Fig. 2.1
Sources of fluoride toxicity in animals
2.1 Natural Sources
Fluorosis in animals can occur due to high fluoride concentration naturally occurring in dietary substances including feed, fodder, concentrate ration, and drinking water.
2.1.1 Forage, Grasses, and Grains
Soil fluoride concentration is believed to have little influence over fluoride concentration in vegetation, inasmuch as most of the fluoride in soils cannot be assimilated readily by plants. However, a direct relation is reported to exist between F concentration in the soil and selected forage. Fluoride in forage increases by 3 ppm for each 100 ppm increase in soil F up to approximately 2200 ppm (Mascola et al. 1974). Other factors modulating fluoride concentration in fodder plants include height of the plant and season. Fluoride content increases with decrease in plant height. The fluoride concentration is higher in plants grown during winter and spring in comparison to those grown during summer and autumn (Mascola et al. 1974). Fluoride uptake by plant roots from soil occurs by passive diffusion, and thereafter F is carried to the shoot by transpiration. Most of the plants do not accumulate fluoride in toxic concentrations and the level usually remains below 10 mg per kg dry weight. The tea plants (family Theaceae) can exceptionally accumulate high F concentrations. However, tea plants or their by-products are not a usual ingredient of animal feed. Many plant species, particularly Acacia georginae and Dichapetalum cymosum (a South African shrub known as “Gifblaar”) can assimilate soil F and convert them into fluoroacetate, which is extremely toxic for animals (Shupe et al. 1984). Fluoroaceate is converted in vivo in mitochondria into fluorocitrate through condensation of fluoroacetyl-Co-A with oxaloacetate by the enzyme citrate synthetase which normally supplies acetyl-Co-A into the citric acid cycle. Fluorocitrate is a strong inhibitor of aconitase, which is an important enzyme of the Krebs cycle. Thus fluorocitrate inhibits the biochemical pathway for energy production in the organism (Patocka and Strunecka 2002).
It is interesting to note that gaseous fluoride compounds can enter leaves through stomatal pores and in high concentration they may induce toxicity signs in plants, including chlorosis, peripheral necrosis, leaf distortion and malformation, and abnormal fruit development. Long-term exposure to F concentrations greater than 0.2 μg/m3 may cause injury to plants (WHO 1984). It has been observed that air-contaminated forage loses a portion of its fluorine to the atmosphere after cutting and during storage as hay (NRC 1960).
The mean fluoride concentration of mineral soils is around 0.2–0.3 g/kg, whereas that of organic soil is usually lower. The fluoride content of topsoil increases by the addition of fluoride containing phosphate fertilizers, pesticides, and irrigation water or by deposition of gaseous and particulate emissions from industry. In New Zealand, it was found that long-term use of phosphorus fertilizers resulted in an increase in total surface soil (0–75 mm depth) fluoride concentration up to 217–454 mg per kg (Loganathan et al. 2001). Fluoride uptake by grazing animals through plant consumption is expected to be much lower than F uptake directly by soil ingestion because most plants do not accumulate F. Therefore, reducing soil ingestion by maintaining good pasture cover can reduce the risk of fluorosis in herbivores (Loganathan et al. 2008). Forage and grasses grown in industrial areas are often contaminated by fluoride-rich industrial effluents or by windblown or rain-splashed soil having a high fluoride concentration. Most industrial fluoride emissions are airborne and fluoride-rich dust, ash, and fumes may contaminate soil, water, and vegetation not only in the industrial vicinity, but also up to a considerable distance from the source of emission (Radostits et al. 2000). In such conditions F concentration in plants depends upon (1) the amount of fluorine released into the atmosphere, (2) the distance between the F emission source and the pasture area, (3) the type of vegetation, and (4) the distribution pattern as affected by the wind and topography (NRC 1960). Plants grown in areas where F is emitted into the atmosphere may contain 500–1000 ppm fluoride. Hence, animals should not be allowed to graze in pasture close to industries such as brick-kiln plants, rock phosphate processing plants, aluminum industry, and so on. Fodder plants grown in polluted areas should be harvested, soaked, and washed with water before offering to animals. This practice will substantially reduce the available F to animals.
Grains usually do not contain toxic concentrations of fluoride. Corn, wheat, oats, and barley grown on F-rich soil have very low F concentrations (less than 10 ppm) and nearly equal those grown on soil low in F (NRC 1960). Nevertheless, sorghum (Sorghum bicolor) consumption in human beings has been found to increase the risk and severity of osteo and dental fluorosis (Krishnamachari and Krishnaswamy 1973; Hari-Kumar et al. 2007). Sorghum grown in fluorotic areas contains a high molybdenum concentration. Molybdenum reduces urinary fluoride excretion and enhances fluoride retention (Stookey and Muhler 1962). A sorghum-based diet therefore increases F retention in human beings (Lakshmaiah and Srikantia 1977) and rats (Lakshmi and Lakshmaiah 1999). It is surprising to note that sorghum plants are more susceptible to hydrogen fluoride gas exposure than wheat plants (Mac-Lean et al. 1984).
2.1.2 Water
The fluoride concentration of natural groundwater depends upon geological factors, consistency of the soil, porosity of rocks, pH and temperature of the soil, complexing action of other elements, depth of wells, leakage of shallow groundwater, and chemical and physical characteristics of water (Li et al. 2014). Both surface and groundwater may have high F concentration in a particular locality, but the level is often higher in groundwater than the surface water. When groundwater percolates through rocks containing fluoride-rich compounds, fluoride leaches out and concentration may increase far above the safe level. The mean fluoride content of rocks varies between 0.1 and 1.0 g/kg. Important fluoride-containing minerals are fluorspar, cryolite, and apatite. However, in most soils, F is associated with micas and other clay minerals that make them less soluble and hence less toxic. Sodium fluoride and magnesium fluoride are also found as natural minerals. Fluoride contamination occurs through a natural process in which fluoride-bearing rocks crumble and break down, but the process can be speeded up if the chemistry of the aquifer is disturbed. Climatic conditions may also influence rate and degree of fluoride dissolution in water from rocks and soil. High F concentration in shallow zone groundwater is largely due to the geochemical F deposition in the vicinity of the groundwater extraction structures. The toxicity potential of fluoride-rich water is also influenced by the ambient temperature; alkalinity; and calcium, copper, and magnesium concentrations in water.
Water having a fluoride concentration up to 1.0 mg/L is safe, levels in between 1.1 and 2.5 mg/L are marginally contaminated, whereas above 2.6 mg/L are considered highly contaminated and dangerous for drinking purposes for human beings (Susheela 1999). It is estimated that total fluoride intake by a person when potable water contains 1 ppm fluoride would be from 0.05 to 0.10 mg per kg of body weight per day (NRC 1960). Most terrestrial animals, particularly domestic herbivores, are supposed to tolerate F in water higher than these threshold levels. However, many reports indicate the appearance of toxic symptoms in cattle, buffalo, and other livestock reared in areas where mean water fluoride concentration is equal to or slightly above 1.5 ppm (Choubisa 1999; Maiti et al. 2003; Ranjan et al. 2009).
2.1.3 Volcanic Activities
Domestic and wild animals often suffer from severe fluorosis by ingestion of water and plants contaminated with volcanic ash (Araya et al. 1990; Bellomo et al. 2007). Volcanic ash usually has a very high soluble fluoride concentration and can be deposited over a large geographical area. In volcanic areas, the fluoride concentrations in water and pasture grass may remain high for years even after cessation of volcanic activities (Araya et al. 1993). The fluoride concentration in volcanic ash emitted by the Hekla volcano, Iceland, was up to 2000 ppm and the forage covered by ash had fluoride concentrations of 350–4300 µg/g (Thorarinsson 1979). In Chile in 1988, fluoride-bearing ash arising from the Lonquimay volcano affected more than 10,000 farm animals and resulted in the death of more than 4000 goats, sheep, cattle, and horses (SEAN 1989). Likewise, the death of thousands of sheep in 1995–1996 was attributed to ashfall from the Ruapehu volcano eruptions in Mexico (Armienta et al. 2011). In Argentina, the ash (“tephra”) released from the Puyehue–Cordon Caulle volcanic eruption in June 2011 reached up to a distance of 1400 km. Severe fluorotic dental lesions were observed in wild red deer who died after the volcanic eruption, which was attributed to consumption of grasses contaminated with volcanic ash (Flueck and Smith-Flueck 2013). In Iceland, thousands of sheep, cattle, horses, and other domestic and wild animals died due to repeated volcanic eruptions over the years (Armienta et al. 2011). Occasionally, acute toxicity in animals may occur after inhalation of hydrogen fluoride released from volcanoes (Weinstein and Davison 2004).
2.2 Anthropogenic Sources
Anthropogenic sources are also responsible for fluoride toxicity in animals. Some of them have been described in this chapter.
2.2.1 Mineral Mixture and Other Feed Supplements
There are several reports documenting mineral supplements as a major source of fluoride toxicity in livestock (Griffith-Jones 1977; Hillman et al. 1979; Singh and Swarup 1995). In a cattle herd numbering 120 animals, introduction of a commercial salt lick with fluoride concentration 1400 mg per kg resulted in the development of fluorotic lesions after one year. The fluoride concentrations in water and pasture samples were within the normal range suggesting the salt lick as the sole source of excess fluoride intake (Schultheiss and Godley 1995). In northern Australia, up to 15 % of cattle in a herd revealed signs of lameness due to use of fertilizer-grade monoammonium and diammonium phosphate as a mineral supplement. The affected animals were given large quantities of the mineral supplement thinking that the lameness was due to a phosphorus deficiency as it was an endemic problem in that area (Jubb et al. 1993). Chronic ingestion of gypsum that was included in a feed supplement and also ingested from fertilizer dumps in paddocks resulted in the death of 226 cattle at a farm over a period of 18 months. Here, direct toxicity of gypsum as well as the effect of excess fluoride was incriminated as the cause of heavy mortality in the cattle herd (Bourke and Ottaway 1998).
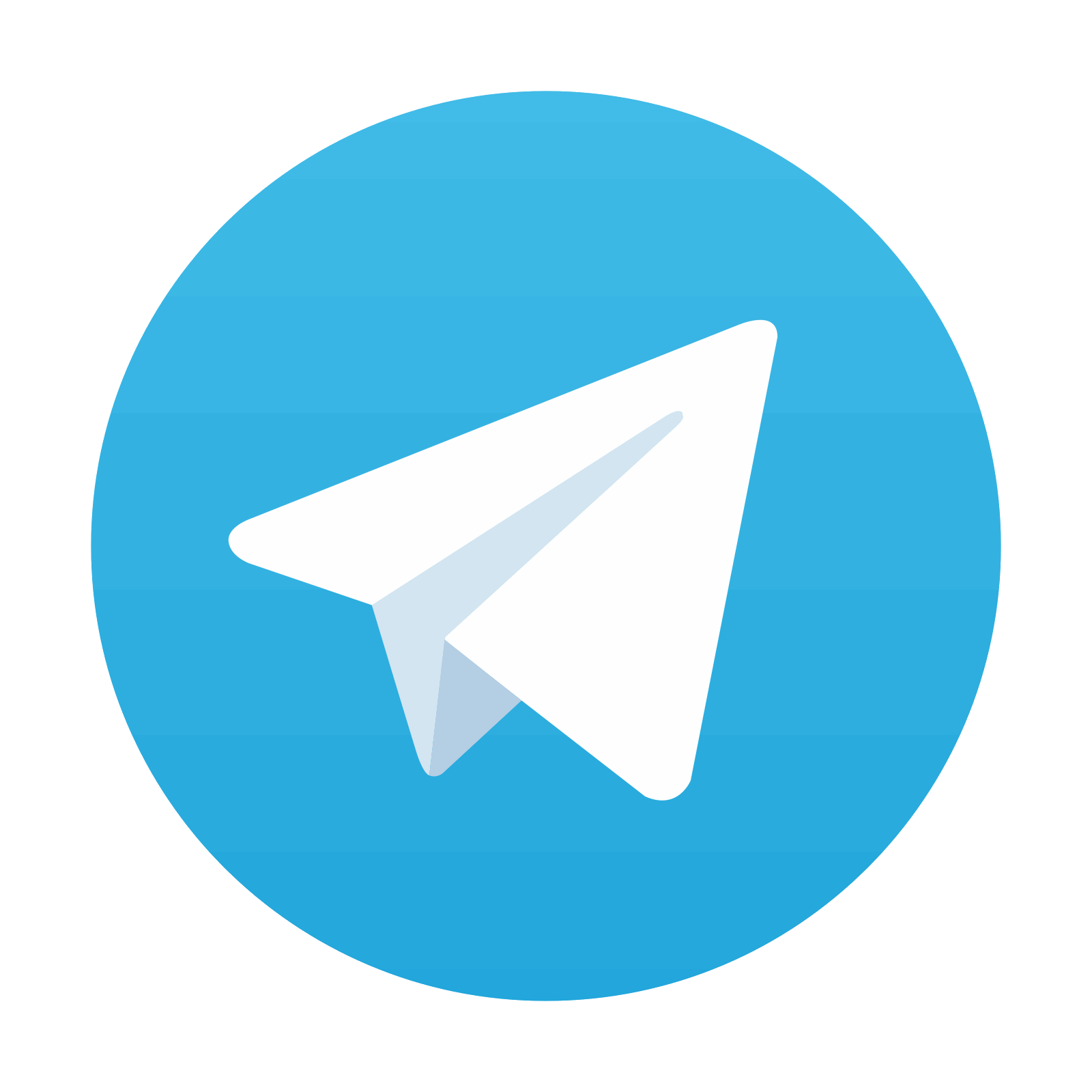
Stay updated, free articles. Join our Telegram channel

Full access? Get Clinical Tree
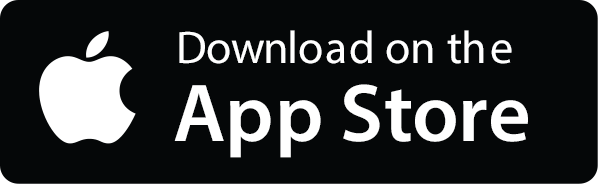
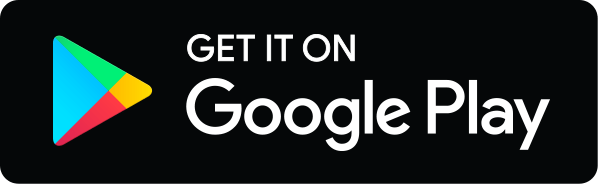