Julie A. Smith1 and Carrie Davis2 1 MedVet Vancouver Emergency Veterinary and Specialty Care, Vancouver, Washington, USA 2 MedVet Dallas Emergency Veterinary and Specialty Care, Dallas, Texas, USA Anesthesia is often required for patients undergoing procedures that incorporate the use of devices that could present a danger to the patient or the anesthetist. This chapter will focus on how to ensure a safe environment for both the anesthetist and the patient during the use of lasers and for diagnostic imaging procedures using radiation or high‐strength magnetic fields. The word “laser” is an acronym for Light Amplification by Stimulated Emission of Radiation. Surgical lasers produce an intense beam of pulsed or continuous invisible infrared light (radiation) that can be hazardous [1–3]. Laser devices are classified and labeled by the manufacturer according to their potential to cause biological damage. The classifications range from Class 1, the safest, to Class 4, the most hazardous. Wavelength, output power, and tissue exposure time are parameters used to categorize lasers and determine which precautions are necessary [4]. The most common lasers utilized in veterinary procedures have high emission levels and are listed in Class 3b or Class 4. The American National Standards Institute’s Z136.3 document provides guidance for the safe operation of lasers in healthcare facilities, including veterinary hospitals [5–6]. The primary hazard associated with Class 3b and Class 4 lasers is related to accidental exposure to laser emissions. Exposure may occur directly from the laser beam, or when the beam is reflected from a polished surface, metal instruments, or other objects. Reflected laser beams are unaltered and contain the same energy as the direct beam. Backscattering can also occur as the energy partially reflects on impact with tissue. The energy reflected in backscatter is less than the direct or reflected beam but may still have a damaging effect on staff and equipment. The Nd:YAG and the Argon laser are known to produce significant backscatter. The parts of the body at greatest risk are the eyes and skin. The eye is extremely sensitive to laser radiation and can be permanently damaged from direct, diffuse, or reflected beams. Even brief or partial exposures can instantaneously damage the cornea, lens, or retina [1–3]. The extent of ocular damage is determined by the laser irradiance, exposure duration, and beam size. Infrared radiation is invisible; therefore, it is possible for eye damage to occur without awareness that an exposure has occurred. Since the eye cannot detect the invisible beam, it does not respond with a blink reflex or by averting the eye from the beam. Laser‐induced eye injury can occur in one or both eyes and may be temporary or permanent [3]. Table 4.1 Most common surgical lasers used in veterinary medicine. Common types of lasers used in veterinary procedures are listed along with their wavelengths and optical density number necessary for proper eye protection. Source: Adapted from OSHA [1]. All persons within the operating area are at risk of eye injury, so it is critical for the anesthesia provider to take precautions and to use protective eyewear (Box 4.1). The United States Occupational Safety and Health Administration (OSHA) requires the use of eye protection for personnel in the presence of lasers that may result in eye injury [1]. Protective eyewear styles look similar but are not all the same. Options are based on the type of laser in use and rated for the specified wavelength range. Eyewear is also rated for its optical density, which is its ability to reduce the beam power. The optical density protection level is unique to each laser (Table 4.1) and will be recommended by the manufacturer in the laser operation manual. The wavelength and optical density for which the protective eyewear is rated can be found imprinted directly on the glasses (Fig. 4.1). It is important to observe warning signs in areas where lasers are in use and not enter without eye protection to prevent inadvertent exposure. Eye protection is also important for the patient. The patient’s eyes should be protected from direct or scattered laser beam by making sure that the eyelids are closed or protected by drapes. Pet eye protection goggles are available in a variety of sizes that are suitable for use with lasers classified as OD 6+ with wavelengths between 800 and 1100 nm. Skin damage from exposure to the direct laser beam is possible for personnel who are very close to the procedure site. The severity of skin damage depends on the total energy deposited and penetration depth of the laser beam. Injuries range from a mild erythematous reaction to a severe burn. Drapes are effective protection for patients, and skin protection is usually not necessary for personnel in the laser use area because the energy density decreases rapidly beyond the focal point [2,3]. Figure 4.1 Protective eyewear performance characteristics are recommended by the manufacturer in the laser operation manual. The optical density (OD) and wavelength (nm) for which the protective eyewear is rated is imprinted directly on the glasses. Source: Dr. Kendall Taney, DAVDC, Center for Veterinary Dentistry and Oral Surgery, Gaithersburg, MD, with permission. The direct or reflected beam of a high‐emission Class 3b or Class 4 laser can ignite combustible materials and is a fire risk. Devastating operating room fires and combustion of the endotracheal tube (ETT) leading to fire in the trachea and lower airways are described in human medicine [7,8]. There is a single report documenting fire occurring during laser surgery in veterinary patients. It describes the ignition of the area around the face mask during inhalation anesthesia in two pet rodents, a mouse and a hamster, during cutaneous mass removal with a diode laser. The fire resulted in life‐threatening burns on the face, head, and upper body of both rodents. Only one animal survived [9]. General anesthesia with inhalant anesthetics and high concentrations of oxygen are commonly used for immobilization during laser procedures. Anesthesia may be delivered through an ETT or by face mask depending on the species and situation. Lasers are frequently utilized for procedures in the oral cavity and close to the airway in veterinary patients where intubation is necessary to ensure proper ventilatory support [10,11]. Commonly used ETTs made of polyvinyl chloride (PVC), red rubber, and silicone are combustible [7,12]. The intense heat of the laser can burn through the ETT in seconds and cause a fire when the beam encounters the oxygen‐enriched gas inside the tube [13]. Metal, copper‐shielded, and insulated ETTs designed for use with laser procedures are commercially available and are the standard of care in human anesthesia care. However, these tubes are expensive, bulky, and not universally safe for all types of lasers, nor are they available in sizes suitable for horses or very small patients [7,14]. A latex red rubber ETT, tightly wrapped with spirally overlapping loops of self‐adhesive non‐reflective aluminum or copper tape, was reported as safe or safer than commercially available stainless steel or insulated ETTs for use with CO2 and Nd‐YAG lasers [7,14–18]. Wrapping the ETT increases the external diameter and makes the surface potentially more irritating to delicate tracheal tissue. Tape can come loose from the wrapped ETT, and it is necessary to carefully examine the tube following extubation to ensure that none of the tape came off in the trachea [2,7,11,14,16]. A simple and effective method for protecting the ETT from combustion is to cover it with a thick layer of saline‐soaked sponges [7,19]. Keep the sponges moist throughout the procedure to dissipate heat from any inadvertent exposure from the laser beam. Count sponges before and after placement to ensure that all sponges have been removed from the oropharynx prior to recovery. Figure 4.2 Crisis checklist in the event of an airway or operating room fire during laser surgery. The ETT cuff and pilot tubing are also susceptible to damage during laser surgery [2,7]. Inadvertent deflation of the cuff will allow leakage of high oxygen concentration and anesthetic gases into the oral cavity and around the head of the patient. Fire may result if the enriched oxygen gas contacts the laser beam. Protecting the pilot tube with saline‐soaked gauze sponges and using saline instead of air to fill the cuff will dissipate heat and maintain patency of the cuff. Adding a dye, such as new methylene blue, to the saline used to inflate the cuff may indicate if the pilot tube is compromised [2,7,19]. When the use of a face mask to deliver inhalant anesthesia in high concentrations of oxygen cannot be avoided, efforts should be made to fit the mask tightly around the face or head of the patient, use the lowest flow rates possible, and provide a physical barrier to prevent oxygen‐enriched gases from encountering the laser beam. The risk of fire hazard during laser procedures can be minimized by choosing injectable anesthesia techniques, allowing spontaneous ventilation with room air or, in the case of laryngeal surgery, placing the ETT nasotracheally or through a tracheostomy [2,10,11]. Inspired oxygen concentrations may be lowered by mixing with medical gas if available. Nitrous oxide should be avoided. It may be necessary to remove the ETT and the oxygen source temporarily at several points during a procedure to facilitate safe access to the laryngeal area. To further reduce risk, avoid the use of flammable antiseptic solutions, drying agents, oil‐based lubricants or ointments, or flammable plastics whenever lasers are being used [2,7,20]. Fig. 4.2 is a crisis checklist of emergent measures if an airway or operating room fire occurs. Emergency procedures should be determined in advance and practiced by all potential participants. Laser–tissue interactions produce a plume of smoke that may contain bacterial and viral particles as small as 0.1–0.3 μm and potentially toxic gases, some of which may be carcinogens. Bronchospasm from bronchial irritation, alveolar edema, interstitial pneumonia, and diffuse pulmonary atelectasis are potential consequences of inhaled laser smoke [2,3]. Air evacuation systems are used to reduce pollution of the workspace and prevent inhalation and alveolar exposure of personnel in the laser‐designated area. Laser filtration surgical face masks are available for added protection. Standard surgical masks alone do not provide adequate protection during laser procedures [3]. Patients who are intubated and connected to an anesthesia machine are not at risk for inhaling laser smoke. However, precautions should be taken for non‐intubated patients to prevent inhalation exposure and potential pulmonary injury. Plain radiography, contrast studies, fluoroscopy, computed tomography (CT), and radiation therapy (RT) all incorporate the use of ionizing radiation. Ionizing radiation exposure is potentially hazardous and causes injury at the cellular level by transferring high levels of energy into atoms and molecules such as DNA, RNA, and other cellular proteins, damaging chemical bonds, and altering the electrical charge to create free radicals or ions [21–24]. Sensitivity to ionizing radiation is highest in the most actively replicating cells of the skin, bone marrow, small intestine, and reproductive cells. Developing embryos are particularly sensitive to the effects of ionizing radiation. Slow growing nerve and muscle cells are the most resistant to radiation damage [21–24]. Effects from the exposure to ionizing radiation may appear promptly or be delayed, depending on the type and duration of exposure. Effects resulting from chronic exposure to low levels of radiation are most relevant to veterinary anesthesia providers. Chronic radiation exposure is associated with an increased incidence of cataracts, squamous cell carcinoma, leukemia, and premature aging [21–25]. Regulations require that personnel limit the level of radiation exposure to “as low as reasonably achievable” (ALARA). This can be achieved by increasing distance from the source, using shielding, and minimizing the number of images and length of time of procedures. The primary source of personnel occupational exposure is from X‐rays that bounce off or scatter from objects in the path of the primary beam. The patient is the major source of scatter radiation. The amount of potential exposure from scatter radiation is directly related to the proximity of personnel to the source of the ionizing radiation and decreases rapidly with increased separation from the source. The best way to reduce exposure is to temporarily leave the radiology suite and view the patient from the doorway or through leaded glass when the machine is in use. When leaving the room is not possible, standing at least 3 feet away from the patient during X‐ray use and wearing a protective lead apron are advised [22,25]. An anesthesia provider may not be able to move away from the patient when involved with positioning patients for radiographs or during fluoroscopy and other special procedures. When this is the case, avoid direct beam exposure and avoid leaning over the patient during imaging. When in close proximity to the source of radiation, protective attire should include a lead apron, thyroid shield, and gloves. A wrap‐around lead apron is recommended for situations that may result in a high level of radiation exposure such as fluoroscopy to provide additional protection. Consider wearing protective goggles if a lot of time is spent in the radiology suite. It is important to note that lead‐lined protective apparel is not designed to provide protection from direct beam exposure [22]. The risk of potential radiation exposure is increased with CT and RT due to higher levels of radiation involved in the procedures. During these procedures, it is safest for the anesthetist to leave the side of the patient and stand behind a portable barrier, move into the control room, or leave the room altogether. It is important to monitor cumulative radiation exposure by wearing a radiation exposure badge or dosimeter [22,23,25]. Each individual is provided with their own personal badge to wear at all times during participation in radiographic procedures, and badges should not be worn outside of the radiology suite. It is recommended that radiation badges be positioned at the neckline on the outside of the protective lead apron. Radiation levels are evaluated at scheduled intervals. Pregnant workers should avoid exposure to radiation as much as possible, especially during the first 16 weeks of pregnancy, when the most rapid cell division occurs. It is important to inform management as soon as pregnancy is suspected so that arrangements can be made to only assign the anesthetist to situations that do not involve radiation. Incorporating the safety precautions of decreasing time of exposure, increasing distance from the source, and using shielding as much as possible will reduce exposure. Wearing two aprons or adding a flap to protect the fetus will increase protection. A separate radiation dosimeter, worn at the waistline under the apron(s), is recommended to monitor fetal exposure. Self‐reading dosimeters are available that provide real‐time measurements to further assure protection. The gestational radiation limit is 10% of the normal limit for radiation workers. Radiology suites are rarely designed with the provision of anesthesia in mind. Therefore, in order to provide optimal anesthesia support for every patient during radiology procedures, communication with radiology personnel is critical. It is important to understand the procedure being done, the contrast agents to be administered, the route and rate of contrast administration, and the estimated duration of the procedure. The signalment and temperament of the patient, the underlying disease process, accessibility of positioning devices, familiarity with the procedure being done, anesthetic drug and equipment availability, and amount of assistance are all factors to consider. Standard radiographs may be obtained easily with positioning devices without chemical restraint when the patient is cooperative. However, not all patients are cooperative and sedation will facilitate good positioning and reduce the need for repeat X‐ray exposure for both patients and personnel. Longer duration procedures or those that may be invasive or painful (e.g., certain contrast studies and fluoroscopy) are best done using sedation or general anesthesia. The anesthetist must ensure access to the patient for monitoring and provision of thermoregulatory support, both of which must be achieved without interfering with the imaging procedure or risking radiation exposure. Patients immobilized for radiographic procedures, even when not intubated or under general anesthesia, should be monitored closely and continuously. Monitoring should, at the very least, consist of direct observation of respiratory rate and effort, evaluation of mucous membrane color, palpation of pulse quality and rate, and the use of pulse oximetry and non‐invasive blood pressure. Select monitors with easy‐to‐read screens, large numbers, and visual alarms that can be easily seen in a dimly lit radiology suite from behind a screen or through an observation window. A source of supplemental oxygen should be available at all times. Be prepared to supplement sedation, intubate, support ventilation, induce general anesthesia, or perform rescue procedures when necessary. Position the anesthesia machine and monitors, so they can be easily observed from outside the room or with a video camera. Controlled ventilation is advisable for ensuring adequate ventilation and consistent inhalant anesthesia delivery in patients during procedures such as fluoroscopy, CT, and RT when the anesthetist must leave the room. CT uses high levels of radiation to obtain cross‐sectional images and requires a motionless subject for best results. The imaging time varies depending on the type of CT being used (i.e., 1‐slice versus 64‐slice). While the actual imaging time may be short, the time to position the patient and evaluate the study can be much longer. Patients experiencing pain may not be willing to lie still or allow positioning with sedation alone. Even slight movement during the scan can result in motion artifacts that interfere with the quality of the images and their diagnostic value. If this occurs, the scan will have to be repeated, which increases the overall anesthesia time and the amount of radiation exposure for the patient. Figure 4.3 Cross‐sectional CT image of a dog’s neck and trachea showing artifact created by the radio‐opaque markers embedded in the wall of many common endotracheal tubes. The artifacts are the white lines radiating outward from the markers in the tube wall situated within the trachea. The radio‐opaque stripe in most commonly used ETTs that makes the tube clearly visible on radiographs causes an artifact on CT images. These artifacts may interfere with the diagnostic value of images of the neck and head (Fig. 4.3). ETTs without the X‐ray opaque stripe are available and should be considered when the ETT will be in the area being imaged. Additionally, armored ETTs (reinforced by metal coils) should be avoided if the tube will be included in the imaging area to avoid artifact and the need for repeated scanning. During CT imaging, the presence of metal objects, such as electrocardiogram (ECG) clips and lead wires, in the scan field can lead to severe streaking artifacts that can obscure or simulate pathology. To avoid interfering with image acquisition, keep ECG leads, other monitoring cables, and any metal‐containing item outside the anatomic scan field whenever possible. Valuable cardiac rhythm information is obtainable even when ECG leads are placed at non‐traditional sites, such as the paws, ears, neck, etc. It may be necessary to disconnect one of the ECG leads temporarily in order to avoid creating artifacts on the images. Collars, halters, horseshoes, nail fragments, or other metal objects should be removed from the patient if they are included in the area being imaged. During CT examination, the patient table will move in and out of the bore of the scanner. The anesthesia delivery hoses must be long enough to move with the patient during imaging without becoming kinked or disconnected. Monitoring cables and fluid administration lines should be positioned so that they will not get caught under the moving table or disconnected from the patient. Thoracic CT imaging, particularly of the lungs, is best done immediately following induction to minimize atelectasis. Maintaining the patient in sternal recumbency may decrease positional atelectasis [personal communication: Ian D. Robertson, College of Veterinary Medicine, North Carolina State University]. Some thoracic studies may require apnea or breath‐hold maneuvers to alleviate motion from chest expansion during ventilation. A brief period of apnea may be induced with hypnotic agents (propofol or alfaxalone) or by hyperventilation. Some facilities employ jet ventilation combined with total intravenous anesthesia (TIVA) to avoid respiratory motion. If lung aeration is required, the anesthetist can manually hold the lungs in inspiration during scanning or perform an alveolar recruitment maneuver. In this situation, the anesthetist should wear a protective apron, thyroid shield, and goggles while tucked behind a mobile shield. Alternatively, an anesthetic machine equipped with a positive end‐expiratory pressure (PEEP) valve or a closed adjustable pressure‐limiting (pop‐off) valve with oxygen flow turned off may allow the anesthetist to temporarily depart the scanning room. Caution is advised with this method and strict, constant attentiveness is necessary to avoid catastrophic barotrauma. RT uses a high dose of ionizing radiation delivered precisely by a direct beam into tumor tissue. The total treatment dose is divided into multiple sessions that are spread out over days or weeks. Patients must be motionless and positioned exactly the same for each treatment to prevent damage to surrounding healthy tissue. A variety of devices are used to aid in positioning patients depending on the location of the area being imaged. Certain positioning devices may interfere with anesthetic and monitoring equipment or influence placement of the intravenous (IV) catheter. Effective and safe anesthetic patient management depends on familiarity with the procedure and the specific device being used. All personnel must leave the room for several minutes during treatment. Patients can be observed from outside the room using a video camera(s), preferably with sound, positioned to provide a clear view of the patient monitor, the anesthesia machine, and the patient. The treatment session can be paused if patient movement or problems are detected. General anesthesia is recommended for horses and other large animals during selected radiographic studies and CT scanning to provide the necessary immobility to obtain diagnostic images quickly and safely. Inadequately immobilized large animals may cause damage to the equipment or injury to themselves. Ropes and positioning pads should be available, and physical effort is required to move the table and patient. Horses with neurologic disease may present a higher risk to staff and themselves, particularly during induction and recovery. Standing sedated CT of the equine head or distal limbs is an option at some facilities. Although the risk of general anesthesia is eliminated, a sedated neurologic or orthopedically unsound horse may fall and injure itself, staff, or equipment. Consequently, non‐slip flooring is advised, and the handler should have an escape route planned. Additionally, a handler may remain horse‐side and that individual should be positioned as far as possible from the radiation source behind a mobile shield and wear an apron, thyroid shield, and goggles. Sandbags, a rope halter, and a V‐shaped trough to aid positioning, in addition to ear plugs and a blindfold to limit patient stimulation, may permit the assistant more distance from the radiation source. Radiographic myelography of equids presents several challenges for the anesthetist in addition to radiation safety techniques. The study should be performed quickly to reduce recumbency time and limit time‐dependent reabsorption and dilution of contrast media that may limit the study’s diagnostic value. Horses presenting for myelography may have neurologic deficits, including ataxia, resulting in additional danger to the animal and staff throughout induction and recovery. Adverse reactions associated with anesthesia or myelographic technique that present danger to staff and patient, such as generalized or focal seizures, may occur during the procedure or at recovery. The anesthetist should be prepared to provide medical management or to re‐anesthetize patients when indicated. Multiple position changes of equine patients require manpower and efficient coordination with all services involved. When spinal compression is suspected, careful manipulation of the patient is crucial to avoid exacerbation of any dynamic component to the compression. Constant vigilance to protect the eye, IV catheter, fluid line, anesthetic, and monitoring equipment while ensuring ETT safety and patency are essential. Positional changes and disconnection of the breathing circuit may result in personnel exposure to inhalant anesthetic. Attention to limb positioning and padding and support are essential. Radiolucent cushions should be available to aid neck positioning for image acquisitions to avoid repeat imaging. Inadequate padding under the patient when placed on the floor or myelogram table may increase the risk of developing myopathy or peripheral neuropathy. Radiocontrast agents are used to improve the visibility of certain structures during radiography and CT. The most common contrast agents used for radiographic procedures are iodine‐ and barium‐based preparations [26]. Barium compounds, used for gastrointestinal studies and given orally, do not usually require general anesthesia in companion animals, but it may be necessary for uncooperative, untamed, or exotic species. Prevention of barium aspiration is a critical part of anesthesia management during gastrointestinal studies, as it can cause serious health effects, ranging from pneumonia to death [26]. Protection of the airway with an appropriately sized ETT and inflated cuff and thorough inspection of the back of the mouth prior to extubation for any signs of barium regurgitation are important. If aspiration of barium is suspected, a thoracic radiograph will be able to identify barium in the lower airways and pulmonary parenchyma. The ETT should not be removed until the patient is awake enough to swallow and cough. Consideration can be given to leaving air in the ETT cuff during extubation in case of unobserved leakage of barium into the trachea proximal to the inflated cuff, though this must be weighed against the risk of potential laryngeal damage. Iodine‐based solutions can be administered orally, intravenously, intraluminally, or into the subarachnoid space. Adverse side effects are infrequent and depend on the site of administration and the type of contrast media used. Contrast reactions are related to the osmolality, molecular size, and complexity of the agent used. Effects range from minor physiological alterations to bronchospasm, renal insufficiency, and life‐threatening anaphylaxis [26]. Contrast induced nephropathy is a poorly understood condition that is increasingly reported in people with pre‐existing renal insufficiency and diabetes [26,27]. Studies in dehydrated dogs have shown reductions in renal blood flow and glomerular filtration rate following parenteral administration of hypertonic contrast media. Prior to administration of contrast agents, evaluate renal and hydration status, correct hypotension, and communicate any abnormalities to the radiologist to prevent possible renal impairment [personal communication: Ian D. Robertson, College of Veterinary Medicine, North Carolina State University]. Reduction in renal function due to contrast agents can usually be reversed with correction of hypotension and rehydration [27]. Myelography is a contrast study using plain radiography or CT scanning, consisting of a subarachnoid injection of a water‐soluble, non‐ionic contrast media either in either the lumbar area or cerebellomedullary cistern. This is an invasive and stimulating procedure, and the patient must be adequately anesthetized prior to the insertion of the needle. Patient movement can result in spinal cord damage or a non‐diagnostic study. Side effects following subarachnoid injection depend on the agent selected, the site of administration, and needle placement. They include tachycardia, bradycardia, vomiting, seizures, and cardiac arrest [28,29]. Asystole that responded to anticholinergic administration was reported in one dog immediately after a cisternal injection of iohexol during a myelogram [30]. Postmyelographic side effects have become less frequent with the development of newer contrast agents. If an adverse reaction occurs, treatment is palliative and supportive. In addition to ensuring an adequate airway and providing oxygen and hemodynamic support, the anesthetist may need to manage anaphylaxis and bronchospasm with antihistamines, epinephrine, glucocorticoids, bronchodilators, vasopressors, or anticholinergics. Extravasation of contrast agents may lead to local tissue damage. The severity of the effects caused by perivascular injection depends on the osmolality of the contrast agent and the volume injected and can range from mild swelling and erythema to ulceration and necrosis [26]. Some radiographic studies require the rapid injection of the contrast agent by hand injection or using a rapid injector pump at flow rates of 0.5–5 mL/s. Large volumes of contrast can be injected subcutaneously if the catheter is not properly placed. Box 4.2 lists steps to reduce the risk of extravasation as well as methods to treat accidental perivascular delivery of a contrast agent. Radiology facilities are often separated from the anesthesia induction area, sometimes on a different floor or in a separate building. Advance planning for transportation to and from the facility, including consideration of monitoring and anesthesia maintenance during transfer, is critical for patient safety. In addition to diligent planning, practice drills for emergent situations in sites distant from the anesthesia area are recommended. In facilities where a rapid return to the anesthesia or intensive care area is not practical, rescue drugs and equipment will need to be transported with the patient or in place in the radiology suite.
4
Safety Considerations for Laser and Radiographic Procedures and Magnetic Resonance Imaging
Introduction
Laser
Laser beam hazard and safety
Laser type
Wavelength (nanometers)
Optical density (OD) number
Carbon dioxide
10,600
6+
Nd:YAG
1064
6+
Ho:YAG
2100
4+
Diode laser
810, 980
5+
KTP
532
6+
Argon
488, 514
6+
Laser fire hazard and safety
Anesthetic considerations for laser surgery
Non‐beam hazards and safety
Radiography
Ionizing radiation hazards and safety
Anesthetic considerations for radiography
Radiocontrast agents
Remote facilities
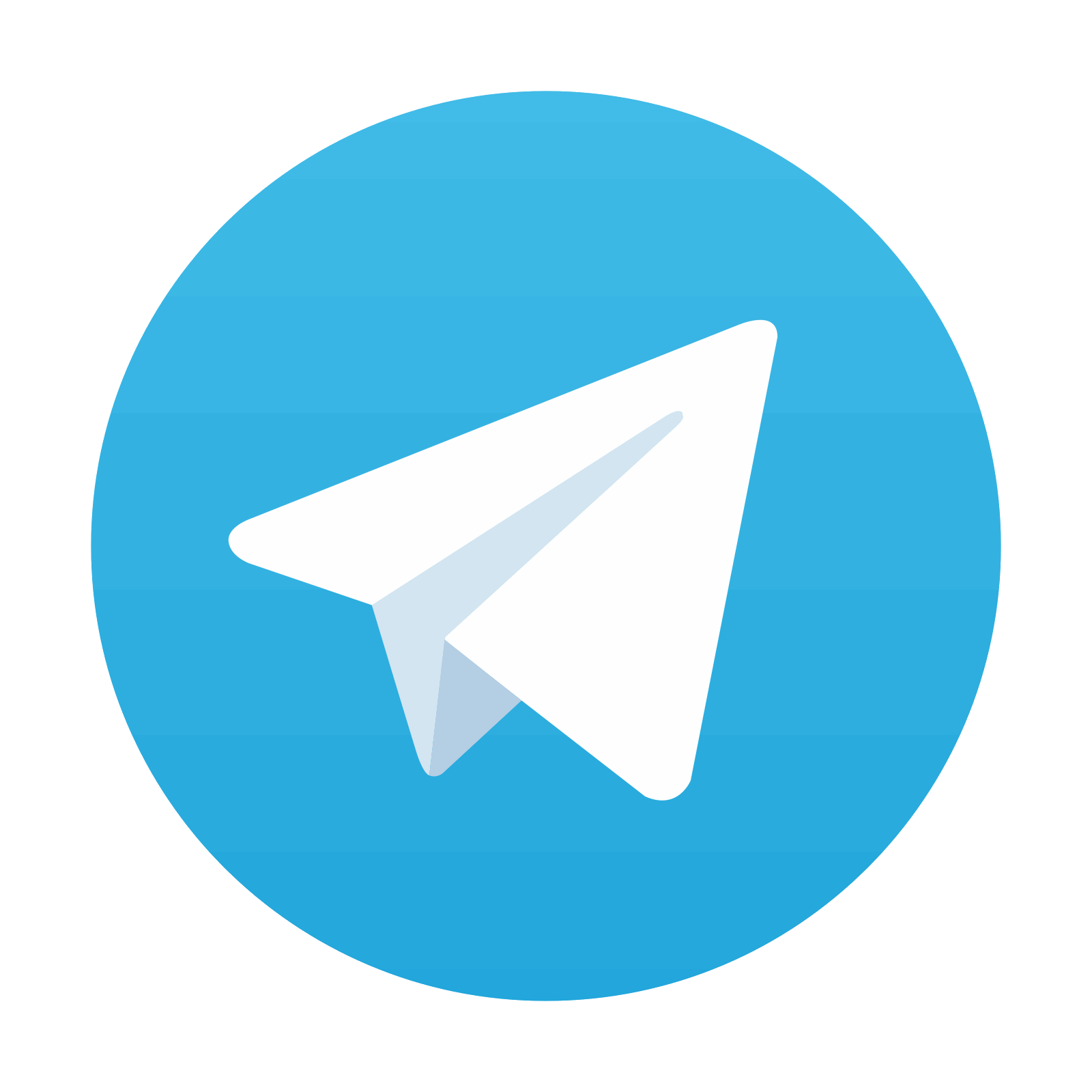
Stay updated, free articles. Join our Telegram channel

Full access? Get Clinical Tree
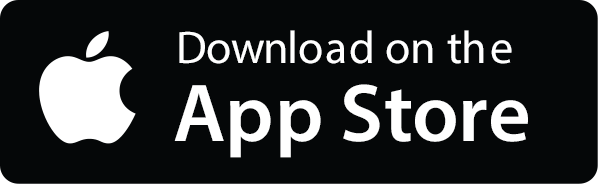
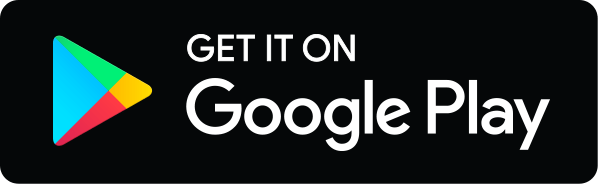