Chapter 4.1
Stem cell therapy in veterinary dermatology
Background – Adult stem cells come from many sources and have the capacity to differentiate into many cell types, including those of the skin. The most commonly studied stem cells are those termed mesenchymal stem cells (MSCs), which are easily isolated from bone marrow and adipose tissue. Mesenchymal stem cells are known to produce a wide array of cytokines that modulate the regeneration process. The ease of collection, propagation and use of these MSCs in therapy of traumatic, ischaemic and immune-mediated skin conditions is emerging.
Approach and evidence – In traumatic and ischaemic skin damage, MSCs are used in tissue-engineered skin and by direct injection into damaged tissue. For immune-mediated diseases, systemic administration of stem cells can modulate the immune system. The earliest clinical work has been with autologous stem cell sources, such as adipose tissue and bone marrow. In immune-mediated diseases, the MSCs are used to downregulate production of inflammatory cytokines and to block T-cell activation. Cells are generally given intravenously. Multiple sclerosis, rheumatoid arthritis and lupus have been successfully treated in human clinical trials. Mesenchymal stem cells can also stimulate resident local cells, such as keratinocytes and progenitor cells, to proliferate, migrate and repair skin injury and disease.
Looking ahead – The discovery of the MSC in adipose tissue has spawned a global effort to utilize these cells in therapy of a wide range of diseases of the skin. Reconstructive surgery, scar blocking and resolution and skin regeneration have all been shown to be possible in human and animal studies.
Introduction
Stem cell therapy is not a new discipline. The first stem cell transplants were performed in the 1950s using bone marrow to reconstitute the marrow of chemotherapy patients.1 These stem cells were primarily haematopoietic and were used to replace the damaged or dead marrow stem cells so that the patient could replenish the white and red cell lineages. In the 1980s, a new subpopulation of marrow cells was discovered and named the mesenchymal stem cells (MSCs), in the belief that this cell type was from the mesenchyme and could be induced to differentiate into a mesenchymal tissue cell type, such as bone or cartilage.2 In the ensuing decades, stem/progenitor cells have been identified in nearly every tissue of the body. In 1998, James Thompson at the University of Wisconsin was the first researcher to isolate and propagate human embryonic stem cells (ESCs) and ushered in the era of ‘stem cell medicine’. Although thought to be the most pleuripotent stem cell, the ESC has not yet proved to be a clinically useful cell type, owing to major risks of tumourigenesis and immune rejection.3 On the contrary, the adult MSC has been shown to be nontumourigenic and nonimmunogenic. The clinical interest in MSCs has focused on their ability to modulate the immune system, to stimulate tissue regeneration and to differentiate into all three germ layer lineages. In vitro models, preclinical animal models and early clinical translation provide evidence that stem cells can prove to be safe and efficacious in the therapy of dermatological conditions in human and veterinary medicine. This review chronicles the progression of basic and clinical research in support of the clinical use of the MSC.
Sources and types of stem cells
What are stem cells? In traditional textbooks, it was taught that stem cells were the origin of all major tissue types and that once cells became partly or totally differentiated into a cell type they were now terminal cells and could not dedifferentiate. It is now known that cells are very ‘plastic’ and can transform more than originally thought.4 As usual, the situation is much more intricate and complicated than the initial studies indicated. Table 1 summarizes the characteristics of several classes of stem cells. There are two major types of stem cells, the ESCs and adult stem cells (ASCs). Embryonic stem cells come from the inner cell mass of an early embryo. Once removed, they can be grown in vitro and are generally immortal in cell culture. They can be induced in vitro to form a very large number of cell/tissue types; however, their nature is to form a whole organism. In research, they are already a very powerful tool in the discovery of mechanisms of healing, disease pathogenesis and organ formation. However, to the clinician, they still have two very critical flaws that block their use in the clinic, as follows: (i) they form teratomas when implanted into a patient; and (ii) they are a foreign genotype and can potentially be rejected by the recipient’s immune system.3 In addition, there is a major controversy about the use of embryos to harvest these cells.
Table 1. A summary of stem celltypes and generalcharacteristics
Adult stem cells are different from ESCs. They are found in almost every tissue of every human and animal and are used by the body to make repairs in everyday life. Some of these stem cells, particularly MSCs, have the ability to produce large amounts of growth factors and can differentiate into many body tissues. They do not form teratomas when injected into patients unless they have been damaged in the laboratory and, if they are from the same patient (autologous), they will not be rejected as foreign. Neonatal stem cells from the placenta, umbilical cord, amnion, etc. are generally considered to be ASCs, because they behave in the manner described above. Induced pleuripotent stem cells are adult cells that have been genetically reprogrammed to revert to a more undifferentiated state. Like ESCs, they can form teratomas and they have also recently been reported to be immunogenic, even if the source of adult cells is from the same patient.5
What are the sources of adult stem cells? They are found in nearly every tissue, including bone marrow, fat, skin, nerve tissue, muscle and many others. If the source is from a different species than the recipient (e.g. pig valves for people) they are called xenogeneic. If they are from the same species but a different individual (e.g. kidney for transplant) they are called allogeneic. If the donor is the recipient then they are termed autologous. The only tissue with adequate concentrations of ASCs for direct (uncultured) use is the adipose depot. All other sources need to be expanded ex vivo in order to have a therapeutic dosage available to treat a patient.
An interesting canine stem cell source was recently identified in the hair follicle bulge.6 Human bulge stem cells have been previously identified, but this is the first verification that there are canine bulge stem cells that share many characteristics with the human bulge cells. This bulge region is found as a subtle swelling of the outer root sheath in both the canine and human hair follicle, near the insertion point of the arrector pili muscle.6 The stem cells were found using labelling techniques at this site during anagen phase, but also in the secondary germ at the bottom of the follicle during telogen, leading to the hypothesis that these are the cells responsible for regeneration of the dermal component of hair follicles during cycling.6 Further discussion of the canine bulge stem cell will be covered in the section on alopecia below.
Identification and characterization of the mesenchymal stem cell
Although there is no single ‘marker’ or identifying characteristic that clearly identifies the MSC, there are a combination of characteristics that are generally accepted to be representative of this cell type. The International Society of Cell Therapy has published their guidelines on the characterization of the MSC.7 The proposed primary characteristics are as follows: (i) the MSC must be plastic adherent when maintained in standard culture conditions; (ii) the MSC must express cell surface markers CD105, CD73 and CD90; lack expression of CD45, CD34, CD14 or CD11b, CD79a or CD19, and HLA-DR surface molecules; and (iii) the MSC must be capable of differentiating into osteoblasts, adipocytes and chondroblasts in vitro. Although stem cell scientists continue to develop more stringent criteria, these basic criteria are generally accepted as the baseline for declaring a cell type as a MSC.
Figure 1 demonstrates the third primary characteristic of the MSC, namely differentiation. These four photomicrographs show adipogenic, chondrogenic, neurogenic and osteogenic differentiation of adipose-derived stem cells (ADSCs).8
Figure 1. Photomicrographs of positive differentiation assays for the following adipose-derived stem cells: adipogenic (a); chondrogenic (b); neurogenic (c); and osteogenic (d). The inset photomicrograph below (c) is a higher magnification view of the neurogenic differentiation slide showing the typical neuronal phenotype. This study used standardized induction media known to induce each specific phenotype, and immunostaining was not employed
(reprinted with permission from Stem Cells and Development (Vol 21(16)), published by Mary Ann Liebert, Inc., New Rochelle, NY).8

Additionally, researchers use gene arrays to evaluate whether the cell type in question is able to express the genes thought to be related to the abilities of stem cells. Common genes expressed in progenitor cells include nerve growth factor receptor, basic fibroblast growth factor 2, frizzled homolog 9 and activated leukocyte cell adhesion molecule. In a recent publication, the authors used these genes in a dolphin skin regeneration clinical study to verify that the cells of interest expressed these genes.8 The International Society of Cell Therapy has not adopted any gene array criteria for MSCs at this time.
Mechanisms of action of the mesenchymal stem cell
In contrast to drug or growth factor therapy, stem cell therapy does not rely upon a single target receptor or a single pathway for its action. In stem cell therapy, the ASCs are delivered either directly to the area of trauma or disease (e.g. wounds, tendonitis or osteoarthritis) or are delivered systemically (e.g. liver disease, renal disease or immune-mediated disease). Both delivery methods take advantage of the ability of stem cells to differentiate into many tissue types and their ability to ‘communicate’ with the cells of their local environment through paracrine modalities to create the optimal environment for natural healing.4
While therapeutically successful, the detailed molecular mechanisms of stem cell-related healing are complex and remain under investigation. Repair occurs through a complex variety of demonstrated stem cell functions, including the following: (i) trophic support;4,9,10 (ii) – anti-inflammatory and immunomodulatory functions;11,12 (iii) revascularization;13 (iv) anti-apoptotic activity;13 (v) differentiating capacity;14 and (vi) homing.15 Although the mechanisms remain under investigation, clinical efficacy is documented in preclinical human and animal trials.16 The public have long used aspirin to decrease fever and control pain, beginning in the late 1800s, but the mechanisms of action were not discovered until 1971 when British pharmacologist John Robert Vane discovered the suppression of prostaglandins and received the Nobel Prize in 1981.17
Stem cell therapy delivers a population of cells able to communicate with other cells in their local environment. Until recently, differentiation was thought to be the primary function of stem cells. However, the functions of regenerative cells are now known to be much more diverse, including immune modulation18 and secretion of cell signalling factors and cytokines that influence both local and remote cell populations.4 These cellular functions are implicated in a highly integrated and complex network. Cellular therapy should be viewed as a complex, yet balanced, approach to a therapeutic goal where the cells take their signals from the microenvironment of the injured tissue. Unlike traditional medicine, in which one drug targets one or a few receptors, a single stem cell therapy can be applied in a wide variety of injuries and diseases.
Trophic support
Multiple studies demonstrate that ASCs secrete bioactive levels of cytokines and growth factors that support angiogenesis, tissue remodelling, differentiation and anti-apoptotic events.10,13 Adipose and bone marrow stem cells secrete a number of angiogenesis-related cytokines, such as vascular endothelial growth factor, hepatocyte growth factor, basic fibroblast growth factor, granulocyte-macrophage colony-stimulating factor and transforming growth factor-β.10,13
Anti-inflammatory/immunomodulatory functions
In general, in vitro studies demonstrate that bone marrow stem cells (BMSCs) and ADSCs limit inflammatory responses and promote anti-inflammatory pathways. When present in an inflammatory environment, BMSCs may alter the cytokine secretion profile of dendritic cell subsets and T-cell subsets, causing a shift from a pro-inflammatory environment to an anti-inflammatory or tolerant environment.12 Bone marrow stem cells and ADSCs do not express MHC class II antigens or costimulatory molecules and they suppress T-cell proliferation.19 Adult stem cells suppress mixed lymphocyte reactions and inhibit T-cell proliferation induced by a third cell type or by mitogenic factors.20 Both types of stem cells are able to control lethal graft-versus-host disease that occurs in mice after haploidentical haematopoietic transplantation.18,20
Revascularization
The stromal vascular fraction extracted from adipose tissue contains ADSCs and endothelial progenitor cells that promote angiogenesis and neovascularization by the secretion of cytokines, such as hepatocyte growth factor, vascular endothelial growth factor, placental growth factor, transforming growth factor, fibroblast growth factor and angiopoietin.21 In an in vivo model of hindlimb ischaemia, intravenous injection of ADSCs was associated with an increase in blood flow and vascular density and incorporation of the cells in the leg vasculature.22
Anti-apoptosis
Apoptosis is defined as a programmed cell death or ‘cell suicide’, an event that is genetically controlled. In normal conditions, apoptosis determines the lifespan and coordinated removal of cells. When acutely injured tissue is denied critical blood flow, ischaemia will result. Adipose-derived stem cells significantly reduced endothelial cell apoptosis during ischaemic events in animal studies.13 Rehman et al.13 demonstrated that ADSCs express factors that support cell survival and avoid apoptosis.
Differentiating capacity
Studies using stem cells from a variety of sources demonstrate a diverse plasticity, including differentiation into adipogenic, osteogenic, chondrogenic, myogenic, cardiomyogenic, endothelial, hepatogenic, neurogenic, epithelial and haematopoietic lineages.4 These data are supported by in vivo experiments and functional studies that demonstrated the regenerative capacity of stem cells to repair damaged or diseased tissue via transplant engraftment and differentiation.23 Nixon et al.24 demonstrated statistically significant improvement in histological repair of a collagenase-induced injury in the superficial digital flexor tendon in horses treated with autologous regenerative cells harvested from fat.
Homing
Homing (chemotaxis) is an event by which a cell migrates from one area of the body to a distant site, where it may be needed for a given physiological event. Homing is an important function of adult stem cells and one mechanism by which intravenous or parenteral administration of MSCs permits a therapeutic cell to target a specific diseased area effectively. A cerebral arterial occlusion model of stroke demonstrated that labelled BMSCs administered intravenously 24 h and 7 days postinjury migrated to the area of injury, dramatically reducing the stroke infarct size.25 Mesenchymal stem cells homed to the lung in response to injury and reduced inflammation and collagen deposition in a mouse model of pulmonary fibrosis.26
The mechanism of how stem cells home to injured tissues and migrate across endothelium is not fully understood. It is likely that injured tissues express specific receptors for chemokines and ligands to facilitate trafficking, adhesion and infiltration of stem cells, as is the case with recruitment of leukocytes to sites of inflammation. Chemokines and adhesion molecules play a significant role in the trafficking of leukocytes, and BMSCs have been shown to express some of these molecules.15
Engineered skin
The discipline of tissue engineering attempts to use scaffolding and cells to create a tissue construct, grown ex-vivo. In the case of skin, the construct can be epidermis, dermis or some combination, to create a transplantable tissue. This engineered tissue can be used in place of autografts or noncellular scaffolding to repair damaged skin, such as in trauma, burns or ischaemic tissue. The largest barrier to the successful transplantation of engineered skin is vascularization. An epidermal graft must be nourished by the passive diffusion of nutrients from dermal capillaries. Early studies by Boyce27 demonstrated that engineered grafts required 10-15 days before dermal capillaries could effectively form. This length of time is not compatible with graft survival. Adipose-derived stem cells co-cultured with human fibroblasts can create a vascularized skin construct.28 This type of construct shows the use of the revascularization capability of stem cells in a combination engineered graft.
Although there are no commercially available canine, equine or feline tissue-engineered products, the human market gives evidence that such grafts are possible, practical and commercially successful (Dermagraft; Advanced Biohealing, Westport, CT, USA, recently purchased by Shire Pharmaceuticals). Dermagraft provides neonatal tissue-derived fibroblasts cultured on a degradable matrix.
Mesenchymal stem cell therapy of chronic nonhealing wounds
Healing of wounds requires the coordination of cell migration, cellular proliferation and differentiation and the assembly of scaffolding (extracellular matrix). Additionally, angiogenesis is required to create the vascular supply route. Typically, a granulation bed forms, and re-epithelialization occurs. In chronic wounds, a dysregulation occurs, resulting in a wound that does not heal by the normal course. As described in the preceding subsections, stem cells have the ability to home to areas of inflammation, interact with the local microenvironment, attract other progenitor cells, differentiate into various tissues types and produce a wide variety of cytokines and growth factors that could influence healing in a chronic wound. Human ADSCs can influence the migration and proliferation of human dermal fibroblasts and, in rodent models, can reduce the time for wound closure.29
A number of rodent models have shown that MSCs applied intravenously,30 topically31 and subcutaneously32 can improve wound healing. Human clinical studies have demonstrated the healing of chronic fistulas in patients with Crohn’s disease, both by local delivery and by the intravenous route.33 Reports on the clinical application of BMSCs in human wound therapies have demonstrated that grafted MSCs facilitate skin regeneration, both in acute and in chronic wounds.34–36 Falanga et al.35 used autologous bone marrow MSCs delivered in a fibrin spray to accelerate healing in human cutaneous wounds.
In a recent study, standardized skin wounds in the dolphin (Turchiops truncates) were treated with cultured ADSCs in a blinded, placebo-controlled clinical study.8 Wound tissue biopsy samples were evaluated by a blinded, independent histopathologist, with analysis of cell proliferation (number of mitoses) and healing rate on days 1, 5 and 15 after treatment. Wounds in dolphins treated with autologous ADSCs showed improved healing compared with the saline placebo-treated dolphins (Figure 2).8
Figure 2. Dolphin skin wound biopsy samples obtained on day 15 after intralesional treatment with adipose-derived cultured autologous stem cells (a) and phosphate-buffered saline (b). There was improved healing in the stem cell-treated group. The arrows show the site of the original wound and illustrate the superior filling in the treated versus control lesion.
Images courtesy of Vet-Stem, Inc., Poway, CA, USA.

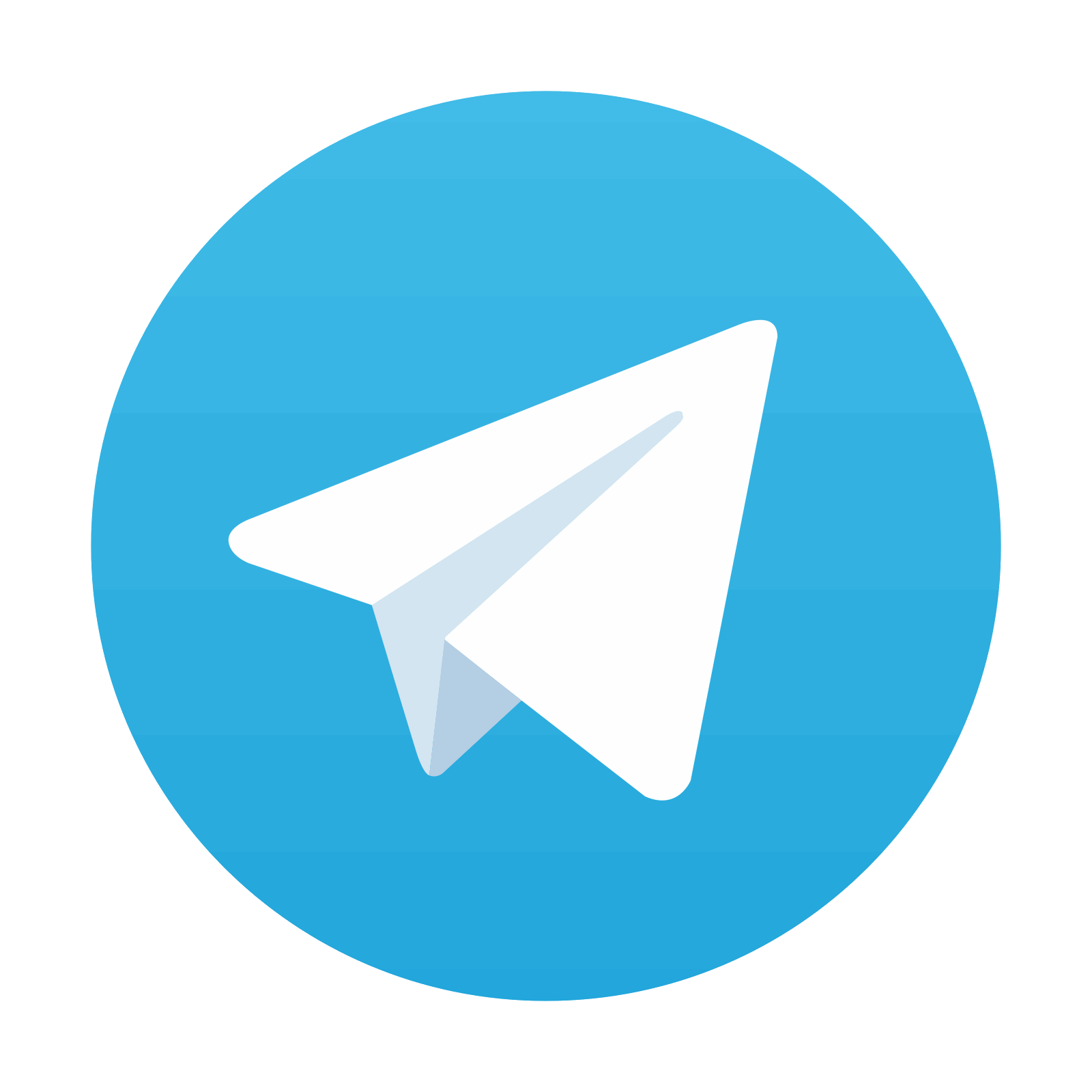
Stay updated, free articles. Join our Telegram channel

Full access? Get Clinical Tree
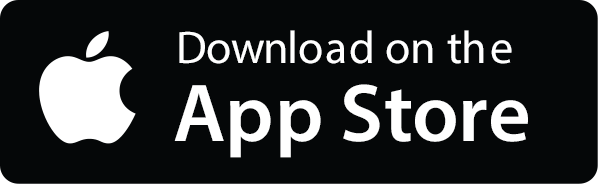
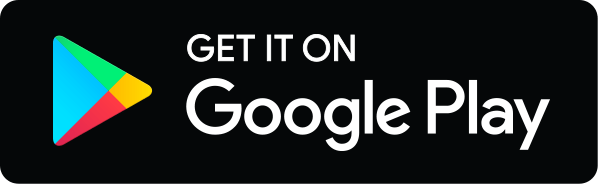