 mM Glutamine 1 ml
100 mM Pyruvate 1 ml
Glucose (20%) 3 ml
HS * 10 ml
6.
Neuron growth media:
FBS* 50 ml
HS* 50 ml
200 mM Glutamine 10 ml
MS to 1 l
7.
Neuron maintenance media:
HS* 10 ml
200 mM Glutamine 10 ml
MS 80 ml
8.
MS/N2.1
N-2 supplements 1 ml
100 mM Pyruvate 1 ml
200 mM Glutamine 1 ml
HS* 2 ml
MS 95 ml
*Always use endotoxin-free, characterized, and heat-inactivated sera for preparing neuronal cultures. We always check different batches of sera beforehand by preparing one 24-well Primaria plate of one-step cortical cultures as described below.
2.3 Coverslips
Besides the material and media described above, you will need the following:
1.
Poly-l-lysine-coated coverslips: For preparing these coverslips, follow exactly the procedure described by Goslin and collaborators (70). The quality of the glass and the preparation of these coverslips are fundamental to the success of the neuronal cultures, principally for the dissociated cultures used in confocal microscopy. For the latter cultures, wax “feet” will need to be added to the coverslips before poly-l-lysine coating as described by Goslin and colleagues.
2.
Coat the above coverslips (with or without feet) with neuron plating media in the tissue culture incubator for at least 5 days before using them.
3 Method for Preparing Mouse Primary Neocortical Cultures
The preparation of mouse cortical neuronal cultures for use in studies of postnatal development of inhibitory interneurons follows well-established protocols that have been optimized by different labs over the last 20 years. Basically, the primary culture system we have used is the one developed for studies of the neurobiology of synaptic development and neurodegeneration, to which we have introduced small modifications to optimize conditions for the correct maturation of the different inhibitory interneuronal types present in cortical tissue. Depending on the type of study, we have used three slightly different ways of culturing cortical neurons which all strictly depend on healthy astrocytes. For all types of cortical neuronal cultures, the preparation and growth of astrocytes, over which embryonic neurons are developed, is fundamental to the success of long-term cortical neuronal cultures. Careful control of the growth of astrocytes has produced, in our hands, cultures lasting for more than 3 months.
3.1 Dissection Procedure and Preparation of Astrocytes
1.
On the day of astrocyte preparation, place thoroughly washed tools in the tray and fill to cover with 70% ethanol. Sterilize for more than 15 min. Working now in a tissue culture hood, discard or recycle the ethanol and place the tools over sterile pads to dry. Keep the tray in the hood because it will be used to carry the tools to the dissection hood after drying. You can further sterilize by turning on the UV lamp in the hood while the tools are drying (about 20 min). Once dried, place them back in the tray and cover it.
2.
Place neonatal (P1–3) mouse pups on ice until they are pale and no longer move (see Note 1).
3.
Sterilize skin with 70% ethanol.
4.
Place pup heads in cold dissection media (DM) in a 100-mm Petri dish.
5.
In a dissection hood, remove the brains and transfer them into a clean 100-mm Petri dish with cold DM.
6.
Under a dissection microscope, remove the meninges and dissect the cerebral hemispheres. Carefully removing all the meninges reduces the amount of endothelial cells, macrophages, and microglia contaminations in the cultures.
7.
Collect the hemispheres in a 60-mm dish containing cold DM and chop the tissue with small scissors.
8.
Transfer the tissue into a 50-ml conical tube.
9.
Add 5 ml of 0.25% trypsin and 1.5 ml of 1% DNase to the tube, bringing the final volume to 13.5 ml with DM.
10.
Incubate at 37°C in water bath for 15 min, swirling occasionally.
11.
Let tissue settle and transfer the supernatant into a new 50-ml conical tube through sterile cell strainer (70-μm nylon mesh) to remove large chunks of tissue.
12.
Add 3 ml horse serum to inhibit trypsin.
13.
To the remaining tissue, add another 5 ml of 0.25% trypsin and 1.5 ml of 1% DNase. And again bring the volume to 15 ml with DM.
14.
Incubate at 37°C as before.
15.
Pour supernatant through the nylon mesh, including remaining chunks of tissue. Do not pipette tissue up and down to dissociate!
16.
Add 3 ml horse serum through the mesh to inhibit trypsin and to wash the remaining tissue.
17.
Centrifuge cells for 5 min at 1000 rpm and resuspend in astrocyte plating media.
18.
Plate cells (three pups/flask) in 75-cm Falcon Primaria flask in 15 ml astrocyte plating media with the addition of 15 μl of EGF (10 μg/ml).
19.
Replace media with the same astrocyte plating media after 2 days if there is too much debris.
20.
Incubate flasks in 37°C/5% CO2 incubator until 80–90% confluency (see Note 2).
21.
The day before removing the astrocytes from the flasks, prepare 12-well or 24-well poly-d-lysine-coated plates. If you use Primaria plates, there is no need to coat them. However, Primaria does not have 12-well plates, so these need to be coated in the following way: dissolve 5 mg of poly-d-lysine (Sigma Cat# P7280) in 50 ml sterile water. Add sufficient amount to completely cover the wells and incubate for >5 h in the tissue culture incubator. Wash three times with sterile water and let air-dry in the tissue culture hood.
22.
Discard the media from the flask containing the astrocytes and wash in PBS (no Ca2+ no Mg2+). If microglia are present, cap the flask tightly and tap it against a hard surface (such as the bench of the tissue culture hood) to release the microglia. Wash again with D-PBS and then incubate at 37°C in 3 ml of 0.25% trypsin for approximately 10 min. You may need to bang the flask against your hand to release the astrocytes (do not use a pipette) and transfer to a 15-ml conical tube. Wash the flask with another 5 ml astrocyte plating media, add this to the previous cells, and spin down as before. Gently resuspend cells in 5 ml of astrocyte growth media with a widemouthed, 5-ml pipette and dilute at a ratio of 2 ml of cells into 48 ml of astrocyte growth media.
23.
Plate in 12-well poly-d-lysine-coated plates (1 ml/well).
24.
Feed by exchanging half of the media once a week only if media become acidic (yellowish).
Astrocytes prepared in this manner are used as feeder layers when preparing two-step or dissociated primary neuronal cultures.
When used as feeder layers for two-step primary neuronal cultures, astrocytes should be allowed to grow to complete confluency in growth media. In general, we use astrocytes plates that have been confluent for about 3 weeks before seeding primary neurons on top of them (see Note 3).
For using as feeder layers for dissociated neuronal cultures, astrocytes should be transferred to MS/N2.1 media when they have reached about 50–60% confluency (see Note 4). These feeder layers should be transferred to MS/N2.1 a week in advance of use.
3.2 Dissection and Preparation of Primary Cortical Neurons
The procedure described below will render two 12-well plates of one-step cultures, and two 12-well plates of dissociated neuronal cultures. If you want to scale up, follow the same procedure up to disaggregating the cells. Then dilute in the corresponding volume of neuron plating media such that the final dilution is 1.25 cortices per 10 ml.
3.2.1 One-Step Cultures
These are the cultures of choice when performing electrophysiological recordings or single-neuron transcriptional analysis (42):
1.
Dissect cortices of two E15 embryos in DM as described for newborn pups in the preparation of astrocytes, making sure you remove the hippocampus.
2.
Collect all four cortices into a 15-ml sterile conical tube and let them settle in the bottom of the tube. Aspirate off the media and add 5 ml of neuron growth media.
3.
Using a widemouthed, 5-ml pipette attached to a pipettor, place the mouth of the pipette against the bottom of the conical tube and gently triturate the tissue until no aggregates are observed (be careful to avoid air getting into the pipette and making bubbles). It usually takes around 5–8 times filling the pipette up and down to the 4-ml mark to disaggregate the tissue. Add to 27 ml of neuron plating media in a 50-ml conical tube.
4.
Mix and plate 1 ml in each well of two 12-well plates containing poly-l-lysine-coated coverslips that have been treated for 5 days in neuron plating media. Alternatively, for testing of sera, or for biochemical studies, you can plate 0.4 ml in each well of a 24-well Primaria plate. Save the leftover cells for the preparation of dissociated neuronal cultures as described below.
5.
Place the plates in a tissue culture incubator (5% CO2) and monitor astrocyte growth and neuronal differentiation. The astrocyte monolayer should be confluent in about 12 days. Do not feed the cells during the first 2 weeks after plating. The plating media is sufficiently rich to sustain growth and differentiation of these low-density cultures. When the astrocyte layer is confluent (usually between 10–14 days in vitro), add cytosine arabinoside (Ara-C) to a final concentration of 10 μM.
6.
After 48 h of Ara-C addition, exchange 0.5 ml of media with neuron maintenance media. In general, these low-density cultures will need to be fed with neuron maintenance media once a week.
3.2.2 Dissociated Neuronal Cultures
These types of cultures are especially suitable for experiments of intracellular signaling and confocal microscopy analyses:
1.
Add neuron growth media to the ∼6 ml leftover cells from the one-step culture preparation to a final volume of 7.5 ml.
2.
Add 300 μl of the above neuronal suspension onto each poly-l-lysine-coated coverslip that has been incubated in plating media for at least 5 days. Do not exchange media. Incubate overnight in tissue culture incubator.
3.
The next day, using sterile forceps and a sterile syringe needle with its tip bent, invert the “feeted” coverslips onto 12-well astrocyte plates that have been grown to ∼50–60% confluency and incubated in MS/N2.1 for 1 week. Do not exchange the MS/N2.1 in the astrocyte plates.
4.
Add Ara-C (5 μM final concentration) to prevent astrocyte growth on the coverslip.
5.
Monitor the development of neurons once a week and feed with MS/N2.1 only if media becomes yellowish (see Note 5 on monitoring the development of neurons).
3.2.3 Immunocytochemistry Methods to Study Parvalbumin Interneurons in Culture
Due to the low number of PV interneurons, both in vivo and in culture, analysis of protein expression patterns as well as signal transduction mechanisms can be studied only by immunocytochemical methods. Thus, the availability of suitable antibodies is a must when studying these interneurons. Following is the protocol we have used over the last 10 years that results in reliable and reproducible fixation of PV interneurons in culture.
Fixation and Blocking
1.
Wash coverslips twice with PBS to remove growth or treatment media, by carefully aspirating half of the media and adding 0.5 ml of sterile PBS. Aspiration must be performed with low vacuum and the addition of PBS is best performed with a widemouthed pipette (not automatic) against the wall of the well to prevent the formation of a vortex in the center that will lift up the neurons. Add 1 ml of ice-cold 4% paraformaldehyde in PBS. This is performed sequentially for each coverslip. Each well should be washed and fixed separately.
2.
Incubate at room temperature for 30 min.
3.
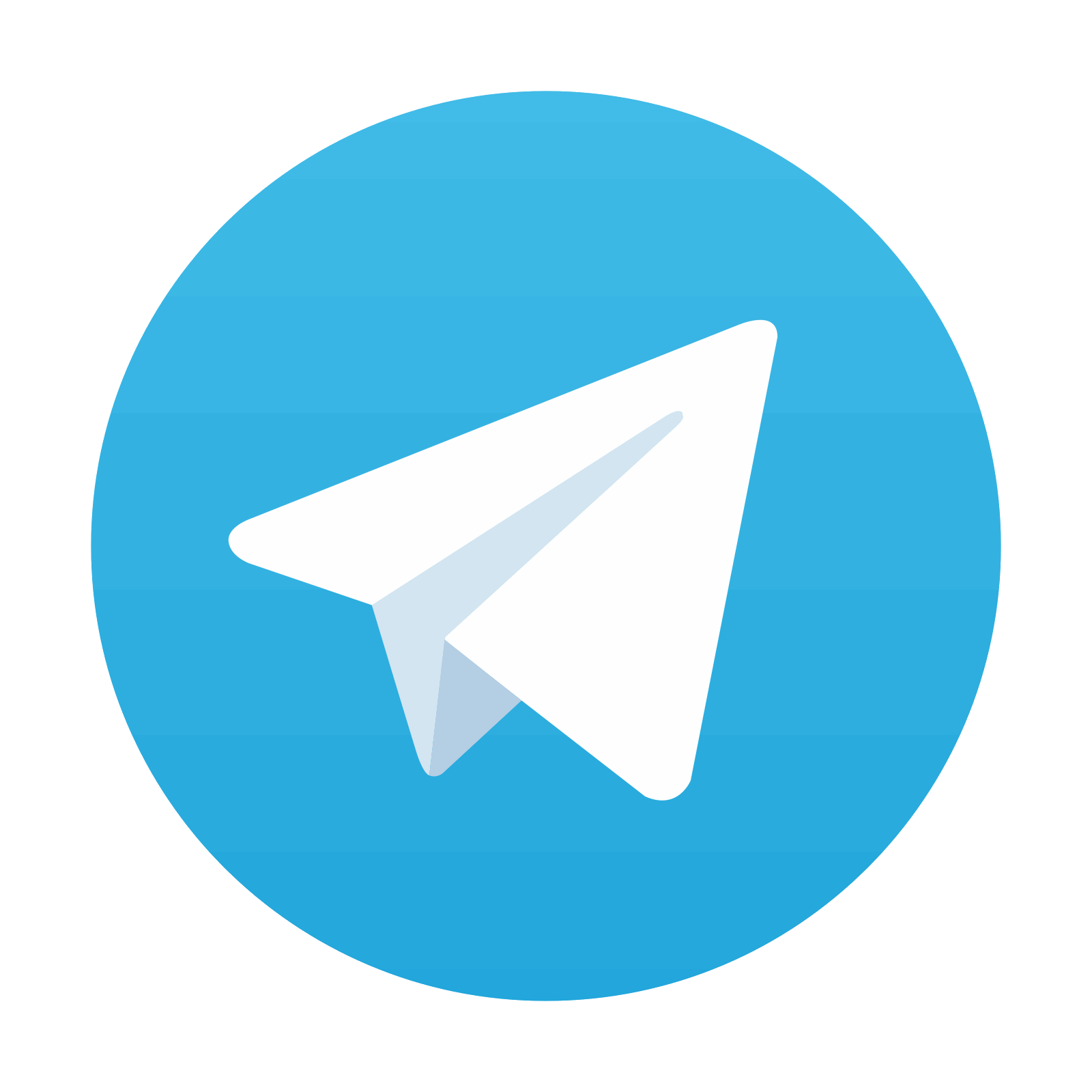
Wash carefully with PBS three times. Once the neurons are fixed, the fixative as well as the washes can be completely aspirated. However, vortexes can still occur, so the same rules apply as for when fixing.
< div class='tao-gold-member'>
Only gold members can continue reading. Log In or Register a > to continue
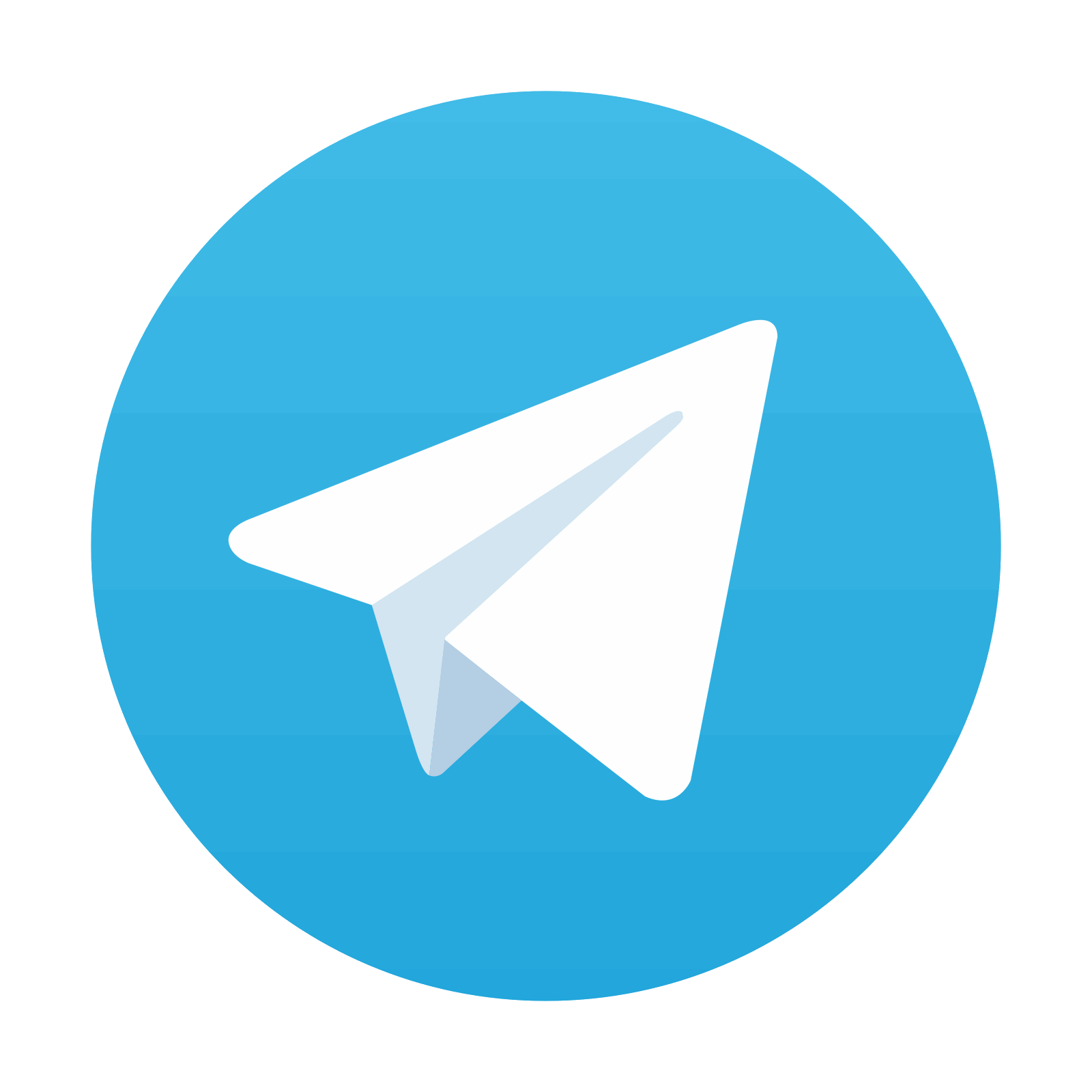
Stay updated, free articles. Join our Telegram channel

Full access? Get Clinical Tree
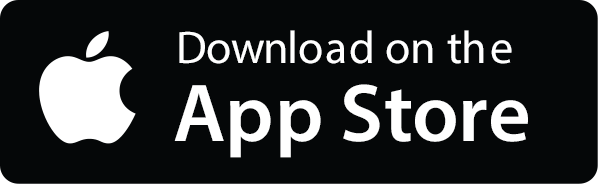
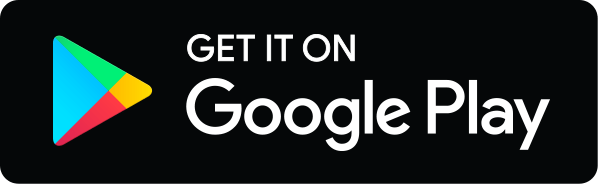
