Fig. 15.1
Differential dynamic behaviour of sperm DNA damage in two ejaculates from two different rams after incubation of the sample for 24 h in INRA-84 at 37 °C. Numbers inserted in the figure correspond to the level of sperm DNA damage registered during different incubation times
While an understanding of dynamics can reveal intra-specific variation between individuals (López-Fernández et al. 2008a; Gosálvez et al. 2009; Johnston et al. 2012a), we have also discovered significant variation in the rate of sperm DNA degradation between species (Gosálvez et al. 2011a). It is highly likely that this variation is a reflection of the structural and biochemical composition of the sperm chromatin. A dramatic example of major differences in the way that the DNA molecule responds can be seen by comparing frozen-thawed rhinoceros and koala spermatozoa. All three species of rhinoceros that have been examined show a massive degradation of sperm DNA integrity following thawing (Portas et al. 2009), whereas koala spermatozoa show little if any major increase in the rate of DNA fragmentation during post-thaw incubation (Zee et al. 2009a; Johnston et al. 2012b). Rhinoceros spermatozoa possess cysteine residues designed to stabilise the DNA through disulphide bonding and yet show rapid degradation, whereas the koala lacks cysteine residues in its suite of protamines, and thereby appears to maintain its stability using a completely different mechanism. It is therefore fundamental when conducting or interpreting sperm chromatin assays that each individual species be appropriately validated. It stands to reason that differences in chromatin protein chemistry should be reflected in differential responses in the assessment of DNA fragmentation. It seems, for example, that the lack of protamine 2 in some species significantly reduces the likelihood of sperm DNA fragmentation (Gosálvez et al. 2011a). While greater numbers of cysteine residues in protamine 1 tend to confer increased sperm DNA stability, this argument is only likely to be relevant for eutherian sperm. Comparative evolutionary studies involving investigation and validation of SDF assays for different species will provide important insights into the way that the chromatin is packaged and its differential response to stressors, such as environmental toxins or cryopreservation.
3 How Should Sperm DNA Fragmentation Be Assessed?
Several techniques have been developed to assess SDF in humans and other animal species. One of the first experimental approaches performed to measure SDF was dual emission of an altered DNA molecule interacting with fluorescent cationic dye assessed with flow cytometry; this is known as the sperm chromatin structure assay—SCSA—(Evenson 1990). The underlying principle for this method involves subjecting the DNA to mild acid in order to denature ds-DB or ss-DB. This process produces a combination of single stranded DNA stretches originating from the pre-existing DNA breaks, together with orthodox Watson–Crick double stranded DNA molecules. Using the metachromatic characteristics of the fluorochrome, acridine orange, it is possible to produce DNA labelling based on a colour code capable of differentiating damaged from undamaged spermatozoa; those spermatozoa which fluoresce green have mostly double-stranded non-denatured DNA, while red-orange fluorescence is indicative of single stranded DNA motifs. This methodology allows for the objective quantification of spermatozoa with fragmented DNA using a flow cytometer or a standard fluorescence microscope.
Another approach that has been successfully implemented to assess sperm DNA breakage is based upon the enzymatic addition of labelled nucleotides to the ends of pre-existing DNA breaks. These techniques include terminal deoxynucleotidyltransferase (TdT)-mediated nick-end labelling (TUNEL), or in situ nick translation (ISNT), using E. coli DNA polymerase (Sharma et al. 2010; Ruvolo et al. 2013). In addition, the comet assay consists of performing single-cell gel electrophoresis on selected cells embedded in microgels (i.e. thin layers of agarose supported by glass). Because of the differential resistance encountered by DNA molecules of different sizes when moving through the gel, a characteristic “comet” distribution is formed after fluorescent staining, with a dense head containing long molecules of DNA and a tail of varying length formed from the shorter fragments. The comet assay can be performed under neutral or alkaline conditions, allowing for the identification of ds-DB or ss-DB respectively. DNA breakage can be evaluated by measuring the number of cells with “comet” tails, as well as the length of the tail and/or percentage of DNA actually contained in the tail (Simon and Carrell 2013). A modification of this technique based on a two dimensional displacement of the DNA fragments offers the possibility of differentiating ds-DB and ss-DB on the same nuclei (Fernández et al. 2001) and has been performed on koala spermatozoa (Zee et al. 2009b).
In our laboratories we have focused on the sperm chromatin dispersion (SCD) test. Improved commercial versions of this test are now available for use in humans and domestic species, and these methodologies can be adapted to a range of wildlife species once the assay has been validated. The SCD is a fast, easy to use method of assessing sperm DNA damage and is based on a controlled DNA denaturation and parallel protein depletion performed on spermatozoa that have been trapped within a microgel. The procedure gives rise to haloes of dispersed chromatin due to the spreading of nuclear DNA loops and/or fragments of DNA; when the sperm nucleus contains fragmented DNA, the size of the halo is directly proportional to the amount of sperm DNA damage. Other methodologies based on the use of specific antibodies against a modified DNA molecule, such as presence of single strand DNA motifs (Zhang et al. 2007), presence of 8-hydroxy-G (Santiso et al. 2010), or indirect markers related to apoptotic processes, are also effective for targeting sperm DNA damage, although at this stage of their development they still have technical and cost constraints for routine use.
While there are some minor differences among the various SDF values produced by the different techniques, in general, the correlation among them is high (Chohan et al. 2006). Despite this agreement, there is nevertheless an unfortunate level of sterile debate on the relative merits of each technique and their respective potential to measure “real” or “potential” sperm DNA damage (Álvarez 2005; Makhlouf and Niederberger 2006; Fernández et al. 2008). It is our view, that such arguments are doctrinaire and fundamentally missing the point when trying to critique the intricate nuances of each technique; rather, they should be utilising the inherent potential differences of each approach to solve the problem.
In terms of selecting the most appropriate technique for the assessment of SDF in wild species, there at least needs to be recognition of the intraspecific differences between sperm structure and chromatin chemistry; for example, it is simply naïve to apply the SCSA using the standardised procedures developed in humans when studying other taxa (e.g. marsupialia or avians) without the appropriate validation. Differential chromatin packaging, closely related to the differential cysteine content in protamine residues, may condition DNA denaturation and subsequently the capacity of acridine orange to bind DNA. In fact it is known that the use of dithiothreitol as an—SS—bond reducer has a direct influence on the capacity to incorporate labelled nucleotides after using the terminal transferase for in situ DNA labelling (Mitchell et al. 2011). In addition to species-specific validation of the assay, it is also important to include appropriate internal controls and to be conscious of how the sperm DNA behaves in conditions that mimic sperm transport and storage in the reproductive tract (Holt and Fazeli 2010); or in the case of those that fertilise oocytes externally (fish and teleosts), the surrounding environment.
4 The Use of SDF in ART: Lessons Learned from Human and Domestic Animals
In general, infertile human males have a higher frequency of spermatozoa with fragmented DNA than fertile controls and there is a certain level of correlation between a poor seminogram and a high level of sperm DNA damage (Liu and Liu 2013). Studies performed on sperm samples used for in vitro fertilization (IVF) demonstrate that the frequency of spermatozoa with fragmented DNA in the sample used for fertilization can influence the reproductive outcome (Bungum et al. 2004). A prospective multi-centre survey analysing 729 couples provided evidence of a correlation between a high incidence of spermatozoa with fragmented DNA in the sample used for insemination and low fertilization rate, poor embryo and blastocyst quality and implantation rate (Vélez de la Calle et al. 2008). However, in other cases, this correlation is not so clearly established and no consistent relationship between sperm DNA damage and embryo quality and/or development can be detected.
The influence of sperm DNA damage on human embryo quality/development tends to be more significant in ICSI compared to IVF cycles (Zini et al. 2011). One of the possible explanations for some of these discrepancies could be related to the selection of spermatozoa for fertilization and embryos for transfer. Only the most viable spermatozoa selected by visual inspection and those embryos presenting the best prognosis for implantation are normally transferred. While it is likely that the influence of sperm DNA fragmentation on pregnancy would be more demonstrable if all produced embryos were transferred (regardless of quality) such a scenario can only ethically be achieved in animal models. While a large systematic review and meta-analysis of clinical data revealed a small but statistically significant association between sperm DNA integrity and pregnancy following IVF and ICSI cycles (Collins et al. 2008), the complexity of this relationship is no doubt related to the fact that the correlations in these data analysis are not consistent.
In an attempt to try and disentangle this relationship, we have been turning to the use of proven donor oocytes to minimise female factor infertility and thereby provide new insights about the impact of SDF. In a recent study, we reported results obtained in 70 couples assessed for SDF and sperm motility at the time of sperm injection where the sperm samples were assessed and processed for ICSI at the same time (Nuñez-Calonge et al. 2012). In this experimental model, there was no difference in the fertilization rate, cleavage rate or embryo quality, between pregnant and non-pregnant couples. However, the rate of SDF of non-pregnant couples was around 23.9 % and was higher than in those couples who achieved a pregnancy (SDF = 17.0 %; P = 0.002). The threshold SDF value obtained in this experiment was 17 % and this value could be used to predict pregnancy with a sensitivity and specificity of 78 % and 71 % respectively (Nuñez-Calonge et al. 2012).
The assessment of sperm DNA integrity appears to be an important adjunct to the determination of sperm quality, providing relevant information, not only for reproductive outcomes but for other andrological pathologies. For example, in testicular cancer, the level of DNA damage is very high (Meseguer et al. 2008; Romerius et al. 2010). In these cases, assessment of the level of SDF can provide valuable information about the potential use of cryopreserved sperm samples prior to chemo- or radio-therapy, so that sperm samples from these patients can be cryopreserved for use post-treatment. Patients with a varicocele usually present with an increased level of baseline SDF; two different levels of affected spermatozoa with sperm DNA fragmentation are easily recognized (Enciso et al. 2006; García-Peiró et al. 2012) and fine control of fluctuations in the incidence of these subpopulations can be used to gain information about the effects of antioxidant treatments on final sperm quality (Abad et al. 2012). Urogenital infections produced by Chlamydia or Mycoplasma infections cause an increased in level of SDF in the ejaculate, but these can be ameliorated using antibiotics at higher doses than those usually recommended (Gallegos et al. 2008). The koala is also known to suffer from chlamydiosis and we are currently examining whether this organism has a similar effect on sperm DNA. The assessment of SDF might also be of relevance in couples with systematic ART failure, or in those cases where the female partner shows poor prognostic conditions such as low ovarian response to stimulation, implantation failure or poor embryo quality; advanced age is also one of the causes that must be taken into account, since the sperm DNA repair capacity of the oocyte in these cases may be compromised.
Associated with strong selection pressure for reproductive performance, a strong correlation between a high level of sperm DNA fragmentation and pregnancy has been established. This is particularly the case for boar or bull (Rybar et al. 2004; Kasimanickam et al. 2006; Fatehi et al. 2006). Strong selection pressure on reproductive performance is likely to be resulting in males with ever decreasing levels of SDF. The most parsimonious hypothesis would be to assume that SDF would have a neutral effect on reproductive outcome such that the distribution of SDF in the population is variable (low and high values), even in those species that are selected for reproductive success. Surprisingly, we actually found this not to be the case, and in those species selected for reproductive characteristics, such as boar, bull or ram, the SDF index was relatively low (López-Fernández et al. 2008a, b; Gosálvez et al. 2011a). Landrace-Large White breed boars, Holstein bulls and Assaf rams, showed values ranging between 0.5 and 10 %, 4.4 % and 4.1 % and 4.1 respectively (Fig. 15.2). Conversely, species that have not been heavily selected for reproductive characteristics, such as human, stallion or donkey, show much more variable values for SDF at 15.8 %, 16.1 % and 16.6 % respectively (Fig. 15.2). This concept obviously has direct relevance for wild populations as the males of rare or endangered, or genetically restricted, populations are purposely often outbred to maintain as much genetic diversity as possible, without any attention to reproductive performance; one would therefore, predict much larger variation of SDF in wild males.
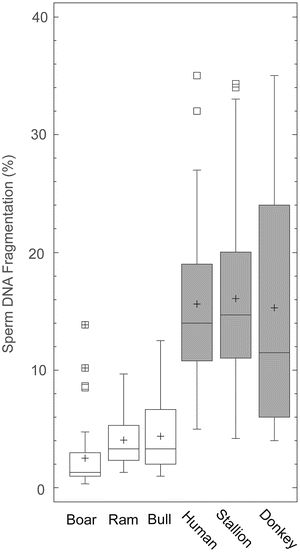
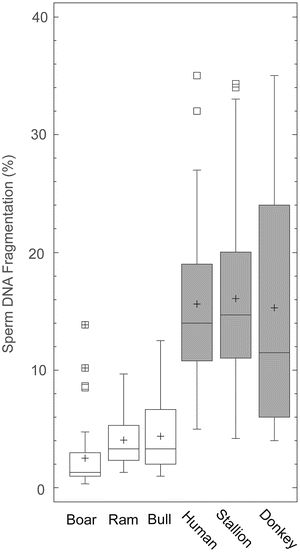
Fig. 15.2
Descriptive statistics for the sperm DNA fragmentation level in two groups of species; in group 1 (white bars) males of each species are from populations selected for ART based on reproductive characteristics (boar, ram and bull) whereas those in group 2 (grey bars) are from non-selected populations (human, stallion and donkeys)
Variable quality in the intra- and inter-specific production of spermatozoa containing a fragmented DNA molecule presents several interesting points that are relevant to the assessment of SDF in wild species. The ejaculates of all species contain spermatozoa with damaged DNA, so that one would perhaps expect continuing directional selection for an important fitness character such as sperm quality, as this result suggests. Further to this, the level of SDF in species selected by man for reproductive purposes is generally lower than those selected for other characteristics. In the case of the boar or ram, all of the animals belong to a random sample from genetic resource centres, where the best animals for particular characteristics demanded by the market are selected. However, all of them also share a common characteristic in that they must be reproductively efficient. For whilst these animals are not deliberately selected by the ART centres to produce low levels of sperm DNA damage in their ejaculate, this is the unintended consequence. This is indicative of stronger selection pressure (whether applied naturally or by human intervention) empirically favouring the presence of spermatozoa with high quality DNA. This mirrors the situation with other sperm parameters where there is considerable evidence that post-copulatory sperm competition is associated with the evolution of sperm features that optimise sperm head and flagellar characteristics and enhance swimming ability (for review, see Birkhead and Immler 2007).
5 The Relevance of Sperm DNA Fragmentation for Wildlife Management and Reproductive Technologies
Environmental destruction, loss of the ecological connectivity, climate change, invasive species or direct predation and hunting are all compromising the survival and conservation of a large number of mammalian species. The Iberian lynx (Lynx pardinus) is a wild felid native to the Iberian Peninsula in Southern Europe and is one of the most critically endangered species currently known to man; it is also likely to be the first felid species to become extinct since prehistoric times. Problems emerging from habitat fragmentation and a dependence on rabbits as their primary food prey species, has been a primary driver for population decline in European felids.
The Mohor Gazelle (Nager dama) were once running free in north Africa during the 1930s but are now confined to purpose built ex situ captive breeding facilities; hunting was a primary cause of extinction in this species (Fig. 15.3). ART has been presented as adjunct discipline to help overcome some of the genetic issues that are likely to arise from small isolated populations (Abaigar and Holt 2001) and as tools to support efforts to reintroduce the species to its original range (Cano et al. 1993).
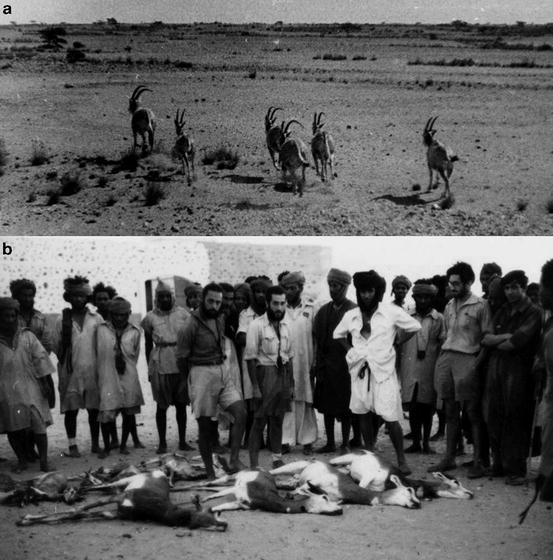
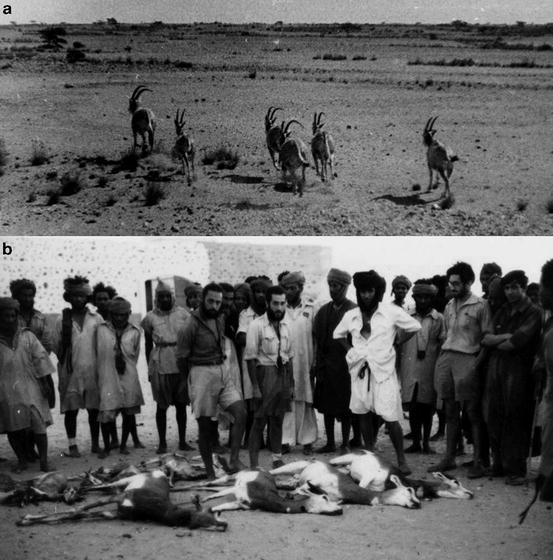
Fig. 15.3
Endangered bovids of Northern Africa (a) One of the last free white Oryx herds (Oryx dammah) running free in El Krat flats in Western Sahara during the 1940s. (b) Indiscriminate hunting of Mohor Gazelles in Tichla-Western Sahara in 1943. Photos courtesy of Prof. José Luís Viejo of Universidad Autónoma de Madrid from the E. Morales-Agacino archives; the original photographs were taken by Dr. Eugenio Morales Agacino during the Sahara campaigns (1943–1956)
Gamete cryopreservation is one of the most challenging strategies to protect and propagate the species but it also allows precise genetic management both in time and space (Holt et al. 1996; Holt and Moore 1988; Watson and Holt 2001). Many frozen-thawed samples of different individuals and of different species can be stored in the one liquid nitrogen container; this reduces the cost and inconvenience of keeping animals and provides the genetic manager with an opportunity to extend the generation interval of important sires (for review see Holt et al. 2003). The shipment of genetics between populations (zoos or isolated fragments) is also greatly facilitated with the use of frozen semen and leads to a reduction in the animal welfare issues associated with genetic exchange.
Compared to the oocyte, the spermatozoon is a more specialised and differentiated cell, designed to leave and exist in isolation from the soma for hours; consequently the DNA is very well protected and packaged for this exposure. Interestingly, there is large variation amongst different species with respect to viability and DNA integrity when the spermatozoa are handled ex vivo (Gosálvez et al. 2011b). This is partly related to the reproductive strategies of the species, especially with respect to whether it utilises external or internal fertilisation. In external fertilisers such as fish and amphibians the spermatozoa begin activation once exposed to the external environment and remain viable for only minutes. One might expect the DNA integrity of these species to decline rapidly and this has been shown to be the case in teleost fish (López-Fernández et al. 2009), but preliminary results on amphibian (Xenopus sp.) spermatozoa in our laboratory indicate that the sperm DNA remains intact for hours after activation, even after cryopreservation. At the other end of the spectrum, boar or bull sperm DNA is able to remain in the reproductive tract for hours or even days (Holt and Lloyd. 2010; Holt 2011) because specific mechanisms (disulphide bonding between cysteine residues) help to stabilise the DNA. This picture is somewhat complicated by observations on marsupials such as the koala, which do not possess cysteine in the protamines, but are still able to show that DNA fragmentation can remain low following incubation or cryopreservation (Johnston et al. 2012a). Clearly these species have evolved a different mechanism to retain DNA integrity for internal fertilisation. Some avian and reptilian species are known to be able to store sperm in the reproductive tract for long periods of time; in the case of some Squamata and Chelonian reptiles sperm storage has been reported to occur for up 5 years (Birkhead and Moller 1993). Given that these internally fertilising species possess no cysteine in their protamines, it would be fascinating to explore how the sperm DNA has been packaged with respect to the protamines in order to provide such stable DNA integrity. The specifics of how sperm DNA responds once it enters the external environment or the female reproductive tract, has practical application with respect to selecting the best species-specific techniques for sperm preservation and for designing quasi-species specific seminal extenders to provide the spermatozoa with the best media for optimal survival.
Since the first recognized attempt at sperm preservation by Spallanzani (1776), there have been many improvements in preservation technology, including new formulations for semen extenders with various empirical combinations of cryoprotectants, addition of molecules to stabilize membranes, antibiotics to avoid bacterial growth, antioxidant molecules and a range of sophisticated freezing protocols (see reviews by Hammerstedt et al. 1990; Foote 1998; Foote and Parks 1993; Maxwell and Salamon 1993; Watson 1995; Royere et al. 1996; Holt 1997; Vishwanath and Shannon 1997; Woelders 1997). More recently, these developments have been coupled with new technologies for individual sperm selection and injection (ICSI) that in essence do not even require a viable spermatozoon to produce a fertilised embryo. Yoshida (2000) has identified a series of inputs where sperm cryopreservation may have a major impact in wildlife conservation including (1) direct conservation and propagation of threatened or endangered species, (2) international exchange for import-exporting genetic lines, (3) conservation of manipulated sperm for gender selection, (4) conservation of genetic lines having superior genetic traits, (5) rare breed or transgenic lines for establishment of genetic resource banks and (6) gamete reserve to be supplied in response to disease-disaster. Johnston and Holt (2013) have suggested that genome banks can also be established from gametes recovered from post-mortem or wildlife destined for euthanasia. There is also the possibility of recovering gametes from diseased animals and treating or cleaning up these cells prior to cryo-banking (Bielanski 2007; and Bielanski Chap. 17, this book; Bostan et al. 2008). Understanding how sperm DNA integrity is affected by the cryopreservation procedure and maintained following thawing and incubation are important considerations when developing protocols for wild species.
6 Case Study 1: The Rhinoceros
Of the five extant rhinoceros species, the International Union for Conservation of Nature (IUCN) Red List of Threatened Species lists three species as critically endangered, the Javan rhinoceros (Rhinoceros sondaicus), the Sumatran rhinoceros (Dicerorhinus sumatrensis), and the black rhinoceros (Diceros bicornis). Another, the greater one-horned rhinoceros (Rhinoceros unicornis) is catalogued as endangered and the white rhinoceros (Ceratotherium simum) is threatened. Ex situ reproductive management, including assisted breeding, offers substantial advantages to the conservation of captive rhinoceros populations but the current breeding programs are unfortunately characterized by a low level of reproductive success. Despite investigations into some of the underlying causes of female rhinoceros infertility (Brown et al. 2001; Hermes et al. 2005), there has been comparatively little consideration given to male infertility. Sperm DNA quality has thus far only been assessed in six different animals using cryopreserved semen samples (Portas et al. 2009). The results showed that the baseline level of SDF was relatively low, but that DNA quality rapidly started to decline after only 4 h ex vivo incubation at 37 °C. After 24 h of sperm incubation at 37 °C, SCD of the spermatozoa show large haloes of dispersed chromatin, revealing massive sperm DNA damage (Fig. 15.4). Freshly collected spermatozoa incubated under similar conditions showed no increase in the basal level of DNA fragmentation for up to 48 h. Clearly, cryopreservation of rhinoceros spermatozoa leads to increased levels of sperm DNA fragmentation, either because of an inherent sensitivity of the cell to cryopreservation or an inappropriate cryopreservation procedure. In addition, we have also observed another peculiar condition of the sperm DNA damage in the rhinoceros that is not common in other species; this is the production of double stranded DNA damage after sperm incubation; comet assays of rhinoceros spermatozoa clearly show small comet tails produced under neutral electrophoretic conditions emerging from a compact core (Fig. 15.4c, d). We have not yet investigated this aspect in any detail but certainly the presence of double strand breaks in the sperm DNA is highly unlikely to be repaired by the oocyte, and would lead to embryonic loss or pregnancy failure.
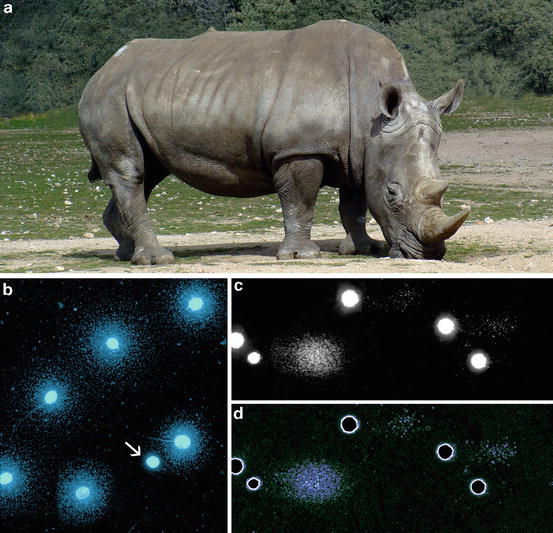
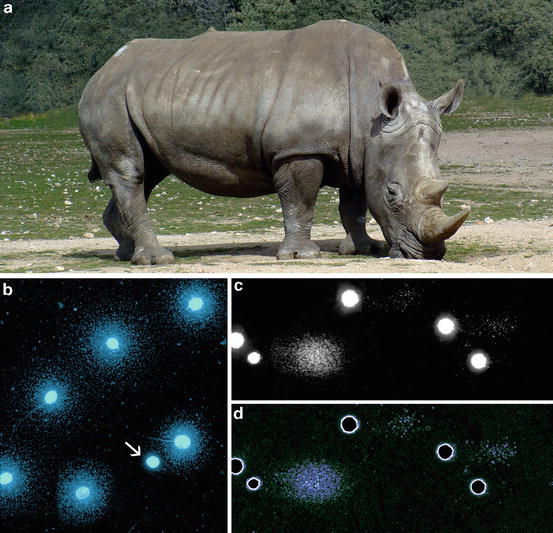
Fig. 15.4
(a) White rhinoceros (Ceratotherium simum) and visualization of sperm DNA damage using the sperm chromatin dispersion test. (b) Spermatozoa showing a large halo of dispersed chromatin are those containing a fragmented DNA molecule while the compact sperm head is regarded as normal. (c) Visualization of DNA damage using a comet assay test illustrates different levels of chromatin damage according to the size of the comet. (d) Electronically filtered image of (c) to enhance comet visualization
The high incidence of sperm DNA fragmentation following cryopreservation in the rhinoceros provides us an opportunity to show how we could use sperm DNA fragmentation analysis to help us elucidate a more appropriate cryopreservation protocol. The hypothesis to be tested in this investigation would be that the lower the rate of SDF, the better the quality of the frozen-thawed spermatozoa. This model is very simple and could be used to analyze the differential negative impacts of different experimental conditions in maintaining in all sperm characteristics ex vivo. We have exploited this model primarily to assess sperm DNA survival (Gosálvez et al. 2009; Gosálvez et al. 2011b), but it is an analogous to the thermal stress tests (Fiser et al. 1991) used to analyze the dynamic loss of the sperm plasma membrane. Using different experimental conditions (Fig. 15.5), for example, the same sperm sample extended in different diluents, we could calculate and compare the final rate of DNA fragmentation (r-SDF) between these treatments after a series of hours of sperm incubation at 37 °C; in the model depicted in Fig. 15.5, we have proposed 12 h of sperm incubation, but this may vary between species. Based on the dynamic behaviour of SDF observed in Fig. 15.5 we could conclude that treatment 1 is the most beneficial for maintaining sperm DNA integrity, since the rate of SDF (r-SDF) is only 0.25 % per hour, whereas in treatment 3 the r-SDF in an equivalent aliquot is a much higher 4.6 % per hour at equivalent incubation times. Similarly, this experimental model could also be used to analyse the impact of different preservation temperatures for liquid storage and or transportation.
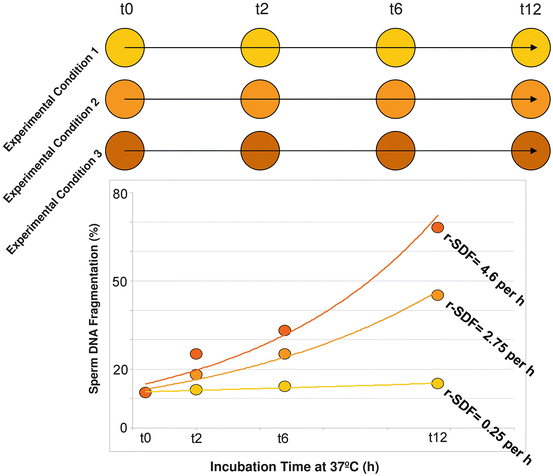
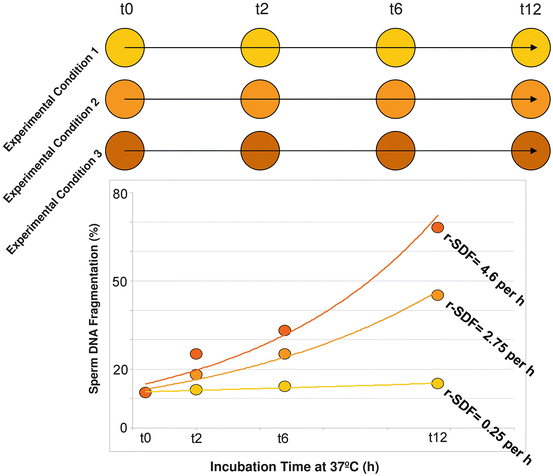
Fig. 15.5
An experimental model to show how the dynamic assessment of sperm DNA damage reveals differences in the cryptic DNA damage, which are not observed after testing for DNA damage values at t0. While the levels of SDF are similar after assessment at t0, these values vary differentially as the incubation time increases, giving rise to different rates of sperm DNA fragmentation (r-SDF)
Prior to chilled preservation and transport, the sperm concentration of the inseminate is typically adjusted upwards to account for loss of sperm viability over time. However, we have recently conducted studies on ram spermatozoa that were somewhat counter intuitive to this strategy, and which showed that higher sperm concentration could actually result in a corresponding increase in SDF (López-Fernández et al. 2010). The dynamic assessment of SDF showed that the r-SDF were not only dependent on the inherent sperm DNA fragmentation expressed immediately after thawing, but also on the sperm concentration within the incubated sample. The application of this same model to test the impact of different DNA stressors on human spermatozoa also showed that DNA fragmentation dynamics could be used to assess ‘cryptic’ sperm damage (Santiso et al. 2012); in this study, increasing acute doses of elevated temperature (41–45 °C), acidic pH and nitric oxide exposure, all resulted in accelerated SDF kinetics following chronic exposure over a 24 h period.
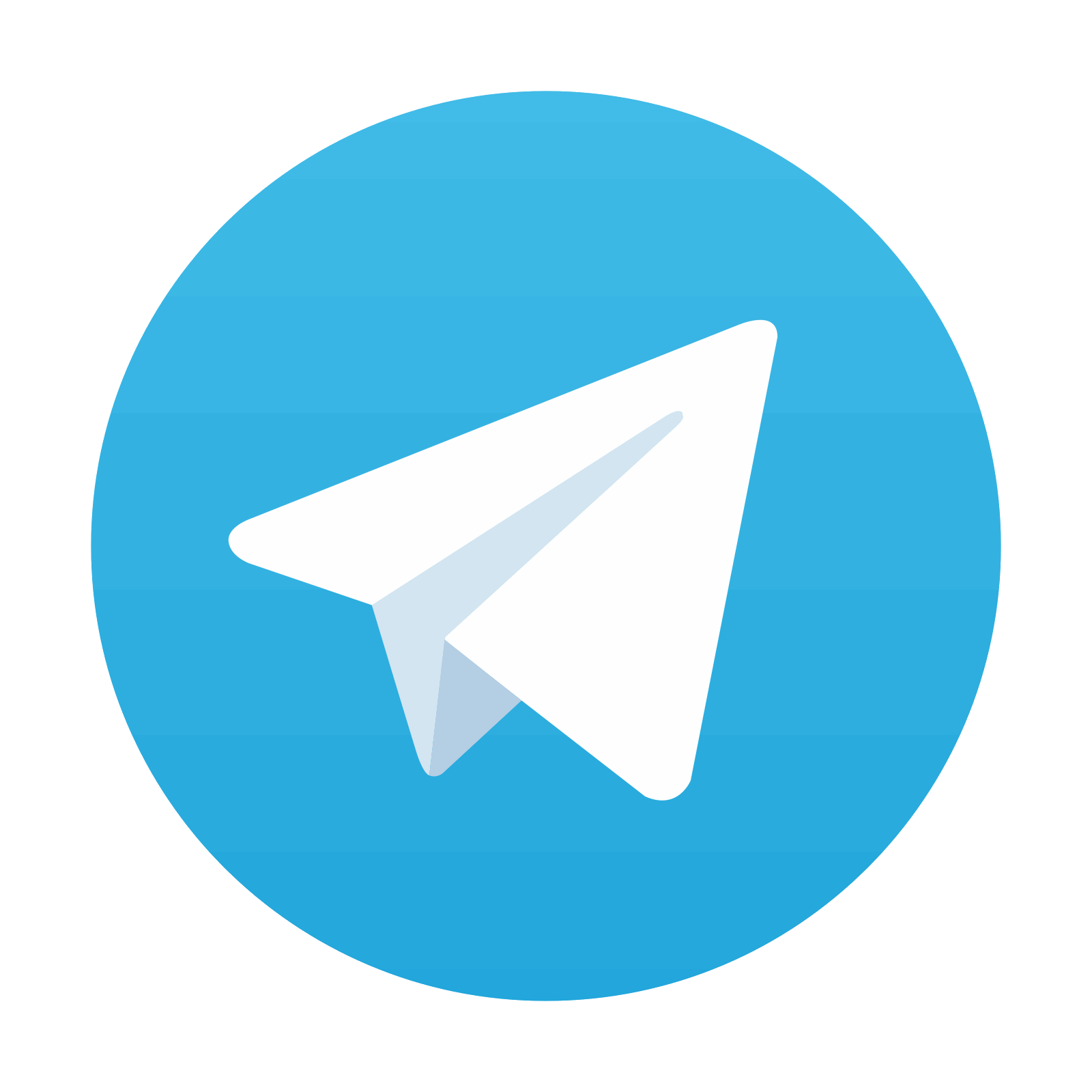
Stay updated, free articles. Join our Telegram channel

Full access? Get Clinical Tree
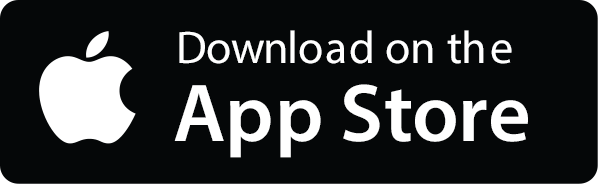
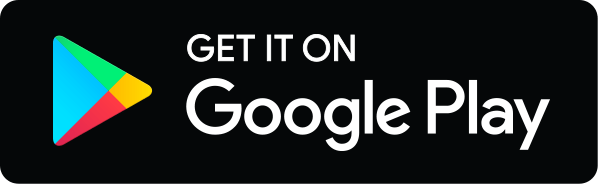