Fig. 1
Mechanisms for free radical production in DM: 1 glucose autoxidation, leading to direct production of ROS; 2 production of advanced glycation end-products, causing protein damages and production of NO; 3 activation of polyol pathway, with sorbitol accumulation and consumption of NADPH; and 4 activation of protein kinase C, activating vasal cells, and inducing vasal permeability
1.
Glucose autoxidation is thought to be the main source of free radicals. Glucose, in its enediol form is oxidised to an enediol radical anion, then converted into ketoaldehydes and superoxide anion radical. Superoxide anion (SO) is the main source of other radicals such as hydrogen peroxide, singlet oxygen, or, in the presence of transitional metals, highly reactive hydroxyl radicals. On the other side, superoxide also reacts with nitric oxide (NO) to reactive peroxinitrite radicals. All these reactive oxygen species (ROS) promote lipid peroxidation, destruction of membranes, and cleavage of DNA, thus inducing cell destruction. Moreover, increased free radical concentration, together with secondary impaired antioxidant content, causes alterations of structural and enzyme proteins, membrane lipids, and nucleic acids, both nuclear and mitochondrial, leading to cell damage or death.
2.
Advanced glycation end-products (AGE) formation is another important mechanism, typical of oxidative stress in Diabetes mellitus (DM). AGE are stable substances resulting from the nonenzymatic glycosilation of proteins through Schiff bases and an intermediate Amadori product. AGE are an heterogeneous group of molecules, whose accumulation in tissues, by means of the linkage with specific receptors (RAGE), leads to inactivation of intracellular enzymes, promotes ROS production, and activates the transcription factor NF-kB. NF-kB acts on the upregulation of many target genes and induces the production of NO. All these mechanisms contribute to aging and destruction of cells including islet beta cells, thus accelerating diabetes onset and complications.
3.
Activation of polyol pathway is promoted by the elevated concentration of aldose reductase (AR) in some tissues such as the crystalline lens and nervous system. In hyperglycaemic conditions glucose passively enters insulin-independent cells (such as crystalline cells, neurons, and erythrocytes), saturates hexokinase (which promotes the production of glucose-6-phosphate), and strongly promotes activation of AR. AR induces the reduction of glucose to sorbitol, which is generally converted by sorbitol dehydrogenase (SDH) in fructose. However, in some cells AR concentration strongly exceeds SDH, thus sorbitol accumulates inducing osmotic cellular swelling and promoting some diabetic complications such as cataract. Moreover, increased activity of AR requires NADPH, thus leading to depletion of NADPH content and reducing the availability of NADPH for regeneration of reduced glutathione via glutathione reductase.
4.
Activation of protein kinase C (PKC) pathway has been reported to be initiated by intracellular accumulation of glucose that causes de novo synthesis of diacylglycerol (DAG), which stimulates PKC. PKC plays a central role in activation of cells, finally leading to an increased production of extracellular matrix and cytokines and to enhanced contractility and permeability of vascular cells. The increased concentration of DAG is probably tissue-specific and may be involved in the pathogenesis of many complications of DM including cardiovascular and renal ones.
Increased production of ROS and depletion of antioxidant content is related both to the onset and progression of DM and to the development of diabetic complications.
Islet beta cells are particularly sensitive to ROS because their content of antioxidant substances is lower than that of other cell types. In type 1 diabetes the cause of insulin decrease is destruction of beta islet cells due to autoimmunitary insulitis. The death of islet beta cells is most likely mediated by the contact with activated macrophages and T lymphocytes and by the exposition to soluble mediators by cytokines, ROS and NO. In type 2 DM, insulin is not decreased, at least at the first phase of the disease, and hyperglycaemia is often associated with hyperlipidaemia. This causes an increased mitochondrial production of superoxide and the activation of inducible nitric oxide synthetase (iNOS), thus leading to the production of peroxinitrite, cellular damage, and impairment of response to endogenous insulin. Recently great attention to mitochondrial dysfunction and production of ROS in type 2 has been focused even if the pathogenesis has not been completely elucidated.
Oxidative Stress and Diabetic Complications
High levels of free radicals cause damage to cellular proteins, membrane lipids, and nucleic acids and increase cellular death. Many ROS have been reported as correlated with different diabetic complications. However, the development of most diabetic complications have to be considered multifactorial, due to the interaction of different pathways leading to oxidative stress. Moreover, it must be considered that most complications are the result of the imbalance between production and abolition of ROS, that is, the increased ROS production and/or the decreased concentration of antioxidants. Oxidative stress has been widely demonstrated as related to diabetic complications in human medicine.
Cardiovascular Complications
Diabetes has been reported as an important risk factor for the development of cardiovascular diseases in humans such as coronary heart diseases, peripheral arterial diseases, hypertension, stroke, and cardiomyopathy. Cardiovascular complications are major causes of death in human diabetic patients [16]. The imbalance of pro-oxidants and antioxidants with excessive, destructive free radical chemistry is at the basis of most of these complications and plasma reduced glutathione (GSH) concentration can provide a good indicator of it.
AGE can also be related with coronary disease by interfering with LDL lipoprotein and facilitating deposition of cholesterol in atherosclerotic plaques in human diabetic patients with hyperlipidaemia.
Peripheral Microvascular Complications
Peripheral vascular diseases are common in human diabetic patients. Many studies show that ROS can play a role in vascular dysfunction and transforming, via oxidative damage, by decreasing the bioavailability of NO, by damaging endothelium, by accelerating endothelial cell migration, and by initiating inflammatory reaction. This leads to endothelial dysfunction which can evolve to hypertension and atherosclerosis [39]. Microvascular complications of diabetes carry a high morbidity and, when associated with macrovascular and cardiovascular complications, also high mortality in human medicine.
Neuropathy
The main pathogenesis of diabetic neuropathy is linked to increased apoptosis of neurons and glial cells due to two main mechanisms: peroxidation of lipids present in plasma, mitochondria, or endoplasmic reticulum membranes; and peroxidation of proteins by some antiapoptotic molecules (apoptosis inhibitory factor, complex 3, Bcl-2) and increasing the expression of some proapoptotic stress proteins.
In contrast with glial cells, neurons, as nondividing cells, are less prone to oxidative stress-induced DNA damages, yet mitochondrial DNA is quite sensitive to oxidative damage thus impairing energy regulation in high energy-requiring neurons. Both neuronal and glial damages may be at the basis of several neurodegenerative diseases [36].
Retinopathy
Retinopathy is one of the most important microvascular and long-term complications in diabetic human patients. It develops in stages and it leads to blindness and visual impairment. The central point leading to diabetic retinopathy is swelling of blood vessels, leaking fluids, neovascularisation, and detachment of the retina. Continued high plasma glucose concentration induces damages via repeated acute and also chronic cumulative changes. Hyperglycaemia damages the tight junction between endothelial cells and promotes endothelial apoptosis, thus increasing vascular permeability and leading to swelling of the retina. An attempt to compensate ischemia by means of new vasal production induces retinal detachment and blindness.
Compared with other tissues, the retina is particularly sensitive to oxidative stress because of the high content of polyunsaturated fatty acids. Another retinal peculiarity concerns the resistance to reverse retinal dysfunction after good glycaemic control is reinstituted. This is probably due to the accumulation of ROS and damaged molecules that are not easily removable after re-establishment of normoglycaemia [21].
Nephropathy
The principal event leading to diabetic nephropathy is the accumulation of extracellular matrix (ECM) protein in the glomerular mesangium and tubulointerstitium. Several different mechanisms are involved including an imbalance between the synthesis and degradation of ECM proteins that, because of their slow turnover, are especially susceptible to oxidative stress and glycation. Glycation of ECM components, leading to AGE formation, induces structural alterations and increased resistance to proteolytic digestion. The accumulation of ECM proteins is particularly apparent in the glomerular basal membrane and can induce microalbuminuria, a very sensitive early marker of diabetic nephropathy. AGE also interact with RAGE influencing the expression of growth factors and cytokines regulating the proliferation of different renal cells. Finally it has also been demonstrated that AGE interact with the renin–angiotensin system and that the use of angiotensin-converting enzyme (ACE) inhibitors reduces the production of AGE and the formation of ROS in experimental DM [15].
Cataract
Cataract is another ocular complication of DM also in human diabetic patients and it is characterised by opacification of the crystalline lens correlated both to the duration and the severity of hyperglycaemia. Several mechanisms contribute to the onset of diabetic cataracts including swelling of the crystalline lens due to osmotic changes, glycosylation of lens proteins and decreased concentration of antioxidants. In particular great attention has been given to the increased concentration of sorbitol due to the elevated AR activity in the lens and to insulin-independency of crystalline cells. This causes increased water influx and thickening of fibres and subsequent cataract.
The concentration of sorbitol in crystalline has been found to be elevated in diabetic human patients and correlated to the one of the other insulin-independent tissues such as erythrocytes [17]. The use of AR inhibitors (tolrestat, epalrestat, ranirestat) to prevent cataract in experimental DM has been proposed in rats but at the moment these drugs are not suggested in management of patients with DM.
Another central point is the cross-linking of lens protein due to nonenzymatic glycosylation. The lens is characterised by an elevated content of long-lived proteins that are exceptionally stable and keep their transparency. The crystalline lens cells have virtually no turnover, thus they readily accumulate AGE which cause oxidation of thiol groups, cross-link formation, and aggregation of the crystalline proteins producing the high molecular weight insoluble molecules responsible for opacification. Moreover, glycation of membrane ATPase, such as Na-K pump, alters intracellular ion concentration and water movement via osmosis [1].
Ketoacidosis
Diabetic ketoacidosis (DKA) is probably the most common short-term complication occurring in diabetic patients. In contrast with other diabetic complications, in whose pathogenesis oxidative stress has been reported, little is known about the correlation between ketoacidosis and oxidative stress. Vantyghem et al. [35] measured malonildialdehyde (MDA) and total antioxidant status (TAS) in diabetic patients with DKA in poorly controlled diabetics without ketoacidosis, in well-compensated DM, and in control subjects, and found that MDA was increased and TAS decreased in DM with DKA but no statistical differences were found with poorly controlled DM. In 2002, Lee et al. [24] found that diabetic patients with DKA showed higher lipid peroxidation and lower vitamin A concentrations than normal and that after correction of DKA, lipid peroxidation increased and the concentrations of vitamins C and E decreased. These results suggest that oxidative stress can be linked both to ketoacidosis and poor compensation of DM even if no direct effect of ketone bodies on production of ROS has been demonstrated. However, the administration of antioxidant vitamins during treatment for DKA can be strongly recommended.
Use of Antioxidant to Prevent Diabetic Complications
Even though many studies suggested the linkage between oxidative stress and diabetic complications, at the moment no direct evidence that ROS production can directly cause or initiate diabetic complications is available as much as hyperglycaemia, hyperketonaemia, and high free fatty acid (FFA) concentrations could act to increase oxidative stress. On the other hand, an important issue is the possible use of antioxidants to prevent, stop, or delay the progression of DM or its complications.
Many different antioxidants, vitamins, coenzymes, and minerals, singularly or in combination, have been proposed and in some nonclinical trials, both on cultured cells and diabetic animal models, positive results in preventing ROS production or diabetic complications have been found [20, 22, 33]. However, large clinical trials on human patients have not been successful, perhaps because of the multiple pathways used by hyperglycaemia to mediate its adverse effects. The introduction of new multifunctional antioxidants might help to reach more clinical results in the near future.
Markers Useful in Monitoring Oxidant Stress in DM
Another important issue is the possible use of markers of oxidative stress to evaluate the level of compensation of DM, to monitor the effect of therapy, and to predict complications. Plasma markers are the simplest to use, however, in some cases, specific cells, such as erythrocytes (RBC), can be used as a good model of what can be found in other tissues in which it is difficult to test oxidative stress in vivo.
Evaluation of Oxidative Stress
Many different biomarkers of oxidative stress have been evaluated in DM, similarly to those already reported for other diseases. The same laboratory tests used in DM are validated for evaluation of oxidative stress in other conditions, thus the principle of the assays is not discussed in the present chapter.
Lipid peroxidation has been evaluated by means of fluorometric or spectrophotometric assays for thiobarbituric acid reactive substances (TBARS), total radical-trapping potential (TRAP), and MDA. They resulted in an increase in both natural and experimentally induced DM in plasma, RBC, and different organs such as the pancreas, lens, retina, nerve, and kidney. The increase of TBARS has been shown to be prevented by treatment with various antioxidants.
The concentration of the major intracellular redox substance, reduced glutathione (GSH), is another frequent test, based on a colorimetric assay that allows us to detect intracellular GSH concentration easily in several cells. In DM glutathione has been found to be decreased in the liver, kidney, pancreas, RBC, nerve, and precataractous lens. On the contrary, plasma concentration of GSH is usually below the lower concentration of linearity of the test, thus it is rarely evaluable.
Enzyme activities such as glutathione peroxidase (GPX) and reductase (GR) that are involved in the detoxification from hydrogen peroxide and regeneration of GSH can be assessed in cells by means of spectrophotometric kinetic tests. Even if there is not complete agreement on the activities of these enzymes in DM, GPX is found to be elevated in most tissues whereas it is decreased in the heart and retina and GR is reduced in the retina and plasma and increased in the heart.
Activities of other scavenging enzymes such as catalase and superoxide dismutase (SOD) can be easily tested in different organs. Catalase is reported to be elevated in the heart, liver, brain, and kidney of diabetic laboratory animals, however, these results are not consistent in all the studies. The same ambiguous results are available for SOD.
< div class='tao-gold-member'>
Only gold members can continue reading. Log In or Register a > to continue
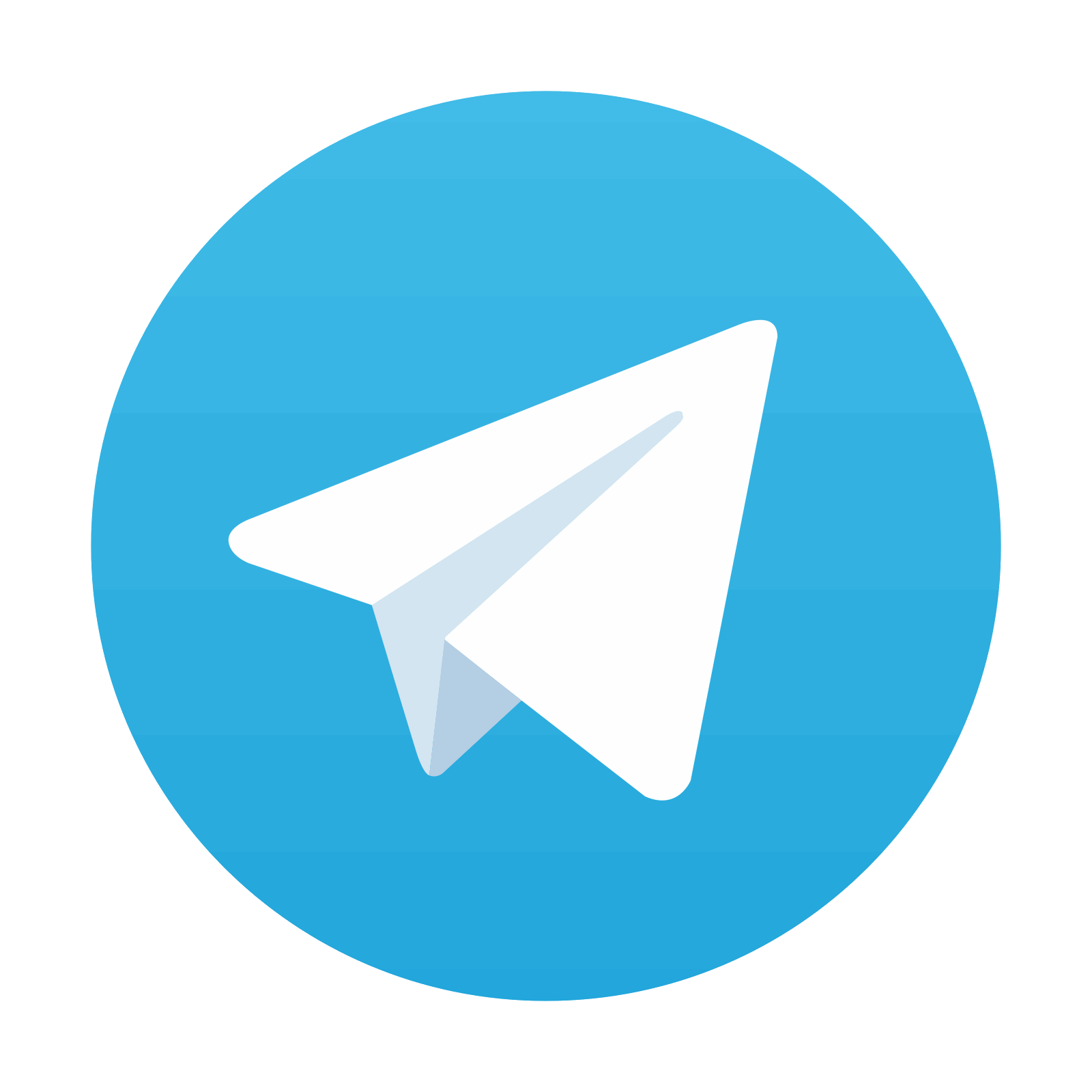
Stay updated, free articles. Join our Telegram channel

Full access? Get Clinical Tree
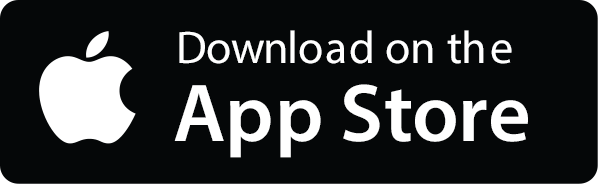
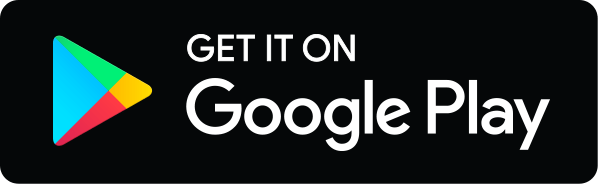