19 Semen Evaluation and Handling: Emerging Techniques and Future Development Heriberto Rodriguez-Martinez* The assessment of male reproductive health (clinical andrology), including the examination of the male genital organs, the ability to mate and the analysis of collected semen, is pivotal to the establishment of artificial insemination (AI) and the selection of breeding sires (Foote, 1999). Laboratory semen assessment has become, along with the selection of the most suitable males (for productivity and fertility), more and more important for the diagnostics of semen processing steps – from sperm cryopreservation to sperm sorting for chromosomal sex. For unselected animals, semen assessment was the only means to determine whether a male was potentially fertile or not. In either case, we have experienced an explosive development of in vitro assays to determine sperm intactness and to measure sperm function. Despite this availability of multiple assays, conventional semen evaluation is still often restricted to determinations of sperm numbers, sperm motility and, sometimes, but rather rarely, sperm morphology. Those few sperm parameters seem sufficient, at least under commercial conditions, to disclose the degree of ‘normality’ of the testes (sperm numbers, morphology) and the epididymides (sperm morphology, motility). Underlying this approach is the fundamental axiom that an ejaculate must contain above a certain number of motile, morphologically ‘normal’ spermatozoa in order to allow minimum sperm numbers to reach the oviducts for eventual participation in the complex process of fertilization, and finally lead to the safe development of a conceptus. This axiom is further reinforced by the increasing belief that, if sperm numbers were high, and despite the presence of morphological abnormalities, the female genital tract is able to select spermatozoa by morphology and, further, that motility is pivotal both in this selection and in the interaction with the female genitalia and the counterpart gamete (Holt and Van Look, 2004; Rodriguez-Martinez et al., 2005; Rodriguez-Martinez, 2007a; Holt, 2009, 2011; Holt and Lloyd, 2010). However, more and more methods are now available for semen evaluation that not only make it possible to disclose the level of ‘normality’ of the male genital organs, but also the capability of spermatozoa (mostly their membranes but also their metabolomics) to interact with the surrounding fluids (the seminal plasma, or SP, female genital fluids and also in vitro culture media), cells (epithelia, cumulus cells, oocytes) or extracellular material (the hyaluronan coating and the zona pellucida, or ZP) before fertilization. Methods are also available to disclose the status of the different organelles, and the integrity of the nuclear genome and of the available transcriptome, which are all related to the capability to initiate early embryo development. Although some of these methods, particularly those of an ‘‘omic’ nature, are still restricted to the research bench, the accompanying development of relevant instruments, from computer assisted sperm analysers for motility (CASA, computer assisted sperm analysis for motility) or morphology (ASMA, computer assisted sperm morphometry analysis) to bench-model flow cytometers (FCs) are making assays accessible for clinical diagnostics and for semen processing for use in assisted reproduction. The reader is advised to look at some of the many reviews that have become available over the past decade, and the references therein, which cover most of the developments made in the past second half of the last century (e.g. Rodriguez-Martinez and Larsson, 1998; Graham, 2001; Katila, 2001; Rodriguez-Martinez, 2003, 2006, 2007b; Parkinson, 2004; Graham and Mocé, 2005; Guillan et al., 2005; Rijsselaere et al., 2005; Petrunkina et al., 2007; Rodriguez-Martinez and Barth, 2007; Mocé and Graham 2008). The relevant questions here are: (i) is current conventional semen evaluation sufficient; and (ii) have we reached a level where current spermatological laboratory methods allow us to disclose the fertility of a male and/or his ejaculate, processed or raw? Conventional analysis of semen is most relevant for the initial investigation of male infertility but, as concluded by this author 10 years ago, only of limited value if prediction of fertility is the aim (Rodriguez-Martinez, 2003). Have we now made sufficient progress towards this goal? What will the situation be in 10 or even 20 more years? The present chapter does not intend to forecast the future, but it does aim to critically review advances in semen assessment methodologies and the capacity that different assays have to prognose fertility (or, perhaps, infertility). Although far from exhaustive, the review attempts to provide a comparative view of the different species for which clinical andrology and modern seminology interact with breeding strategies and the application of assisted reproduction technologies (ARTs). Particular attention is paid to new methods for determining DNA and transcript intactness, as well as to those biomimetic in vitro assays that, by resembling events during sperm transport, storage and interaction with the female genital tract and the oocyte, best provide clues for sperm selection and the role of sperm sub-populations in the ejaculate. Finally, it attempts to identify those techniques that might be available for clinicians and breeders 20 years from now. The clinical andrological examination of a sire, including the screening of his semen, aims not only to determine the normality of the function of his testes and genital tract, but also his capacity to mate. Such an examination provides an andrological diagnosis with a final estimation of his potential capacity to procreate, which, among animal breeders, is often known as a breeding soundness evaluation (BSE). The BSE (as described in accompanying chapters), combines a general clinical examination, a detailed genital evaluation, a testing of the sire’s capacity to mate and laboratory evaluation of the ejaculate(s) (Kastelic and Thundathil, 2008). The BSE, mostly applied to production animal species, has helped us to identify clear-cut cases of infertility, or even of potential sub-fertility, thus facilitating the removal of sub-fertile males from both natural and assisted breeding (AI in particular). For species other than production animals, andrological screening has also proven to be essential, especially when detailed semen evaluation has been included, to determine the causes of substandard semen/sperm quality, and to establish the risk of infertility. As such, it pursues a purely diagnostic purpose (which is the primary objective of clinical andrology), when the restricted possibilities for treatment that we have for most pathologies are considered. Diagnoses of infections (usually of the epididymides and the accessory sexual glands/urethra) are seldom followed by treatment in production animals, mainly because of the cost and relatively low rate of success of such treatments. Such infections are, however, often treated in companion and sport animals in a manner that often mimics human medical treatments. The value of these treatments is, in many respects, questionable. Hormonal treatments of, for instance, cases of primary spermatogenic failure (either diagnosable or idiopathic), are particularly discouraged, both for their relative ineffectiveness and because of their cost in domestic animals. In species that usually have a comparatively ‘less good’ semen picture, for example the feline, canine or equine, semen evaluation is directed towards the identification of whether the problem is related to sperm numbers or sperm dysfunction, as ‘treatment’ opportunities might be present even without hormones. Low sperm numbers in the ejaculate denote a low spermatogenic output, which might be optimized by the establishment of an individual ‘rhythm’ of semen collections, taking care of maintaining sperm maturation thresholds and the best possible sperm output per ‘abstinence’ (or noncollection) period (time elapsed from last collection). Often, this procedure might improve chances of fertility by the implementation of ‘controlled mating intervals’. Assessment of the type of sperm dysfunction that may be present is most relevant here so that the most appropriate type of assisted reproduction technology can be determined, e.g. lowsperm dose AI, in vitro fertilization (IVF) or even intracytoplasmic sperm injection (ICSI), and, if possible, applied. This is relevant for some pet breeds and particularly for horses, where sub-fertility is widespread among stallions and results with conventional IVF are, as yet, suboptimal. The situation is far from similar in animals (mostly production animals) that have been selected for their fertility. Semen evaluation in breeding sires of production species (such as the bovine, ovine or porcine) often goes beyond the primary diagnostic purpose (early diagnosis of subfertility, so that the sire is promptly removed to avoid unnecessary costs), and extends to prognose levels of their ‘expected’ fertility before AI is used and their selection as top sires is decided upon. Thus, early identification and removal of the ‘notso-good’ sires is a priority. Despite this, during clinical andrological examinations, fertilizing capacity is not monitored by a simple spermiogram, and is often only assessed after breeding by natural mating or AI of a certain number of females. The recordings of fertility are, therefore, of utmost importance if a male is to be downgraded, from ‘normal’ fertility output to subfertility or, in serious cases, to permanent infertility (sterility). The registering of male fertility varies in stringency among species, mostly depending on the number of females involved. In some species (e.g. the equine and canine), sires breed relatively few females and, owing to the innate variation present between ejaculates and males, they yield fertility results of less analytical reliability than livestock species in which sires breed with large numbers of females. In the equine, as stallions might breed with the same females over several oestrus cycles per season, the resulting cumulative fertility outcome ought to be properly expressed (as per oestrus or mating/AI, or per season) for reliable comparisons. The bottom line is that the larger the number of females mated or inseminated with a certain male, the higher the stringency of the fertility registry. Controlled mating or AI is used in livestock (bovines, ovines, porcines) where the ejaculate of a sire is used on a large number of females via AI and the number of bred females is high, both allowing an adequate register of male fertility via a large number of opportunities of conception. In dairy cattle, there is a generalized use of the average rates of non-return to oestrus (non-return rates, NRR) when 28, 56, 60–90 or 124 days have elapsed since the first AI. The NRR is a composite parameter, resulting from conception and gestation variables, two essential events that show the effectiveness of AI. These data must be corrected for conditioning factors using appropriate statistical methods (mixed model, repeated measures); these modify the initial means by the effects of the herd (number of animals, location of farm), the number of AIs (1st or 2nd), female characteristics (age, parity, milking stage, etc), year, season, inseminator, day of the week, etc., to avoid drawing false conclusions. In addition, sire fertility varies over time (Hallap et al., 2004, 2006a), and this must be considered too. Boar studs can be subjected to a similar approach, while introducing new parameters such as prolificacy (number of piglets born) and even viability (numbers of piglets born alive). Estimations of in vitro fertility depend on the type of test used to study a heterogeneous suspension of pluri-compartmental, terminally differentiated cells: the spermatozoa. Current assessment of ejaculated spermatozoa focuses almost solely on the number of spermatozoa (per unit of volume, i.e. concentration or as total per ejaculate) and their motility (sometimes including their kinematic patterns, if a CASA instrument is used), although other aspects, such as pH and ejaculate volume are also included. Few studies are published on the relation between neat semen and fertility because after collection (when it is at 35°C), semen is always extended and then either chilled or frozen (from room temperature at +20°C, or fridge temperature at +5°C, or anything in between), and used thereafter for AI; these processes introduce other variables into the assessment of semen (its extension, dilution of the SP, additive effects, cooling, time, etc) – considerations that will be discussed later. The same considerations apply to sperm numbers, which implies that each sperm AI dose contains a specific number of spermatozoa. However, as handling (including cryopreservation and other manipulations) causes cell death, shortens lifespan and modifies the fertilizing capacity of various proportions of spermatozoa, sperm numbers in an AI dose are often excessive so that breeders are safeguarded in providing fertility to their customers. Only when viable sperm numbers are decreased to possible ‘threshold numbers’ do we see a proven relation between sperm numbers and fertility (Tardif et al., 1999; Christensen et al., 2011). Subjectively assessed sperm motility has been statistically related to fertility even for post-thawed semen in bulls (Rodriguez-Martinez, 2003) and in pigs (Cremades et al., 2005). Studies in other species have yielded erratic results, with large variation between laboratories, owing to operator bias and differences in numbers of breedings/female numbers used to determine fertility (Rodriguez-Martinez, 2006). Kinematic analyses using CASA instruments have shown variable correlations between particular motility patterns, such as linearity, and fertility in the field (Bailey et al., 1994; Holt et al., 1997; Zhang et al., 1998; Hirai et al., 2001; Januskauskas et al., 2001, 2003). Further, combining motility patterns with other parameters of sperm function in AI dairy sires has allowed a reasonable estimation of fertility (Januskauskas et al., 2001), as exemplified in Fig. 19.1. Major constraints for conventional CASA instrumentation relate to the variability between users (Ehlers et al., 2011), as well as to the low sperm numbers analysed per sample; when these factors are combined with the still high cost of the instrumentation, the wider use of CASA instruments is jeopardized (Feitsma et al., 2011). There is now alternative instrumentation available (Qualisperm™, Biophos, Switzerland, Plate 62) that is based on a different principle from that used in CASA, i.e. the classical digitalization of centroid movement over time. This novel technology is based on correlation analysis of single particles (spermatozoa) in confocal volume elements. Individual spermatozoa are projected on to a pixel grid of a CMOS (complementary metal-oxide semiconductor) camera, and the algorithm analyses the number of fluctuations using a correlation function instead of trajectories (as in the CASA system). The new system benefits from a high throughput (usually four fields per minute) and analyses >2000 spermatozoa/sample; it has been thoroughly tested on several species (Tejerina et al., 2008, 2009; Johannisson et al., 2009). Most often, the proportion of morphologically normal spermatozoa in the ejaculate of a bull is related to its fertility post AI (Phillips et al., 2004; Al-Makhzoomi et al., 2008), by reflecting, together with sperm numbers and their motility, the degree of normality of spermatogenesis and sperm maturation within a cohort of sires. Continuous selection of breeding sires, and of dairy bulls in particular, for sperm quality (including morphology) has substantially decreased the incidence of sperm abnormalities in bulls of similar age within the space of 30 years (see Rodriguez-Martinez and Barth, 2007). Morphological abnormalities are always present in any ejaculate, although various forms of abnormality differ in their impact on fertility. Some are specific defects that hamper fertilization, while others, such as the pyriform or pear-shaped sperm head deviation, impair proper embryo development (Rodriguez-Martinez and Barth, 2007), thus calling for frequent (intervals of 2 months) detailed assessments of sperm morphology in AI stud sires using wet or stained smears (Plate 63a,b). The reliability of such analyses requires large numbers of spermatozoa to be assessed per sample, i.e. 200 per wet-smear and 500 for stained sperm heads; the latter allows for determinations of defects that have clear relationships with fertility due to their uncompensable nature, such as the pyriform sperm head shape (as an expression of a defective chromatin condensation during spermiogenesis) (Al-Makhzoomi et al., 2008). Software for the ASMA system has been developed since the 1980s, and has now reached an acceptable reliability for the analyses of sperm head dimensions, though other types of sperm abnormalities cannot yet be determined (Auger, 2010), and nor are there clear relationships of sperm head morphometry with fertility (Saravia et al., 2007b; Gravance et al., 2009). At specialized laboratories, the evaluation of semen in vitro goes beyond routine andrological screening. First of all, these laboratories attempt to disclose biomarkers of sperm function (e.g. intactness and reactivity of membranes, organelles, chromatin, etc.) that have proven relevant for fertilization (Silva and Gadella, 2006). Thereafter, they explore, via in vitro testing, how relevant the interactions are that occur between the spermatozoa and the female genital tract, and with the oocyte vestments; they also examine the process of fertilization itself, including the early development of the embryo. Finally, they seek to determine how the different outcomes relate to fertility (Rodriguez-Martinez, 2007b). A summary of current exploration methods for these sperm attributes, and of the various functional tests available, is provided in Table 19.1. Intactness, stability and reactivity of the plasma membrane to stimuli from surrounding fluids and cells are prerequisites for the viability and fertilizing capacity of the spermatozoon. The simplest method used nowadays to study the integrity of the plasmalemma, besides microscopy using wet smears or the use of the membraneimpermeable dye eosin (such as the eosin–nigrosin test), is to expose spermatozoa to a hypo-osmotic saline solution (HOS-test or HOS-t). Cells with an intact, functional membrane change in volume by allowing water to enter the cell. Results from both the HOS-t and its subsequent development into the ‘cell volumetric electronic cell sizing method’ (Neild et al., 2000; Petrunkina et al., 2001) have provided significant correlations with fertility. Table 19.1. Summary of sperm attributes to be evaluated, current and possible future methods for assessment, and their individual or combined relations to fertillty The most advantageous use of fluorophores is when they are combined. For instance, subtle changes in the plasma membrane such as modifications in permeability can be disclosed by combining several fluorescent probes, such as SNARF-1, YO-PRO-1 and ethidium homodimer, the so-called triple stain (Peña et al., 2005a, 2007), as exemplified in Plate 65. Viable, membrane-stable spermatozoa are able to pump out small probes such as YO-PRO-1, which always enters living cells. Sub-viable cells, i.e. those with incipient membrane destabilization, become permeable and unable to pump out YO-PRO-1, which then stains the DNA bright green and can easily identify the sub-viable cells before they show other signs of degeneration (Peña, 2007). Membrane permeabilization is followed by modifications of the position of phospholipids such as phosphatidylserine (PS), which can be mapped by the fluorophore combination of Annexin-V/PI (Januskauskas et al., 2003; Peña et al. 2003, 2005a, 2007; Saravia et al., 2007a). Earlier changes in the membrane, related to the destabilization of the lipid layer, can also be followed using a triple combination of fluorophores: Merocyanine-540 (Mero-540), YO-PRO-1 and Hoechst 33342. Plate 66a–b shows typical readings obtained using two different probe combination for the estimation of sperm asymmetry. This approach has proven effective in disclosing defective spermatozoa in several species, and gives good correlations with fertility (Hossain et al., 2011a). Acrosome intactness, a prerequisite for fertilization, can be readily examined in vitro using phase contrast microscopy (Rodriguez-Martinez et al., 1998), or it can be examined by fluorophore-linked lectins using multiparametric analysis (Nagy et al., 2003, 2004). Spermatozoa can be stained with different dyes, i.e. SYBR-14/PI to identify membrane integrity, with PE-PNA(phycoerythrin conjugated peanut agglutinin) for acrosome integrity (see Plate 67), and Mitotracker Deep Red for mitochondrial intactness (Nagy et al., 2003, 2004). The intactness of the approximately 100 mitochondria of sperm is indispensable for cell life and function, from motility to capacitation. Despite this, correlations between mitochondrial status and fertility have been variable, mostly owing to the changes in mitochondria functionality over time, as monitored when using the mitochondrial stain JC-1 (5,50,6,60-tetrachloro-1,10,3,30-tetraethylbenzimidazolyl-carbocyanine iodide), which can discriminate between spermatozoa with poorly and highly functional mitochondria (Martinez-Pastor et al., 2004; Hallap et al., 2005b; Peña et al., 2009), as exemplified in Plate 68a,b. Besides energy, sperm mitochondria produce by-products of the metabolism of oxygen, including superoxide. This superoxide converts into the damaging hydrogen peroxide, a reactive oxygen species (ROS), which is mostly, but not completely converted to oxygen and water by the enzymes catalase or superoxide dismutase (also known as antioxidants or scavengers). A certain level of ROS is essential for sperm function, including fertilizing capacity, but only when it is kept at optimal levels by the antioxidant capacity of SP (Awda et al., 2009; Mancini et al., 2009; Am-in et al., 2011). When excessive numbers of leucocytes are present in the ejaculate or the semen is subjected to oxidative stress (as during cooling in the absence of SP or other natural scavengers), increased ROS generation, either extrinsic (leucocytes) or intrinsic (sperm neck cytoplasm in immature or morphologically abnormal mitochondria), act to deteriorate sperm motility (Guthrie et al., 2008), sperm membranes – through lipid peroxidation (LPO), and DNA integrity – via breakage and cross linking of the chromatin (Aitken and West, 1990; Koppers et al., 2008). ROS levels are very variable, making their proper determination difficult, though this is possible using the probe hydroethidine. Plate 69 shows such an FC mapping of sperm ROS obtained by loading the sperm with hydroethidine and Hoechst 33258. A useful alternative, which measures oxidative stress and ROS effects indirectly, is to determine the levels of LPO in the membrane lipid bilayer by using the 5-iodoacetamidofluorescein probe family (BODIPY-C11®) (Aitken et al., 2007; Guthrie and Welch, 2007; Ortega Ferrusola et al., 2009a). A representative scanning in stallion spermatozoa is shown in Plate 70a-d. Mammalian spermatozoa have tightly compacted eukaryotic DNA, established by transformations during spermiogenesis in which the sperm chromatin replaces histones, first by transient proteins and then by protamines (Oliva and Castillo, 2011). The DNA strands are highly condensed by these protamines and form the basic packaging unit of sperm chromatin: the toroid. Toroids are even further compacted by intramolecular and intermolecular disulfide cross links between protamine cysteine residues (Schulte et al., 2010). There are differences in compaction between species; those with less compacted chromatin (equines or canines, for instance) are theoretically more prone to DNA damage. Protamine deficiency in loosely packed chromatin can be monitored by the fluorochrome chromocanmycin-A3 (CMA3) (Tavalaee et al., 2010). Sperm chromatin can show different abnormalities related to compaction: from damage to the actual DNA physical integrity – as single- or double-stranded DNA strand breaks, nuclear protein defects interfering with histone or protamine conversion and DNA compaction – to chromatin structural abnormalities such as defective tertiary chromatin configuration. The latter scenario implies that defects will occur in the decondensation of the nucleus, thus impairing fertilization, whereas the other defects can jeopardize embryonic development as the oocyte (albeit being able to repair a limited amount of sperm DNA damage) would not be able to correct such damage (Johnson et al., 2011). Sperm DNA disorders also include mutations, epigenetic modifications, base oxidation and DNA fragmentation (which is also related to sperm handling). In accord with knowledge of its increasing relevance, the evaluation of DNA integrity has increased over recent years (Barratt et al., 2010). DNA fragmentation, by being considerably present in the sperm of sub-fertile males, is considered to be the most frequent cause of paternal DNA anomalies transmitted to progeny. Damaged sperm DNA may be incorporated into the genome of the embryo, and participate in or lead to errors in DNA replication, transcription or translation during embryo development, ultimately contributing to diseases in future generations (Katari et al., 2009). Moreover, DNA damage may remain in the germ line for generations, a matter of concern related to the increasing use of ICSI (which, today, is even used in animals) (Aitken et al., 2009). Sperm DNA fragmentation can be studied by many techniques, including staining with the DNA fluorophore PI, which, in species where DNA compaction is not high, can present two types of staining, i.e. dimmer and brighter (Muratori et al., 2008). The former relates to low sperm quality, while the latter is present among spermatozoa with good motility and morphology, without necessarily affecting their capacity to fertilize. This might not represent a problem, provided that the amount of damage is not high, because a fully mature oocyte has the capability to repair some degree of DNA damage, for instance single-stranded DNA breaks. Several methods are used to determine DNA damage: (i) the single-cell gel electrophoresis assay (COMET); (ii) the terminal deoxynucleotidyl transferase-mediated fluoresceindUTP nick-end labelling (TUNEL) assay; (iii) the acridine orange test (AOT); (iv) the tritium-labelled 3H-actinomycin D (3H-AMD) incorporation assay; (v) the in situ nick translation (ISTN) assay; (vi) the DNA breakage detection-fluorescence in situ hybridisations (DBD-FISH) assay; (vii) the Sperm Chromatin Dispersion test (SCD Halo); or (viii) the evaluation of the degree of induced denaturation of the DNA (the so-called ‘sperm chromatin structure assay’, SCSA) (Fraser, 2004; Evenson and Wixon 2006; Tamburrino et al., 2012). Examples of spermatozoa examined with some of these techniques are illustrated in Plate 71a–d. Most of the above methods can use fluorescence microscopy, but SCSA and TUNEL are usually explored via FC. Although SCSA has been extensively used, the outcome has provided conflicting relationships with fertility (Rodriguez-Martinez and Barth, 2007; Christensen et al., 2011). The conflict has arisen from a single study relating the percentage of spermatozoa with denatured DNA (through an index of DNA fragmentation, DFI), to high, moderate or very low fertility values in humans, when DFI thresholds of ~0–15%, 16–29% and >30%, respectively, were recorded as in direct relation to the pregnancy outcome using ART (Evenson and Jost, 2000). Such relationships have been questioned (Collins et al., 2008). A threshold for sub-fertility in bulls and boars has also been calculated (as percentage DFI), albeit using semen from young, unproven bulls with low fertility (40–60% of 90 day NRRs) and boar semen without any fertility recordings (Rybar et al., 2004). These calculated threshold values (20% for bulls and 18% for boars) were much lower than those obtained with human semen. The lack of reliable fertility data when calculating these limits also leaves these values highly questionable, especially in the light of other, wellcontrolled studies of AI-sires with proven and varied fertility, in which neither proven stud bulls nor stud boars in AI-breeding programmes reached these high values. For instance, sperm DFI values (even as a percentage of cells with high COMP at values (i.e. cells outside the main population with a high proportion of single-stranded DNA over single + double-stranded DNA) in breeding sires are low (<5%) (Boe-Hansen et al., 2005; De Ambrogi et al., 2006; Christensen et al., 2011), even in frozen–thawed semen, where the processing ought to facilitate DNA damage (Januskauskas et al., 2001, 2003; MartinezPastor et al., 2004; Hallap et al., 2005a; Hernández et al., 2006; Chistensen et al., 2011). Conversely, equine sires unselected for sperm quality had higher DFIs and these lacked a clear relationship with equine fertility (Morrell et al., 2008). SCSA does not specifically identify the amount of DNA damage, unlike TUNEL, but rather its susceptibility to harsh treatment. The TUNEL method is more cumbersome though, and this has led to modifications of the method. For example, a TUNEL/PI procedure is now available that combines the accuracy of TUNEL and the differentiation of two sperm populations depending on PI intensity, of which one is probably participating in fertilization as the damage has no relationship with either sperm motility or morphology (Muratori et al., 2008). Alternatively, the use of dithiothreitol (DTT) to decondense sperm nuclei and inclusion of a stain for dead cells provides a higher accessibility to the TdT (terminal deoxynucleotidyl transferase) enzyme of TUNEL, in combination with the detection of DNA fragmentation in live spermatozoa (Mitchell et al., 2011). Taking all the above considerations into account, TUNEL appears to be a more sensitive method to predict infertility than SCSA, as determined in a recent meta-analysis (Zini et al., 2008). The structural integrity attributes of the sperm that have already been described are a prerequisite for sperm function, provided it is proven that they work in a concerted manner. For instance, it is logical to consider that the determination of membrane integrity in ejaculated spermatozoa is linked with sperm motility, as well as with the capacity of viable spermatozoa to undergo sperm capacitation and change their activated motility to a hyperactivated pattern, as occurs in vivo (Rodriguez-Martinez, 2007a). Sperm capacitation is, moreover, a stepwise process that includes the removal of epididymal and SP-adsorbed proteins, loss of membrane cholesterol, an increase in membrane fluidity due to lipid modifications, an influx of Ca2+ to the sperm perinuclear and neck regions and flagellum, the generation of controlled amounts of ROS, as well as the phosphorylation of protein residues (Gadella and van Gestel, 2004; Harrison and Gadella, 2005; O’Flaherty et al., 2006; Tulsiani et al., 2007; Fabrega et al., 2011). These are steps that can be measured in vitro and, ultimately, associated with the fertility of the males. The earliest stages of sperm capacitation, those related to bicarbonate and Ca2+ triggered phospholipid scrambling, can be mapped using Merocyanine-540 or Annexin-V, as these measure general or specific destabilization of the plasmalemma and both are related to the fertility of the semen assayed, either fresh (Harrison, 1997) or frozen–thawed (Peña et al., 2004, 2007; Januskauskas et al., 2005; Hallap et al., 2006b), when a bicarbonate challenge has been used (Bergqvist et al., 2006; Saravia et al., 2007a). Calcium influx elicits tyrosine phosphorylation, and apparently initiates sperm capacitation and hyperactivation (Suarez, 2008a,b). Therefore, mapping of low intracellular Ca2+ levels in spermatozoa should be a good parameter for discriminating between semen samples (or even individual boars) (Hossain et al., 2011b; Waberski et al., 2011). Ca2+ influx and displacement within the spermatozoon have been followed throughout capacitation using Indo-1 acetoxymethylester or Fluo-loaded spermatozoa (Hossain et al., 2011a,b). Most changes in relation to capacitation are registered during the later stages of this process, and can be indirectly visualized by the incubation of viable spermatozoa with the fluorescent antibiotic chlortetracycline (CTC), which monitors the displacement of Ca2+ in the sperm head, in preparation for the occurrence of the acrosome reaction (AR) (Rodriguez-Martinez, 2007a). In AI bulls with known fertility, the proportion of noncapacitated spermatozoa (CTC stable, unreacted) in AI semen samples was significantly related to fertility (Thundathil et al., 1999; Gil et al., 2000). The capacitation of spermatozoa, following Ca2+ and bicarbonate signalling, activates adenylate cyclase and the resulting cAMP in turn activates intracellular protein kinases that enrich the equatorial subsegment in tyrosine-phosphorylated proteins (Piehler et al., 2006; Jones et al., 2008). There is now an anti-phosphotyrosine antibody, and FC can estimate the amount of global protein residue phosphorylation in the sperm plasma membrane, thereby providing evidence of the real-time proportion of spermatozoa that might have engaged in the process of capacitation (usually 5–10% of all cells at a given time) (Sidhu et al., 2004). When the AR was induced in vitro by exposure to homologous zona pellucidae (ZPs), or to specific proteins isolated from the ZP (Rodriguez-Martinez, 2006), it was clear that not all spermatozoa show the AR immediately upon challenge with ZP proteins, indicating that they do not respond at one time to the stimulus, as seen for capacitation (see above). Timed retrieval of spermatozoa from pig oviducts (Tienthai et al., 2004) in relation to spontaneous ovulation indicated that most spermatozoa remain uncapacitated before ovulation, perhaps as a result of their sequential exposure to the bicarbonate-rich upper oviductal fluid (Rodriguez-Martinez, 2007a). Overall, the data suggest that slow responders to species-specific effectors of capacitation or the AR would probably be the ones potentially fertilizing in vivo, confirming the findings of an existing relation between the percentages of non-capacitated spermatozoa (stable; see above) and fertility. Easier in vitro AR-inducing procedures have been attempted, including exposure to progesterone (Aitken, 2006), selected glycosaminoglycans (GAGs), or the divalent cation ionophore A23187, which bypasses the physiological receptor activation/signal transduction yet promotes a massive Ca2+ influx similar to that recorded during capacitation and sperm–ZP binding (see Rodriguez-Martinez, 2006). In either case, rates of AR-responsiveness were significantly and positively related to fertility (as 56 day NRR post AI in cattle, or as farrowing rates in pigs). This is illustrated in Fig. 19.2 for frozen–thawed spermatozoa from individual AI dairy bull sires (diamonds) with various AI fertility in the field (Januskauskas et al., 2000a,b). Spermatozoa from human males, boars and bulls evidently contain the hyaluronan (HA) receptor CD44 in their plasma membranes (Huszar et al., 2003; Tienthai et al., 2003; Bergqvist et al., 2006) and should thus bind to solid state HA depots (PICSI Sperm Selection device, USA) (Huszar et al., 2007). The HA binding seems to trap only mature spermatozoa that are able to react with the HA and exhibit some degree of hyperactivated-like motility pattern. This technique was primarily designed to select the best spermatozoa for ICSI in humans, but was later used for stallion spermatozoa (Colleoni et al., 2011). The spermatozoa assessed were not only mature, but even had intact chromatin (Razavi et al., 2009; Yagci et al., 2010) – although they were not selected for normal morphology (Petersen et al., 2010). However, the number of spermatozoa that have been explored with this method is small, and relationships between sample test results and fertility are yet to be established. An alternative to solid-state HA depots is HA-containing media, in which the results of sperm selection are quite similar, but where the fluid allows for higher sperm numbers (Parmegiani et al., 2010). Spermatozoa have also been explored for their ability to bind to the epithelium of the oviduct in vitro, an ability present in vivo, when potentially fertile spermatozoa reside in the oviductal sperm reservoir (Rodriguez-Martinez et al., 2005). The binding of spermatozoa to homologous oviductal epithelial cells in vitro has been found to prolong their life (Lefebvre and Suarez, 1996), thus indicating that sperm co-culture with oviductal explants might mimic the capacity of a semen sample to colonize the tubal reservoir and would, therefore, indicate its potential fertilizing capacity, because uncapacitated spermatozoa are preferentially bound (Fazeli et al., 1999). It should be noted here that the outcomes of such tests only provide reliable correlations when high-quality sperm samples are tested (De Pauw et al., 2002), and have shown only marginal relationships with fertility (Waberski et al., 2005, 2006). The effective binding of the spermatozoon to the ZP is a critical step in the process of fertilization. The binding is species specific, only elicited by capacitated spermatozoa and precedes the AR. As ZP binding can easily be performed in vitro, several sperm–ZP binding tests have been designed since the 1980s, either using whole ZPs (oocytes), or hemi-ZPs (cleaved oocytes) (Rodriguez-Martinez, 2006). Even though the findings from ZP-binding tests have yielded significant correlations with AI fertility in pigs (Lynham and Harrison, 1998; Ardon et al., 2005) and bulls (Zhang et al., 1998), the biological significance of this assay is questioned, mainly because physiological sperm capacitation, and hence the AR, do not involve all spermatozoa at a given time. A representation of the relationship of a ZP binding (in whole oocytes) to fertility, depicting the presence of false positive and false negative cases, is shown below (Fig. 19.3). An alternative method tested in many laboratories is the ability of presumably capacitated spermatozoa to penetrate into homologous oocytes in vitro, the so-called oocyte penetration test, under conditions of in vitro oocyte maturation (IVM) (Martínez et al., 1993; Henault and Killian, 1995; Brahmkshtri et al., 1999; Oh et al., 2010). This test allows for screening of degrees of polyspermia, numbers of accessory spermatozoa in the ZP, decondensation ability (male pronucleus formation) and the testing of several males simultaneously (provided that each semen sample is loaded with a different fluorophore), and it also relates to fertility (Henault and Killian, 1995; Gadea, 2005). The method is simple and requires little equipment, though (i) the oocytes maturity level rules, and (ii) not all spermatozoa at a given time are capacitated and prompted to engage in penetration of the ZP. These basic timing hurdles might explain some of the variation in penetration rates found, rather than mirroring possible fertility differences among sires. Different end points in fertilization and subsequent early embryo development can be determined using IVF, so spermatozoa of various species have been investigated for relationships between in vitro outcome/s and field fertility when the same semen (or males) was used for AI. In most cases, the approaches were retrospective, i.e. the fertility levels of the semen or males used were already known; only a few tests were really done prospectively, i.e. the semen was coded, used in vitro and the outcomes used to calculate an ‘in vitro fertility’ that was subsequently compared with the ‘real fertility’ in the field. It soon became apparent that significant relations appeared even when the semen used had wide variations in fertility, and results could be accepted as reliable when the conditions for IVF were of a certain stringency and stability, i.e. low sperm numbers used, there were the same levels of success in a control line over time, there were no major differences between cleavage and morula/blastocyst yields, etc. Unfortunately, in most studies (see Rodriguez-Martinez, 2007b), there were only low-to-medium relations with fertility (Table 19.1), and these were lowest for morula/blastocyst rates. As it can be seen in Table 19.1, the outcomes of most in vitro analyses of individual sperm attributes and/or functional assays (i.e. from motility to embryo development assays) show variable relationships with fertility, and these are not always statistically significant. This should not come as a surprise, because the ejaculate is not a suspension of a homogenous population of spermatozoa. Spermatozoa within an ejaculate differ morphologically, they move differently and their genome and transcriptome differ between cells. All cells, even those that were ‘siblings’ during spermatogenesis, are in principle different. For that reason, they neither display the same ability to interact with other cells nor have the same fertilizing capacity. The ejaculate is, therefore, considered to be a heterogeneous accumulation of various sperm cohorts – products of different spermatogenic waves that had matured along the ducti epididymides and were stored in their caudae as different cell sub-populations until mixed in the ejaculate. Moreover, the use of functional in vitro methods (ZP binding, IVF, etc.) bypasses some of the functional deficits of spermatozoa and aggravates the degree of uncertainty for relations with fertility in vivo (Barratt et al., 2011). Differences are apparent between the assays and/or attributes tested. For instance, membrane integrity evaluated via FC or fluorometry appeared to be more closely related to semen fertility than was sperm motility. This does not imply that some of the testing can be eliminated. For example, sperm motility is particularly difficult to eliminate as it embraces a number of attributes, including intactness of the plasmalemmae; it is also a simple and inexpensive procedure. Furthermore, it is important to remember that the significance of any differences encountered is a direct function of the number of spermatozoa examined (sample power), such as might be exemplified by the difference between assessing 100 spermatozoa or thousands per sample. Prognostic strength can be also gained by carrying out different assays, even when this implies that some attributes are measured more than once. There is little inherent risk in such an approach, as the spermatozoa tested with one assay are different from all others; thus, a battery of tests is always advantageous (Rodriguez-Martinez, 2003). In line with this approach, several groups have combined the results of in vitro tests of the same semen samples in multiple regression analyses (see Rodriguez-Martinez and Barth, 2007), which have yielded higher correlations with fertility, even when they are retrospective (see Table 19.1). Calculation of ‘predicted’ fertility, in which the outcomes of various methods of semen evaluation in vitro were combined in multivariate analysis before the fertility of the ‘donor’ males was tested in the laboratory or the field, has proven valuable (Zhang et al., 1999; Gil et al., 2005; Ruiz-Sanchez et al., 2006). Figure 19.4 depicts how in vitro data could be predictive of in vivo fertility when the results of various in vitro methods of evaluation of frozen–thawed semen from unproven young dairy AI bull sires (11–13 months of age) were combined to calculate their expected (potential) field fertility. A strong relationship (r = 0.90) was found between predicted and real fertility. This approach enabled identification of sub-fertile bulls, whose expected and real fertility was below the limit considered for sub-fertility (a 62% non-return rate); in contrast, other young bulls that had been predicted to have satisfactory fertility had non-return rates of ≥65%. Identification of sub-fertile sires have been obtained with other bull(Januskauskas et al., 2000b; Hallap et al., 2004) and boarstud populations (Ruiz-Sanchez et al., 2006). Interestingly, most sperm parameters (and to some extent even fertility) appeared to be maintained over the functional lives of the sires, provided no pathologies are acquired between measurements (Zhang et al., 1997, 1998; Hallap et al., 2005a, 2006a). However, intrinsic variation between ejaculates within sire was always present, which requires analyses of many ejaculates. The spermatozoa we examine are not only different from each other, they are differently processed. They are either collected in different parts of the ejaculate (from those species with fractionated ejaculate) or they are extended, chilled, frozen, or even subjected to sperm sorting, or further prepared for IVF. All of these different scenarios result in spermatozoa with added differences in the intactness of the attributes that they need to interact with the environment (the female), including fertilization. This superimposed variation confounds further our capacity to discern the intrinsic value of in vitro outcomes for the estimation of potential fertility from iatrogenic implications. In other words, we need to apply a holistic view when examining a subsample of semen. Can we mimic sperm selection in the oviduct? Probably so, but only to some extent, because we still do not know if or how the female modulates all of the steps involved behind the restrictions in vivo. We do know, however, that ejaculated spermatozoa can be separated in vitro into sub-populations by differences in motility kinetics (Abaigar et al., 1999; Cremades et al., 2005; Peña et al., 2005b, 2006; Holt et al., 2007; Martinez-Pastor et al., 2011), membrane integrity (Perez-Llano et al., 2003), head morphometry (Núñez-Martinez et al., 2005; Peña et al. 2005b, 2006), sustainment of handling and cryopreservation (Gil et al., 2005; Peña et al., 2006; Roca et al., 2006), and the ability to respond to specific stimuli such as bicarbonate exposure (Holt and Harrison, 2002; Gadella and van Gestel, 2004; Holt and Van Look, 2004; Satake et al., 2006), or to specific proteins isolated from the oviduct (Coy et al., 2009). Several of these studies were based on individual sperm attributes, and some considered the fluids surrounding the spermatozoa, their composition and even their rheological features, with viscosity being among these. The ultimate goal would be to find a method that, by mimicking what occurs within the female genital tract, could preselect spermatozoa with primary competence for fertilization (Rodriguez-Martinez, 2006). Methods for the in vitro
Linköping University, Linköping, Sweden
Introduction
Clinical Andrology and Diagnostic Seminology: Can they Prognose Fertility – or Just the Risk Level of Never Reaching Fertile Status?
Does the Exploration of Other Sperm Attributes, including Testing Sperm Function In Vitro, Prognose Fertility?
Functional Assays, Higher Discriminatory Value?
Have We Found Biological Relationships with Fertility that Have Diagnostic and Prognostic Value?
Sperm Selection: The Panacea?
Stay updated, free articles. Join our Telegram channel

Full access? Get Clinical Tree
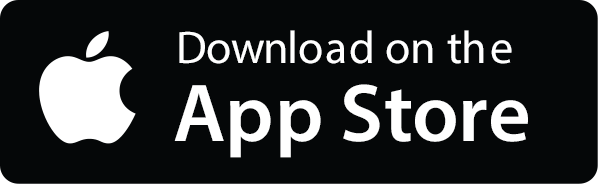
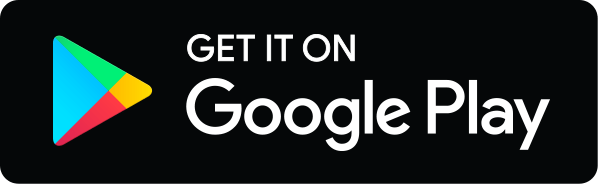