Simon T. Kornberg1 and Brigitte A. Brisson2 1ACVIM, Southeast Veterinary Neurology, Miami, Florida, USA 2CVS, University of Guelph, Guelph, Ontario, Canada Vertebral column fractures and luxation pose a unique challenge due to their variability in location, severity of instability, and various associated lesions within the injury site. Often, fractures will occur alongside luxation, traumatic disk extrusions, and other systemic injuries. Due to these variations and potential co‐morbidities, careful decision‐making aided by cross‐sectional imaging, sound surgical principles, and proper implant selection is essential in assuring good, lasting outcomes. In traumatic cases, whether it be from a fall, vehicular accident, bite wounds, projectile injuries, or other, it can be assumed that the patient may be systemically unstable. Proper triage and systemic stabilization should be performed as necessary. If deemed safe to do so, a complete neurologic examination to localize the lesion accurately and to provide a prognosis should be performed prior to providing sedation/analgesia. Cranial nerves should also be examined to ensure there is no evidence of head trauma. Initial patient assessment may include abdominal and thoracic focused assessments using ultrasound and thoracic, abdominal, and spinal radiographs or awake trauma CT to assess for pulmonary lesions, bladder rupture, and potential spinal instabilities that may require immediate intervention or considerations when handling the patient. Adequate pain control should be provided, and in the case of open fractures, antibiotic coverage may also be prudent. Standard orthogonal radiographs of the spine or trauma CT should be obtained to assess location, and severity of injury. This may also help with further selection of cross‐sectional imaging modalities. Ideally, both magnetic resonance imaging (MRI) and computerized tomography (CT) should be utilized when assessing spinal injuries. MRI allows for enhanced assessment of the spinal cord, adjacent soft tissue injury, and traumatic disk extrusions. CT allows superior assessment of bone, 3D reconstruction, and is sensitive in detecting the instability even if the lesion is nondisplaced. It should be utilized to aid implant selection by assessing patient specific anatomy, implant corridors, insertion angles for safe placement, and optimal cortical bone purchase [1] (Figure 12.1). The three‐compartment concept [2] should be utilized in the decision‐making process when assessing where or whether spinal instability is present. This concept divides the vertebral column into dorsal, middle, and ventral compartments. The dorsal compartment comprises the spinous process, dorsal ligamentous structures, lamina, articular processes, and pedicles. The middle compartment comprises the dorsal longitudinal ligament, dorsal annulus, and dorsal aspect of the vertebral body. The ventral compartment comprises the remaining vertebral body, nucleus pulposus, remainder of annulus, and the ventral longitudinal ligament. When two or more compartments are compromised, the lesion should be considered unstable (Figure 12.2). The need for stabilization can be assessed by utilizing this concept; however, other considerations such as neurologic status, chronicity, concurrent injuries, and imaging findings should also be considered in the decision‐making process (Figure 12.3). Figure 12.1 (a) Axial CT with superimposed pin insertion angle and location. (b) Postoperative axial CT of the same dog with a bicortical pin placed. Figure 12.2 Illustration of the three compartments in the lumbar vertebrae. The dorsal compartment in RED includes the spinous processes, dorsal ligamentous structures, lamina, articular facets, and pedicles. The middle compartment in BLUE consists of the dorsal longitudinal ligament, the dorsal annulus, the floor of the vertebral canal, and the transverse processes. The ventral compartment in YELLOW includes the vertebral body, the annulus fibrosus, the nucleus pulposus, and the ventral longitudinal ligament. Figure 12.3 Axial CT of thoracic vertebrae, two sequential CT slices (a is cranial to b) showing nondisplaced fractures of the vertebral body (ventral compartment), floor of the vertebral canal (middle compartment), and pedicle and articular facet (dorsal compartment). This is an example of an unstable but nondisplaced fracture evidenced by multicompartmental involvement. Due to the unpredictable nature of traumatic injury, concurrent compression with bone fragments, disk material, or hemorrhage may be present. It may therefore be necessary to combine stabilization of the spine with spinal cord decompression techniques. Cross‐sectional imaging with both MRI and CT is the most reliable method to determine whether decompression is needed in addition to spinal stabilization. Other indications for spinal stabilization include certain iatrogenic interventions that can unavoidably cause theoretical destabilization of the spine. Examples of this include bilateral hemilaminectomy over multiple vertebrae, radical dorsal laminectomy, and hemilaminectomy with corpectomy [3]. These procedures may be necessary in certain patients who require surgery for IVDD, tumor removal, or correction of a vertebral malformation. It is essential to apply the three‐compartment concept to determine whether stabilization is required and carefully plan surgical approaches and fixation placement in these patients. When considering reduction, while complete anatomical alignment is preferable, on occasion it may not be possible due to chronicity or extent of the injury. In these cases, stabilization is still beneficial and should be performed even with suboptimal alignment. Pre‐drilling is necessary for the implantation of cortical screws. A 1.5‐mm drill bit is used prior to inserting a 2.0‐mm bone screw. A 2.0‐mm drill bit is used prior to inserting a 2.7‐mm screw, and a 2.7‐mm drill bit is used prior to inserting a 3.5‐mm screw (Table 12.1). High‐speed drilling and low‐speed screw insertion is recommended to prevent thermal necrosis. Stacked drill guides, drill stops, and – more recently – 3D printed custom drill guides can be used to safely drill to the ideal depth and insertion angle [4, 5]. Pre‐drilling is also recommended for insertion of positive‐profile threaded pins. It has been shown to decrease microfractures during pin insertion and to allow the removal of loose bone dust that could otherwise become impacted in the insertion corridor and could lead to instability of the implant over time. Pre‐drilling is achieved using a drill bit of a slightly smaller gauge than the intended pin followed by low speed power (150 rpm) pin insertion. Smaller dogs’ spines can generally accommodate 5/64th or 3/32nd inch pins, while 1/8th inch pins are typical for larger dogs. The sharp pin tip can be cut prior to insertion into the pre‐drilled bone to prevent inadvertent iatrogenic injury to soft tissues at the point of exit (Table 12.1) (Figure 12.4). Table 12.1 Suggested screw and pin sizes with corresponding drill bit size for pre‐drilling. When selecting an implant, it is important to consider surgical experience, anatomical considerations, and type of stability needed. The most commonly used implants include screws and plates and screws/pins and PMMA. Bone plates come in two major types: conventional and locking. Conventional, nonlocking plates require precise contouring and friction so they must sit flush against the surface of the bone to maintain fixation. These also require bicortical purchase which can be technically challenging to achieve in the spine. Due to the undulating anatomy precluding consistent contact of the bone plate, impracticality of bicortical fixation in some locations, and high motion causing shear forces in multiple directions, conventional plates are generally suboptimal for spinal fixation compared to newer locking plates and PMMA constructs in both versatility, implant stability, and ease of application. Conversely, locking plates do not require friction or bone contact in order to provide rigid fixation. These work by various mechanisms that lock the screws into the plate, creating a rigid construct that does not require bone to plate contact. Locking plates allow for a monocortical fixation that is low profile but without the drawbacks of a conventional plate. Locking plates, with some specific exceptions, require screws to be placed perpendicularly through the holes in order to engage the locking mechanism. This can prove a challenge when placing implants in predefined vertebral corridors and provides limited flexibility in this regard. Although not theoretically necessary, it is preferable to contour a locking plate as close as possible to the vertebral body to reduce the torque applied to the screw head and neck and reduce the risk of failure due to screw pull‐out or shear. There are a variety of proprietary locking implants available. Examples include the locking compression plate (LCP) plate by Synthes, the polyaxial locking plate system (PAX) system by Securos, the string of pearls (SOP) plate and the newer Pedicle Screw System, both by Orthomed. While LCP plates require the plate to be perpendicular to the screw insertion into the bone, the latter three mentioned offer various degrees of flexibility with insertion angles by either contouring the plate (SOP), providing screw angle flexibility through the plate holes (PAX), or allowing the screws to be placed first and the rods to be attached and tightened at the nodes (Pedicle Screw System). Discussions on which system is best is beyond the scope of this chapter, and the decision to use either of the systems should be made with the individual case in mind and by the comfort and familiarity of the surgeon with the system (Figure 12.5). Figure 12.4 Illustration of the application of a positive‐profile pin bicortically in a lumbar vertebra. (a) The vertebral body is pre‐drilled at a pre‐measured angle and depth using anatomical landmarks. Care should be taken to avoid advancing the drill bit past the transcortex. (b) A depth gauge is used to probe the corridor to determine the length and integrity. This ensures that adequate depth and purchase can be obtained with the implant. (c) A positive‐profile pin is placed using low power (150 rpm) insertion. This should be advanced to the predetermined depth, measured from preoperative imaging. Positive profile pins and polymethyl methacrylate (PMMA) constructs offer greater flexibility in implant placement, as the pins can be placed in a desired location and angle with the PMMA molded over to incorporate the construct. Positive‐profile threaded Steinmann pins are preferred over cortical screws when incorporated in PMMA, due to their more substantial core diameter which resists shear forces and reduces thread failure [6]. Bicortical pin placement is recommended for PMMA constructs. PMMA should be mixed thoroughly according to directions provided by the manufacturer. Although it can be molded manually by hand and placed into the construct, syringe application allows for more homogeneous mixing and leads to greater uniformity of the structure through the PMMA bridge, creating a more stable construct [7]. This can be achieved through a large catheter tip syringe, with perforations placed by the surgeon to allow air to be evacuated. The plunger of the syringe should be removed and the tip of the syringe should be blocked while the viscous mixture is poured into the proximal end of the syringe. The plunger should then be replaced and with the catheter tip still blocked, air should be evacuated with light depression of the plunger, avoiding the mixture escaping through the perforations. The PMMA should then be applied with the syringe and molded using either an osteotome, freer periosteal elevator, or similar. Care should be taken to incorporate all screw heads or pins and ensure that the construct is evenly distributed without air pockets or gaps. During the curing process, an exothermic reaction occurs. This can damage adjacent soft tissue structures so copious amounts of sterile saline should be flushed while the curing process takes place; this is especially important if spinal cord decompression was performed. Once cooled and hardened, it is important to assess the structure for rigidity. Some surgeons advocate adding antibiotics into the PMMA mixture. This is controversial as it may disrupt the strength of the PMMA construct [8]. PMMA constructs can be challenging to remove in the event of complications or infection (Figure 12.6). Figure 12.5 Examples of available locking plate systems (a and b) bilateral application of SOP plates in the lumbar spine. (c) Model depicting bilateral application of the Pedicle Screw System in the lumbar spine. (d) Postoperative lateral radiograph of Pedicle Screw System application in the lumbosacral spine. Source: Courtesy of Dr. Oscar Thamar‐Torres, DVM. Figure 12.6 Intraoperative images of two different pin and PMMA constructs (a) prior to placement of PMMA and (b) following placement of PMMA. Note that the pins in (b) could have been cut shorter prior to PMMA application. When applied correctly, both the PMMA construct and various locking plate constructs are appropriate methods for spinal fixation [9, 10]. Theoretically, stainless steel has a much greater fatigue resistance compared to PMMA. This is further supported by the tendency of PMMA constructs to fail where the screw enters the PMMA, when compared to plates which fail at the neck of the screw, likely due to a stress concentration effect [7]. in vivo, it is difficult to ascertain which one of these methods is more effective, and it is likely dependent on anatomy and operator experience. Using the largest possible core diameter screws or positive‐profile pins and ensuring adequate bone engagement is the most important aspect of selecting a fixation method. The thoracolumbar spine is the most common location for spinal stabilization, with T13–L1 being most affected as it is the interface between the more stable thoracic and the more flexible lumbar spine, creating a natural stress riser at this location. Unstable high thoracic fractures and luxations are uncommon due to the stabilizing nature of the ribs and more prominent thoracic limb musculature. Hyperflexion injuries are more commonly seen and typically result in caudal endplate fractures at the associated disk space. This can also lead to concurrent intervertebral disk extrusion.
12
Stabilization of the Thoracolumbar Spine
Preoperative Planning
Decompression with Stabilization
Technique
Dog size
Recommended cortical screw size
Recommended pin size
Drill bit size for pre‐drilling
Small
2.0
5/64
1.5
Medium
2.7
3/32
2.0
Large
3.5
1/8
2.7
Implant Selection
Thoracolumbar Spine
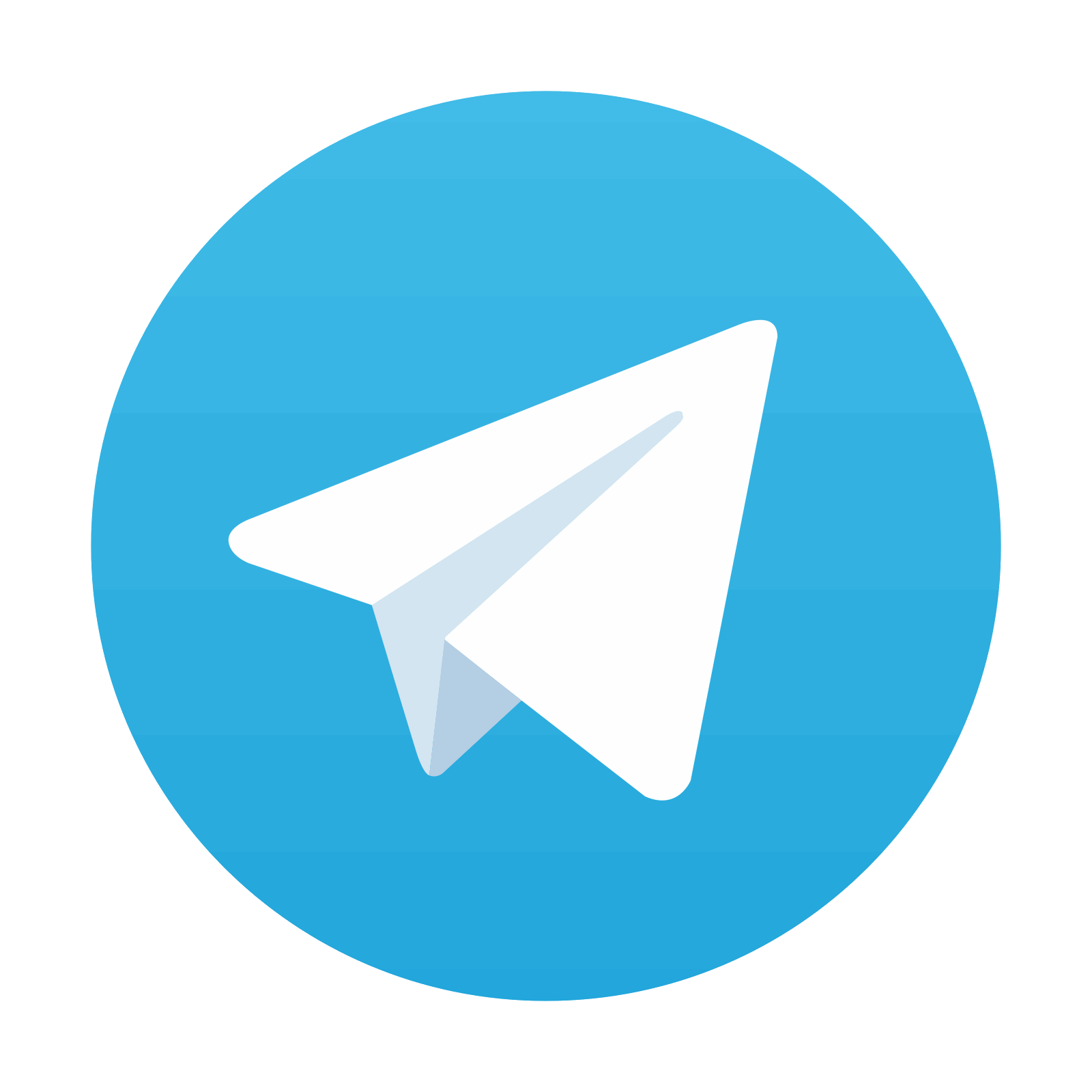
Stay updated, free articles. Join our Telegram channel

Full access? Get Clinical Tree
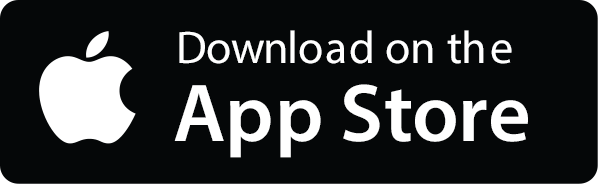
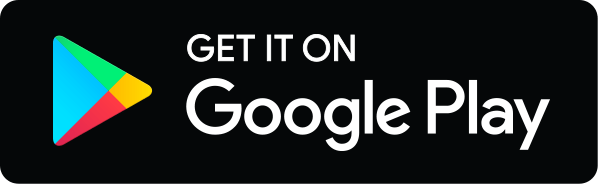