Fig. 1
Left eye of the dog with chronic quantitative tear deficiency. Note hyperemia and thick discharges, vascularized and opaque cornea (chronic keratitis)
The cornea is a highly differentiated tissue that allows the refraction and transmission of light. It is basically a concave–convex lens with an anterior face in close contact with the precorneal tear and a posterior face covered with aqueous humor. Because this liquid is mainly responsible for maintaining the physiological requirements of the cornea, the cornea lacks vascularization. It is composed of a stratified squamous nonkeratinized epithelium, a stroma of connective tissue, and an endothelial cell monolayer. Although this nonvascular tissue is apparently simple in its composition, the great regularity and uniformity of its structure allows the accurate transmission and refraction of light [13]. It possesses effective low molecular weight antioxidants (such as ascorbic acid, glutathione, and alpha tocopherol) [14–18] and high molecular weight antioxidant enzymes (such as superoxide dismutase, glutathione peroxidase and reductase, and catalase) [19–21]. Works carried out by Kovaceva et al. have shown differences between the concentration of antioxidants in the corneal epithelium of animals with daily activity and that of animals with nocturnal behavior; these differences are considered due to the exposure of diurnal animals to UV rays [22].
Due to its location, the cornea is constantly exposed to the effects of radiation, atmospheric oxygen, environmental chemicals or pollutants, and physical factors. These external agents can cause an oxidative imbalance in the cornea. Corneal inflammatory processes (keratitis), which have a high diagnostic frequency (Fig. 2), can also produce this alteration.
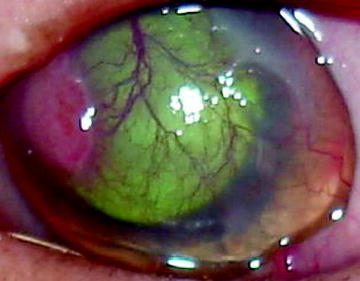
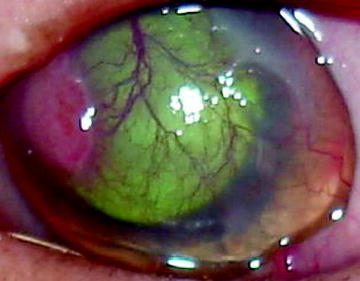
Fig. 2
Keratitis affected left eye. The superficial cornea is both infiltrated and vascularized
Cells in the epithelium and corneal stroma are capable of producing superoxide via NADPH oxidase [23]. The superoxide thus produced potentially contributes to the processes that occur during corneal inflammation. This process involves neutrophil infiltration, which may obviate ROS production by the membrane-bound NADPH oxidase system and cytoplasmic granule myeloperoxidases [12, 24]. As suggested by Chandler et al. this process can be increased by exposure to UV, which causes overexpression of matrix metalloproteinases that are observed with changes in the cornea that allow an influx of inflammatory cells and vascularization [25].
Oxidative stress can alter the natural equilibrium between proteinases and proteinase inhibitors in favor of the former, engendering pathological degradation of collagen and proteoglycans within the corneal stromal extracellular matrix [26].
Independently of the cause, the deficit of antioxidant agents in the ocular surface, both in tears as in the cornea, is extremely important because the ocular surface is the mucosal surface most environmentally exposed to the effects of external agents [27–29] which exacerbate the production of ROS and free radicals, thus increasing the oxidative injury [24].
Therefore, it would be interesting to find the association between the treatment of the primary cause of the disease and antioxidant agents in some lesions of the ocular surface, in order to minimize the alterations of the oxidative disbalance and thus maintain corneal transparency.
Uveitis
The inflammation of the uveal tract (iris, ciliary body, and choroid), known as uveitis, is the most frequently diagnosed disease in this tissue, in both dogs and cats. The process can affect different structures that form the uveal tract or the uvea as a whole. In veterinary medicine, there are numerous causes that can trigger uveitis, ranging from local injuries to systemic diseases (Table 1) [30]. The alteration in visual function in patients with uveitis is caused by different factors, one of which is the damage caused by infiltration of inflammatory cells. The disruption of the blood barrier resulting in protein extravasation and cellular infiltration into the aqueous humor and anterior chamber is clinically called Tynall Effect (scattering of particles in acolloid medium), and if the process allows an excessive increase in cells deposited in the anterior chamber it is called hypopyon, and changes in the metabolism of corneal endothelium that are observed with loss of corneal transparency as a result of edema (Fig. 3) [31]. The inflammatory cells accumulated in the uveal tract, mainly mononuclear and polymorphonuclear phagocytes (PMNs), generate ROS. All these processes are related to an increase in cytokines, metabolites of arachidonic acid (eicosatetraenoic acid), nitric oxide (NO), including radicals, such as superoxide anion (O2·−) and hydroxyl radical (HO·) and nonradicals, such as hydrogen peroxide (H2O2) and singlet oxygen (1O2). Different studies showed that the cytokines, tumor necrosis factor alpha (TNFα), and several types of interleukins enhance the inflammatory response in both the aqueous humor and uveal tissue [32, 33]. ROS play a key role in the development of uveitis, because these can increase vascular permeability, damage to extracellular matrix proteins, enhance cytokine expression, activate endothelial cells, increase adhesion molecule expression, and increase the formation of chemotactic factors [34]. Superoxide and hydrogen peroxide are among the most important primary species generated by these phagocytes, This process, and other free radicals are formed and are associated with tissue damage. Although H2O2 is a strong oxidant, its reaction with organic compounds is low. In the presence of H2O2, the myeloperoxidase released by phagocytes catalyzes the formation of hypochlorous acid. This acid reacts with other metabolites derived from O2− to form HO·, these hydroxyl radicals have been implicated as the ultimate cytotoxic agent because of their high reactivity, including peroxidation of membrane lipids [35].
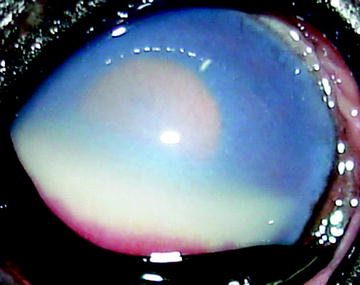
Table 1
Uveitis triggers
Exogenous | Endogenous |
---|---|
Trauma | Infections (viruses, fungi, and bacteria) |
Corneal ulcers | Parasites |
Deep wounds | Cataracts |
Neoplasia | |
Immune mediation |
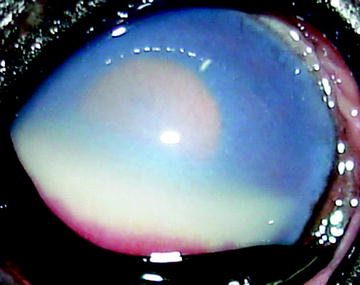
Fig. 3
Anterior acute uveitis in a dog with lymphosarcoma. Note severe corneal edema, hipopyon is limited to the lower anterior chamber and miosis
On the other hand, it has been shown that activated phagocytes synthesize considerable amounts of NO, which is the oxidation product of one of the guanidino-nitrogens of L-arginine. The reaction is catalyzed by two different forms of the enzyme NO synthase (NOS) [32]. Park et al. showed that the IL-1, TNF-ά, and IFN-γ are directly implicated in the production of NO [36]. The reaction between superoxide and nitric oxide proceeds with near diffusion-limited rates, yielding peroxynitrite (ONOO−), and is the most potent biological oxidant obtained from NO and O2 [33]. Physiologically, the uvea possesses a high metabolic aerobic activity with a consequent production of O2– and H2O2. However, diverse intracellular enzymatic systems prevent the accumulation of metabolic waste highly toxic for the eye.
The inflammatory process of the choroid (choroiditis) usually involves the retina, producing chorioretinitis, due to the close relationship between both tissues (Fig. 4). The retina is a structure particularly sensitive to oxidative damage because of its high consumption of O2 and its high percentage of polyunsaturated fatty acids (PUFAs) [37] which are required by retinal membranes to build up a highly fluid microenvironment for the rhodopsin visual pigment that is responsible for the light signal transduction and its direct exposure to light [38]. Free radicals may trigger the initiation of lipid peroxidation (LPO) of PUFAs, leading to the release of lipoperoxyl radicals that induce oxidative stress, which is closely related to photoreceptor membrane lysis. Vision can be seriously affected by oxidative stress damage to the retinal membranes.
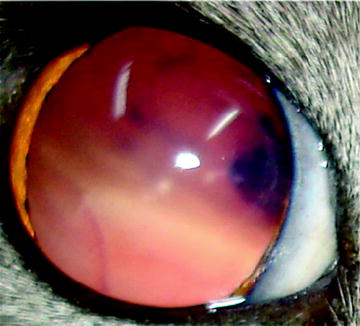
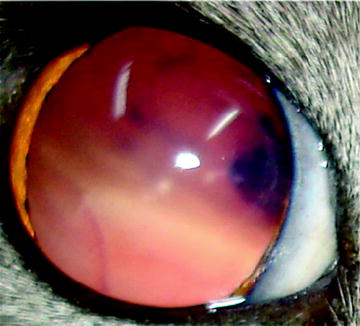
Fig. 4
Complete bullous retinal detachment in this domestic shorthair with advanced chorioretinitis
Special attention has been recently devoted to ROS as factors that initiate the retinal degeneration induced by inflammation. In fact, in experimental uveitis, all the products of LPO, as well as a high concentration of peroxynitrites (ONOO−), have been identified [39].
The aim of the therapy of the inflammatory processes of the uveal tract is based on preventing the development of the inflammation with steroid and nonsteroid anti-inflammatory drugs. A large number of research works have shown the use of antioxidants and their positive effects in the anti-inflammatory processes.
Glaucoma
The regulation of the intraocular pressure (IOP) depends on the balance of the complex mechanisms involved in the production and drainage of the aqueous humor. The aqueous humor is generated and evacuates constantly and in equilibrium in the structures of the anterior segment of the eye, for its own nutrition and the maintenance of the ocular tone [40]. Glaucoma is an optic neuropathy, characterized by the progressive degeneration of retinal ganglion cells (RGCs) associated with an elevated IOP and visual field damage, representing the final stage caused by a number of different conditions affecting the eye. In dogs, glaucoma has an estimated prevalence of 1.8% [41], whereas in cats it is often secondary to other ocular diseases [42]. In dogs, the most frequently diagnosed glaucoma is closed-angle glaucoma, which is characterized by a degeneration of the optic nerve head and a progressive deterioration of the visual function, in spite of the decrease observed in the IOP, which suggests the existence of other factors producing optic neuropathy (Fig. 5) [43]. Although it is considered that the increase in the IOP is the most important risk factor (neurodegeneration) of the glaucoma, there are several associated factors such as increases in the levels of glutamate [44], the metabolic alteration of nitric oxide [45], vascular factors (ischemia) [46], and oxidative damage caused by overproduction of ROS [47].
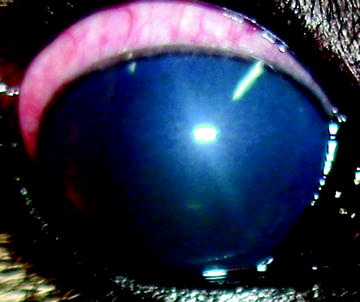
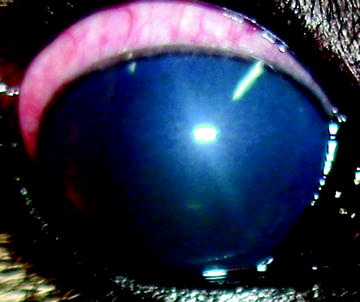
Fig. 5
Early state primary glaucoma. Both corneal edema and mydriasis are clinicals signs
Several works have shown that oxidative DNA damage in the cells that compose the trabecular meshwork is associated with an alteration in the structure of the extracellular matrix, which produces a decrease in the drainage of the aqueous humor and an increase in the IOP because of its constant production [48, 49]. Weinstein et al. have demonstrated an increase in active matrix metalloproteinases in the iridocorneal angle tissue from glaucomatous eyes when compared to normal eyes [50]. Alterations in the irrigation (ischemia) of the retina and the optic nerve increase the levels of glutamate in the vitreous, which in turn produces cellular degeneration of the retina caused by excitotoxic damage to the RGCs through activation of ionotropic and metabotropic glutamate receptors [51–53]. Activation of glutamate receptors is thought to initiate damage in the retina by a cascade of biochemical effects such as activation of neuronal nitric oxide synthase (nNOS) and increase in intracellular Ca2+. Glutamate has been described as a major contributing factor to the loss of RGCs.
Several works have associated the increase in endothelin-1 with the etiology of glaucoma. An increase in this endogenous vasoconstrictor is related to ischemia, which can exacerbate the neurodegeneration by increasing the levels of glutamate [54]. Nitric oxide plays a significant role in the pathophysiology of glaucoma, because an increase in its synthesis has been associated with a decrease in the number of RGCs. The uncontrolled increase in NO may lead to significant oxidative stress, as NO couples with the superoxide anion radical (O2·−) to generate the highly reactive and destructive peroxynitrite anion (ONOO·−). Indeed, there is certainly ample evidence documenting the toxicity of NO in neural tissue [45]. Gionfriddo et al. have shown that the intracellular antioxidant glutathione is decreased in the glaucomatous eye [55]. Finally, several authors have shown the processes by which oxidative stress plays its role in the pathogenesis of glaucoma and have allowed us to gain insights into the molecular mechanisms involved in the physiopathology of glaucoma and the later degeneration of the retina.
Cataract
The crystalline is a transparent, soft, intraocular biconvex lens. The fiber cells are filled with high concentrations of crystallines, the proteins that provide a uniform refractive index to the fiber and minimize light scatter. All these components are inside an elastic capsule, which conditions the molecular transport with the aqueous humor and supports the insertion of the zonular fibers [56]. In its equatorial region, the lens has a layer of epithelial cells that terminally differentiate into fiber cells. These cells elongate toward both the anterior and the posterior poles. The newly formed fiber cells develop over the older fibers and displace them toward the center of the tissue. Cataract is an opacity of the lens of the eye. There are many distinct types of cataract defined on the basis of the section of the lens that has become opaque (Fig. 6) [57]. This alteration affects mainly canines, especially certain breeds [58], and is one of the main causes of blindness in this species. Park et al. have demonstrated that the age of onset and gender distribution of cataracts in small-breed dogs are different depending on the breed [59]. Cataracts in different dog breeds can be of genetic, metabolic, toxic, or traumatic origin, or due to senility, environmental factors and other causes (Figs. 7 and 8) [60–62].
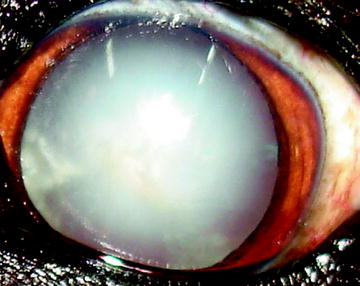
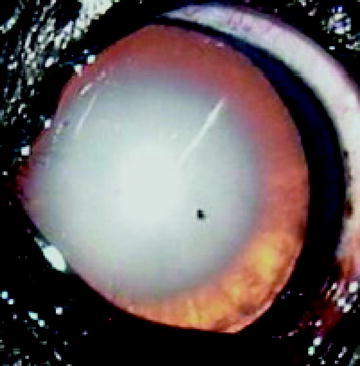
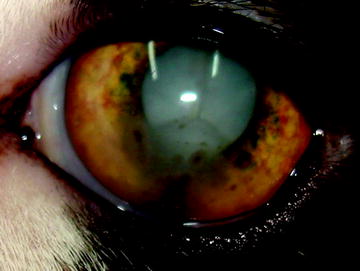
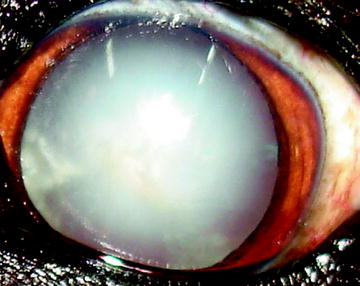
Fig. 6
Typical appearance of a mature or complete cataract
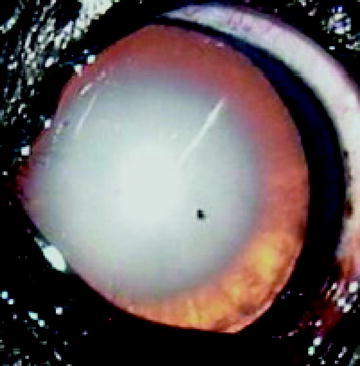
Fig. 7
Typical age-related cataract in a 13-years-old English Cocker Spaniel. Note the dense nuclear sclerosis
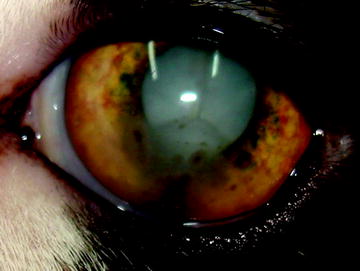
Fig. 8
Toxoplasma-induced anterior uveitis, as in this domestic shorthair, is characterized by iridal nodules, keratic precipitates and cataract
Cataracts originate from modifications in the uniformity of the protein density of the crystalline, which produces changes in its refraction index and is manifested by its opacity. Although the biochemical events that lead to these modifications are not well understood yet, several researchers have found that in this disorder insoluble proteins are increased and that the insolubilization process is accelerated. It seems that this insolubilization is produced by the formation of covalent bonds as a result of protein oxidation. The sulfur-containing amino acids present in the proteins are usually very susceptible to oxidative damage, generating disulfides that can cause the establishment of double bridges between proteins or subunits and the formation of aggregates [63]. The crystalline has a high concentration of reduced glutathione, which maintains the thiol groups reduced, which in turn contributes to its complete transparency.
Ultraviolet (UV) radiation reaches the crystalline lens directly, where it generates free radicals that damage essential molecules. This situation provides favorable conditions for the induction of ocular destruction by ROS and reactive nitrogen species [64].
The exact mechanism leading to cataract formation is still largely unknown [65]. The lens epithelium plays an important role in the regulation of nutrient and ion transport to all cells in the lens, and actively participates in adaptive responses to environmental and chemical stressors [66]. Oxidative stress is currently no longer the hypothesis for the development of cataracts, but it is known that it not only causes protein damage and precipitation in lens fibers, but also contributes to the dysfunction and apoptotic death of lens epithelial cells [67–69].
McCarty et al. and Spector have demonstrated that the damage to the lens epithelium caused by UV radiation or oxidative stress (i.e., an increase in H2O2) represents an initiating event in cataract formation, emphasizing the importance of maintaining the integrity of the epithelial layer [70, 71]. Wu et al. have recently reported that sustained oxidative stress impairs the cell signaling involved in NF-κB activation, which increases the death of the lens epithelial cells and exacerbates cataract genesis [72].
The lens, as well as other tissues, has an antioxidant system and high levels of glutathione. In patients with cataracts, these levels are lower than normal [73]. This is in agreement with other studies that have reported an increase in ROS and other free radicals in the aqueous humor both in patients with cataracts and in cell cultures of lens epithelium [74]. The oxidative stress associated with increased levels of ROS is known to accelerate cataract formation in laboratory animal models [75]. Another antioxidant agent is superoxide dismutase. Dingbo et al. demonstrated an increase in the expression of this antioxidant agent in lens epithelial cells when stimulating them with H2O2 [76].
On the other hand, Babizhayen et al. showed that the initial stages of cataract are characterized by the accumulation of primary LPO products and a reliable increase in oxiproducts of fatty acyl content of lenticular lipids. The lens opacity degree correlates with the level of LPO fluorescent end-product accumulation in the lens, which is associated with sulfhydryl group oxidation of lens proteins due to a decrease in reduced glutathione concentration in the lens [77]. In most tissues of the body, including the lens, superoxide is converted into hydrogen peroxide by superoxide dismutase, but even hydrogen peroxide can become highly toxic because it produces the hydroxyl radical OH· This toxicity is prevented by catalase and glutathione peroxidase. These enzymes protect the lens by a system of antioxidant molecules, the lynchpin of which is glutathione, and the role of which has been expertly reviewed by Marjorie Lou [78].
Surgery (phacoemulsification and aspiration) is the main therapeutic tool against cataracts. However, adverse effects, such as corneal epithelium damage, mechanical and thermal damage due to the procedure, and formation of free radicals due to the effect of ultrasound (because ultrasound causes the disintegration of water molecules and the consequent formation of hydroxyl radicals) have been described. However, because sodium hyaluronidate is known for its capacity to remove free radicals, it is used for this surgery technique.
Nonsurgical alternatives based on antioxidants, for both the systemic and the topical treatment as well as for the prevention of cataracts, are currently being tested [79–81]. Finally, it is important to point out that some studies with dietary supplements with antioxidants in dogs and human patients with cataracts have shown a decrease in the development of cataracts [82–84].
Retina
The retina is the innermost layer of the eyeball and it is made up of cells with vastly different functions. The retina is composed of two types of photoreceptor cells, rods and cones, that contain a photosensitive pigment called rhodopsin, which allows them to perceive the light stimulus.
The functioning and maintenance of these types of cells require a high consumption of energy: three to four times more oxygen than other cells of the retina and neurons of the central nervous system (CNS). They are probably the cells of the organisms with the highest oxidative metabolism. In as much as they generate adenosine triphosphate mainly from glucose by oxidative metabolism and glycolysis. They are composed of a high percentage of PUFAs, which are required by retinal membranes to build up a highly fluid microenvironment for the rhodopsin visual pigment that is responsible for the light signal transduction. On the other hand, high unsaturation indices make these membranes highly vulnerable to oxidative damage [85].
In the retina, the main sources of ROS are exposure to light, mitochondrial respiration, cellular metabolic reactions such as phagocytosis of outer segment tips by retinal pigment epithelium (RPE) cells, lipofuscin phototoxicity, and protoporphirin photosensitization. From the physiological point of view, ROS are constantly generated in the course of photochemical reactions that convert light energy to chemoelectrical signal [86].
The RPE plays a key role in the functioning and metabolism of photoreceptors. This epithelium controls the volume and composition of fluids in the subretinal space by means of the transport of ions, fluids, and metabolites, and is responsible for the maintenance of the integrity of the RPE–photoreceptor interface. In addition, the RPE plays a key role in the phagocytosis of the external segment of photoreceptors, an important process in the regeneration of the photopigment and the activity of detoxification (antioxidants).
There are different mechanisms by which the retina can suffer alterations in the reduction–oxidation (redox) status. It is known, for instance, that certain wavelengths can cause phototoxicity or photochemical lesion [87]. Short wavelength radiations (the spectrum of rhodopsin) and blue light (absorption peak of 440 nm) have an impact on the function of the pigmentary epithelium and photoreceptors, producing photochemical damage and death by apopotosis [88]. This light energy causes the generation of free radicals in the eye tissues, thus promoting LPO (lipid perioxidation). The neuroretina is extremely sensitive to this process because of its high content of PUFAs, especially the external segment of the cones and rods, which contains a high concentration of 22:6 ω3 (DHA) [89].
< div class='tao-gold-member'>
Only gold members can continue reading. Log In or Register a > to continue
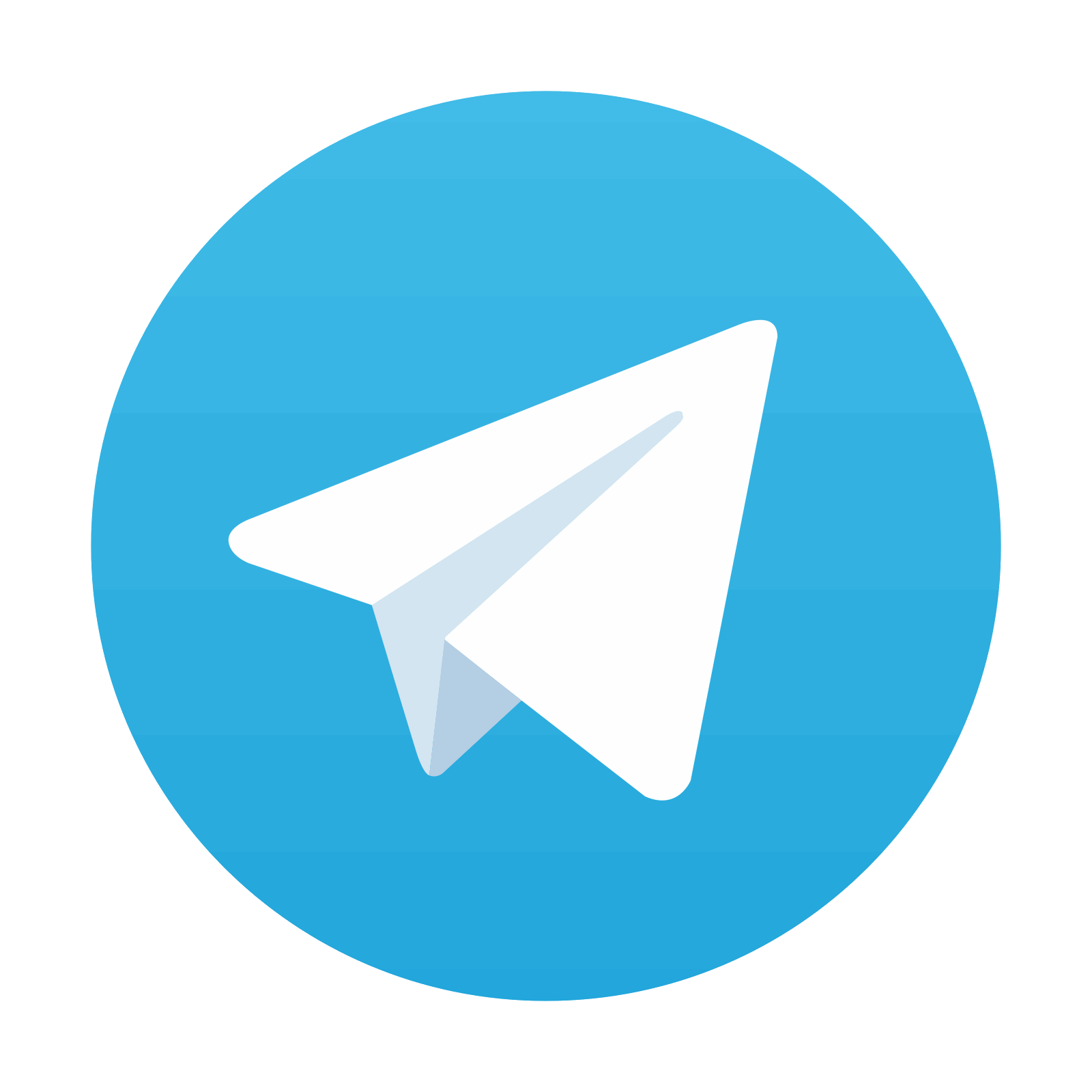
Stay updated, free articles. Join our Telegram channel

Full access? Get Clinical Tree
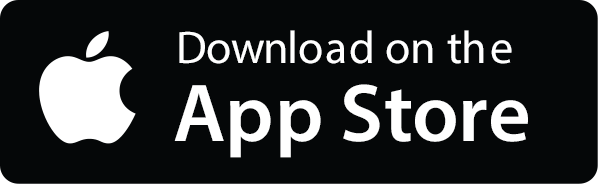
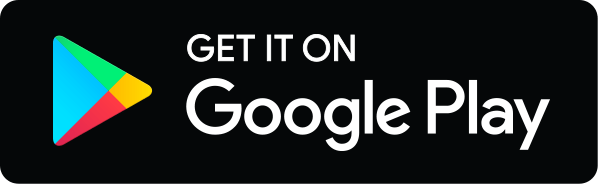