CHAPTER 32 Rhodococcus equi
Rhodococcus equi infection was first described in horses in 1923.1 It is now recognized worldwide as a major cause of disease in foals 3 weeks to 6 months of age. The most common clinical manifestation is pyogranulomatous pneumonia, although a variety of other clinical problems may be identified. The disease has the potential to cause significant losses, especially on farms where it is enzootic. Infrequently, R. equi causes infection in adult horses, generally thought to be associated with immunosuppression. R. equi has been isolated from a wide variety of species, including cats, dogs, goats, cattle, camelids, pigs, crocodiles, and other indigenous animals. Clinical disease is uncommon in these species, and infection is often localized. R. equi is now considered an important pathogen in immunocompromised human patients, particularly those infected with human immunodeficiency virus (HIV).
ETIOLOGY
Rhodococcus equi, previously known as Corynebacterium equi and Mycobacterium equi, is a facultative intracellular bacterium that resides within macrophages.2,3 Rhodococci belong to the family Nocardiaceae, order Actinomycetales. R. equi is a pleomorphic, gram-positive organism with a rod-coccus life cycle.2,4 Depending on growth conditions and the phase of the life cycle, it may appear either coccoid or as long rods or short filaments with rudimentary branching. The organism is aerobic, nonmotile, asporogenous, and partially acid-fast. In vitro, optimal growth occurs at 30° C (86° F) and at a pH between 7.0 and 7.5.3,5
Rhodococcus equi is a member of a unique phylogenetic group within the Actinomycetales, known as the mycolata.2,6 This group contains a number of pathogenic genera in addition to Rhodococcus, including Mycobacterium, Corynebacterium, and Nocardia. The distinguishing feature of the mycolata is their distinct, lipid-rich cell envelope that contains mycolic acids, a large proportion of which are linked to the peptidoglycan-arabinogalactan cell wall polysaccharide and (glyco)lipids. This characteristic cell envelope forms a permeability barrier to hydrophilic compounds, which makes some type of permeability pathway necessary for the bacteria. Two channel-forming proteins, or porins, have been identified in R. equi.7 The unique mycolic acid–containing cell envelope of R. equi is of clinical significance because it is thought to play a role in survival of the bacteria under harsh conditions, such as those within macrophages, and may also influence antibiotic susceptibility patterns.6–8
The numerous different strains of R. equi include both virulent and avirulent variants. Strains may be identified by a variety of characteristics, including the degree of virulence, serotyping, and restriction endonuclease (RE) digestion of genomic and plasmid deoxyribonucleic acid (DNA).9–16 In one study, 44 strains were identified among 209 isolates, with five strains accounting for more than half the isolates.17 It was determined that a small number of strains account for clinical disease, and that in some cases, disease may be caused by simultaneous infection with multiple strains.
Virulent strains of R. equi are characterized by their ability to survive and replicate within macrophages (Fig. 32-1). This ability is associated with the presence of a large virulence plasmid of approximately 80 to 90 kilobases (kb), which was initially identified in isolates from diseased foals.12–14,18 A number of different strains carry the virulence plasmid, and these strains appear to be geographically widespread.17 The DNA from the virulence plasmids of various isolates have been analyzed by RE digestion, and based on the digestion patterns, at least 10 distinct but closely related plasmids have been identified.19–26
The virulence plasmid has been sequenced and contains 69 open reading frames in three functional regions.27 Two of these regions contain genes that are similar to those encoding proteins involved in conjugation and in plasmid replication, stability, and segregation. The presence of genes resembling those required for conjugation suggests that the virulence plasmid may be transferred from virulent to avirulent strains, although this has not been demonstrated. The third region of the virulence plasmid is a 27.5-kb pathogenicity island that encodes seven related virulence-associated proteins (Vaps A, C, D, E, F, G, and H) and four additional extracellular proteins. The first Vap to be identified was VapA, a highly immunogenic surface lipoprotein of 15 to 17 kilodaltons (kDa).12,13,18,28 Of the VapA homologs, all are expressed except for VapF. Vaps C, D, and E are secreted, but unlike VapA, are not anchored to the cell wall.29 The genes of the virulence plasmid show little homology with known genes in other species, making predictions of function difficult and suggesting that R. equi employs novel virulence mechanisms. Two of the proteins that have homologs in other bacteria are transcriptional regulators. Although the precise functions of the Vaps are unknown, it is known that the virulence plasmid expressing VapA is required for pathogenicity in foals.6,14,15 Also, VapA is necessary for the replication of R. equi within macrophages and increases the cytotoxicity of R. equi for murine macrophages.14,30–32
Gene expression by bacteria is often regulated by complex networks that respond to environmental signals. In R. equi, expression of VapA is influenced by environmental conditions, with maximal expression occurring at high temperature (37° C [98.6° F]) and low pH (6.5).33,34 At least five environmental signals, including temperature, pH, oxidative stress, magnesium and iron, contribute to the regulation of gene expression within the pathogenicity island.6,29,35–37 Because the conditions that upregulate expression generally tend to be present when there is bacterial interaction with the host, the pattern of gene expression is consistent with the role of these genes in virulence.
Virtually all clinical isolates of R. equi from horses contain the virulence plasmid and express VapA, but this is not true of isolates from other species.6,15,24,38–41 A second plasmid, 79 to 100 kb in size, has been identified that expresses VapB but not VapA.6,15,42,43 Although this plasmid has not been associated with naturally occurring disease in foals, it can cause disease after experimental inoculation and appears to be of intermediate virulence.44 In isolates from swine, 60% are positive for VapB, whereas other isolates lack a plasmid.45 In human patients affected with R. equi, strains may contain VapA or VapB or may lack a virulence plasmid.42,46 It is unclear if the severely immunocompromised status of the majority of human patients with R. equi allows relatively avirulent organisms to produce disease or if there are distinct, species-specific virulence determinants.
EPIDEMIOLOGY
Rhodococcus equi is widespread in soil samples and in the feces of herbivores, especially horses.47–57 Because R. equi is present on virtually all horse farms, exposure of horses is common worldwide, and the majority of horses have antibodies to R. equi. In soil samples the greatest numbers of organisms are found in surface soil, with almost no bacteria found at a depth of 30 cm (1 foot) or more.56 Rhodococcus equi can be isolated from the feces of adult horses, reaching numbers of 102 to 103/g of feces.50,54–56 This generally represents acquisition from contaminated soil and passive intestinal carriage in adult horses, rather than actual colonization of the intestine.
In foals, R. equi can be first isolated from the feces at about 1 to 2 weeks of age, with most foals becoming positive by 4 weeks of age.54,58 Up to the age of about 3 months, R. equi can actively multiply in the intestine of foals, reaching concentrations of 104 to 105/g of feces or higher and then declining to adult concentrations. Foals with rhodococcal pneumonia often swallow sputum infected with large numbers of virulent organisms, which may then multiply in the intestine, resulting in high numbers in the feces and significant environmental contamination.
Under suitable conditions, R. equi can multiply further in the environment. Rhodococcus equi grows substantially better in soil enriched with feces than in soil alone, and it is hypothesized that the organic acids in manure, such as acetate and propionate, support growth.5 The organism tends to replicate better at a relatively warm temperature and in a neutral soil (pH 7.3) compared with an acidic soil (pH <5.5).5 In addition, decreased soil moisture and decreased pasture cover have been associated with high numbers of environmental R. equi.59 In some cases, a single gram of soil may contain millions of virulent R. equi.50,54–56,60
Inhalation of dust particles containing virulent R. equi is the major route of infection in foals. Experimentally, aerosolized bacteria and intratracheal and intrabronchial inoculation of bacteria in foals can produce pulmonary lesions similar to those of natural infection.61–63 In one study, R. equi was isolated from the air in stalls, with the number of organisms in the air increasing on dry, windy days.55 A study of six farms found poor correlation between the numbers of virulent R. equi in air samples and soil samples on a given farm.59 Although ingestion is a common route of exposure, in most cases it does not result in clinical disease.50,54,64,65 Rarely, R. equi infection is acquired through contamination of a wound.66,67 Once infection is established, R. equi may disseminate to distant sites by hematogenous spread.
Foals are generally exposed to R. equi early in life, but the time when foals actually become infected has not been well established. It has long been thought that the age of onset of clinical disease is associated with the period of waning maternal antibody.50,54,68 However, one study concluded that foals are infected much earlier, during the first several days of life.69 This study was based on a retrospective analysis of the age at onset of clinical signs and the age at death caused by R. equi pneumonia using Sartwell’s model to determine if there was a logarithmic normal distribution consistent with a point source of infection. The interpretation of these data has been controversial, and although it is accepted that foals developing R. equi pneumonia can be infected in the first week of life, it has been proposed that infection is not limited to this period.70 Experimental studies show that foals remain susceptible over a wider age range.44,61–63,70 Currently, no experimental data support the long incubation period (30–90 days) that would be necessary in some cases if all foals that develop rhodococcal pneumonia after natural exposure were infected in the first few days of life.44,61–63,70
Exposure of foals to R. equi is common because the organism is present on most horse farms, but the prevalence of clinical disease is highly variable. On many farms the disease is unrecognized, whereas on others it is either sporadic or enzootic. The prevalence varies widely between farms and years, with rates ranging from 0% to 100%.50,71–76 Many enzootic farms have prevalence rates of 13% to 25%. The mortality rate is also highly variable, with death or euthanasia occurring in 0% to approximately 30% of cases.71,73,76–78
A number of theories have been proposed to explain the difference in prevalence between farms. Although it was initially proposed that the prevalence of rhodococcal pneumonia correlated with the number of R. equi bacteria in soil, this was not supported by soil cultures.49,79–82 Subsequently, it was hypothesized that farms with enzootic disease were more heavily infected with virulent strains of R. equi than those farms where the disease was not present. Although initially supported by Takai et al.,57 other studies have not supported this theory.23,83 Martens et al.83 compared 33 farms with R. equi and 33 farms without a history of R. equi and found no significant associations between disease status and isolation of R. equi from soil or detection of VapA in soil isolates. These findings suggest that it cannot be determined whether foals on a given farm are at increased risk of developing rhodococcal disease based on soil culture and VapA results.
Several epidemiologic studies have assessed risk factors for the development of rhodococcal disease, evaluating variables such as soil geochemistry, breeding-farm characteristics, management and preventive health practices, and foal-related factors.72,84–87 Although study results have varied somewhat, some general risk factors have been identified. Farms with large acreage, large numbers of mares and foals, and a population of transient mares and foals are at high risk for foals developing rhodococcal pneumonia.85,86 High foal density is associated with an increased risk of farms being affected by R. equi in one study, but a subsequent study found no association.85,86 There has been no evidence that poor farm management or lack of attention to preventive health practices contributes to the probability of rhodococcal pneumonia.85,87 The role of housing has been evaluated, but the significance of the findings is unclear. In one study, having concrete floors in the foaling stalls was associated with an increased risk of developing disease, whereas in a second study, housing foals in stalls with dirt floors appeared to increase risk.85,87 The investigators recommend that these results be interpreted with caution until more data are available. One study evaluated soil samples from affected and unaffected farms for multiple factors, including pH, salinity, and nitrate, and found no association between any soil factor and the R. equi disease status of the farms.72 In another study, rhodococcal pneumonia was associated with an environment that participating veterinarians subjectively determined to be moderately to severely dusty.85 One theory currently under investigation is that foals developing rhodococcal pneumonia are born to mares that shed high numbers of organisms in their feces, but as yet, no data support this hypothesis.
Limited studies have attempted to identify host factors of foals that influence the outcome of exposure to R. equi. To identify differences between foals that become affected and those that remain healthy on enzootic farms, Chaffin et al.88 evaluated hematologic and immunophenotypic parameters in foals at 2 and 4 weeks of age before the onset of clinical disease.88 Foals with a CD4/CD8 ratio of less than 3.0 appeared to have a higher risk of developing rhodococcal pneumonia. In addition, the number of segmented neutrophils was lower in foals that subsequently became affected compared with foals that remained healthy. However, the significance of these findings is unclear because the data were confounded by farm-related differences, and there was considerable overlap between values for affected and unaffected foals. Flaminio et al.89 found that absolute and proportional B-cell concentrations were greater in foals with active R. equi pneumonia than in healthy foals of the same age. It has also been suggested that genetic factors may play a role in susceptibility to R. equi, because limited data suggest an association between foal death caused by R. equi and the type of transferrin, an iron-binding protein with differing genotypes and variable bacteriostatic properties.90 Another hypothesis is that infection of foals with equine herpesvirus type 2 (EHV-2) is a predisposing factor for invasion of the respiratory tract by R. equi.91,92 At this time, host factors that influence susceptibility to R. equi in foals are poorly understood.
PATHOGENESIS
Mechanisms of Disease
The infectivity of R. equi is limited to cells of the monocyte/macrophage lineage, and the basis of this organism’s pathogenicity is its ability to replicate in and eventually destroy macrophages.6,15,32 R. equi strains cured of the virulence plasmid are unable to survive and replicate in macrophages and are avirulent for foals and mice.12,13,15,63 Specifically, it has been demonstrated by targeted “knockout” mutants that VapA is necessary for full virulence in mice, as well as for multiplication in ex vivo murine macrophages.30 Thus, although the function of the virulence plasmid is not fully understood, it appears to be essential for full virulence. Defining the precise mechanisms by which rhodococci survive and replicate within macrophages, as well as the role of the virulence plasmid, is key to understanding the pathogenesis of the disease.
The means by which R. equi enters the macrophage may influence survival within the cell. Bacteria interact with a surface receptor on the macrophage and are internalized by phagocytosis, becoming enclosed in a portion of the macrophage plasma membrane, forming a phagosome. The specific phagocytic receptor involved mediates differences in internalization mechanisms and macrophage activation. R. equi appears to bind primarily to macrophages through fixation of complement after activation of the alternative complement pathway, then binding to the macrophage complement receptor type 3 (CR3), also known as Mac-1 (CD11b/CD18).93,94 In general, complement receptor–mediated phagocytosis is not associated with a high level of production of reactive oxygen intermediates and proinflammatory mediators, possibly allowing the organism to avoid antibody-associated macrophage-killing pathways.95 Brumbaugh et al.96 reported that phagocytosis of R. equi by equine macrophages was not associated with a functional respiratory burst. In contrast to complement receptor–mediated phagocytosis, entry into the cell through the Fc receptor after opsonization with specific antibody is associated with significantly enhanced killing of R. equi by equine macrophages.6,97,98 The presence of the virulence plasmid does not appear to influence the uptake of R. equi by macrophages because both virulent and avirulent strains are phagocytosed to a similar extent.31,99
Once within the macrophage, virulent strains of R. equi continue to multiply within the membrane-enclosed vacuoles, whereas avirulent strains multiply at low levels initially and cease to grow after approximately 6 hours.8,32 The means by which virulent R. equi avoids the killing mechanisms of macrophages are not fully understood. Initially, a lack of phagosome-lysosome fusion was reported.97,98 It now appears that there is a complex alteration of the normal phagocyte maturation process, which is at least in part regulated by the virulence plasmid. Based on studies with murine macrophages, Toyooka et al.99 reported that phagosome-lysosome fusion occurred with both virulent and avirulent R. equi, but that the phagolysosomes containing virulent organisms were not as acidic as those containing avirulent organisms. Also in murine macrophages, Fernandez-Mora et al.100 demonstrated that R. equi–containing vacuoles progressed normally through the early stages of phagosome maturation, but that maturation was ultimately blocked. This block in maturation was regulated by the presence of the virulence plasmid. Strains with the virulence plasmid maintained a nonacidified compartment for 48 hours, whereas strains lacking the plasmid acidified progressively.
Ultimately, the replication of R. equi within macrophages results in the death of the host cell. In vitro, macrophage degeneration is apparent by approximately 8 hours after infection and is marked by 24 hours.97,98 The cytotoxicity is linked to virulence, although the exact mechanisms are unclear. Virulent R. equi bacteria are cytotoxic for murine macrophages, and the cytotoxicity is strongly upregulated by the presence of VapA-expressing plasmids.31 Isolates with a VapB-expressing plasmid are less virulent and have a lower cytotoxic potential, whereas isogenic strains without a plasmid are avirulent with a very low cyotoxic potential. The cytotoxicity requires viable bacteria. Cell death occurs by necrosis rather than apoptosis.
A number of additional factors may play a role in the pathogenesis of R. equi. The polysaccharide capsule, the presence of lipoglycans, mechanisms of iron acquisition, and the enzyme cholesterol oxidase, termed “equi factor,” may all contribute to virulence.8,15,101 Some evidence suggests that the length of the mycolic acid carbon chain in the cell envelope influences virulence, because strains of R. equi with longer mycolic acid carbon chains are more lethal in mice and cause greater granuloma formation than those with shorter mycolic acid carbon chains.8,102 The modulation of cytokine production by virulent strains may contribute to pathogenicity.103 Virulence is linked to resistance to β-lactam antibiotics in a study of non–plasmid-containing R. equi strains isolated from humans.104,105 However, a study of R. equi isolated from infected foals and soil from affected and control farms found no significant correlation between β-lactam resistance and either the presence of VapA or the disease status.83
Large numbers of cells migrate to the site in response to infection with R. equi, ultimately resulting in granuloma formation. There is an influx of neutrophils, and one early hypothesis stated that a defect in neutrophil function contributed to disease; however, neutrophils from foals are fully bactericidal.94,97,106–108 The precise signals that influence granuloma formation are largely unknown, but it is clear that a complex network of cytokines and chemokines is involved. Granulomas may help contain and control infection but may also contribute to the pathology of disease; they are associated with the secretion of several inflammatory mediators and may allow proliferation of organisms and cause the loss of pulmonary function.109
Immunity to Rhodococcus equi
Role of Innate Immunity
The replication of R. equi in nonactivated resident macrophages is critical to the pathogenesis of disease, but at the same time the killing of R. equi by activated macrophages can be important in the control of infection. Mice with macrophages that are deficient in either of two radical-generating pathways have an increased susceptibility to infection with R. equi.110 Macrophage activation by R. equi in mice involves toll-like receptor 2 (TLR-2).111 This may be significant because human neonates have diminished TLR-induced responses compared with adults, and it has been hypothesized that inefficient TLR-2 signaling may contribute to the enhanced susceptibility of neonatal foals to R. equi.
Neutrophils may also be important in controlling R. equi early in the course of infection. The induction of a neutrophil deficiency in mice during the first week after experimental rhodococcal infection resulted in more severe disease and in significantly increased tissue concentrations of R. equi.112
Role of Antibody
Several lines of evidence support a role for antibody in protection against R. equi, in addition to the observation that the age of onset of disease in foals typically coincides with the waning of maternal antibody. Antibodies to R. equi, including antibodies to the 15- to 17-kDa antigen of virulent R. equi, are widespread in horses and are found in the majority of foals within the first 3 months of age.13,113–116 An inverse correlation may exist between antibody concentrations and disease severity and prevalence.98,114,115,117 After challenge of immune adult horses with virulent R. equi, an antibody response is characterized predominantly by increases in concentrations of immunoglobulin G subtypes IgGa and IgGb, which are important antibody isotypes in opsonization and complement fixation.118 During in vitro experiments by Hietala and Ardans,97 opsonization with R. equi–specific antibody increased phagosome-lysosome fusion and significantly enhanced the killing of R. equi by alveolar macrophages from foals.
Additional evidence supporting a role for humoral immunity in protection against R. equi comes from studies of passive immunization through the administration of hyperimmune plasma (Box 32-1). Several studies investigating the protective effect of hyperimmune plasma have been performed in both mice and foals. The results in mice have been variable, with some studies indicating that hyperimmune serum was not protective against experimental challenge and others indicating a protective effect.28,119,120 Similarly, studies in foals have had varied results. The administration of hyperimmune plasma does not always have a significant protective effect, but it does prevent or reduce the severity of pneumonia in foals that are either experimentally or naturally R. equi infected.73–75,121–125 Hyperimmune plasma does not alleviate clinical signs or alter the course of the disease when administered to foals 7 days after experimental challenge with R. equi; thus it appears that the protective components in immune plasma are primarily effective in the prevention of infection.122
It is uncertain which specific components of hyperimmune plasma are responsible for enhancing protection against R. equi. In addition to immunoglobulin, hyperimmune plasma contains a number of substances, including fibronectin, interferon, complement factors, and cytokines. In a study by Hooper-McGrevy et al.,126 the same degree of protection was provided by purified immunoglobulin specific for VapA and VapC as by hyperimmune plasma, suggesting that immunoglobulin was the primary component of hyperimmune plasma that conferred protection, and that specific antibodies against VapA and VapC were protective. In contrast, Perkins et al.123 found no difference in the incidence and severity of disease after experimental challenge with R. equi in colostrum-deprived foals given either normal equine plasma or hyperimmune plasma. Because the survival rate for foals in both groups was approximately 70% without antibiotic therapy, both normal and hyperimmune plasma were thought to provide some protective effect, although there was no untreated control group. The researchers therefore concluded that either only a small amount of antibody was sufficient to enhance protection, or that factors in the plasma other than immunoglobulin were responsible for the protective effect.
Several studies have evaluated the protection provided by the ingestion of colostrum from mares immunized against rhodococcus. Martens et al.127 found that the passive immunization of foals by ingestion of colostrum from mares immunized with live R. equi did not provide protection against experimental challenge. Similarly, in field studies by Madigan et al.,74 in which pregnant mares were immunized with an R. equi bacterin, and by Prescott et al.,124 in which pregnant mares were immunized with a VapA extract, foals were not protected from natural infection with R. equi. In contrast, in a field study by Cauchard et al.,128 in which pregnant mares were immunized with either VapA protein antigen or whole killed R. equi, vaccination did appear to provide protection. In general, the protection provided by colostrum has not been as consistent as that with hyperimmune plasma. One possible explanation for this difference is that factors in plasma other than antibody significantly contribute to protection. An alternative explanation is that the isotypes of antibody necessary for protection may not be present in high concentrations in colostrum. Furthermore, the specific immunogens or immunization protocols employed may not induce sufficient concentrations of antibody or antibody with the appropriate antigen specificity.
Role of Cellular Immunity
Considerable evidence supports the importance of cell-mediated immunity in the control of R. equi infection. Much of this evidence comes from a mouse model of rhodococcal disease, although there are some data in horses. In mice, both CD4+ and CD8+ T lymphocytes contribute to immune clearance of R. equi from the lung.129–131 Mice that lack CD8+ but not CD4+ T lymphocytes are able to clear infection completely in the period studied, whereas mice lacking CD4+ but not CD8+ T lymphocytes are able to decrease bacterial numbers significantly in the lung.
Studies have emphasized the role of CD4+ T lymphocytes because these cells appear to be both necessary and sufficient for clearance of R. equi.129–131 CD4+ T lymphocytes are further characterized as T helper 1 (Th1) or T helper 2 (Th2) cells, depending on their cytokine secretion patterns.132 These CD4+ subtypes have been best defined in mice. CD4+ Th1 cells secrete primarily interferon gamma (IFN-γ), a potent activator of macrophage microbicidal activity. In comparison, CD4+ Th2 lymphocytes secrete predominantly the interleukins IL-4, IL-5, and IL-13, which potentiate the humoral immune response. In mice, secretion of IFN-γ by CD4+ Th1 lymphocytes appears to be absolutely required for clearance of R. equi.130 Adoptive transfer of a R. equi–specific CD4+ Th1 cell line mediates clearance in immunodeficient nude mice that normally are unable to control pulmonary bacteria.131 In contrast, nude mice that receive an R. equi–specific CD4+ Th2 cell line are unable to clear bacteria and develop prototypic pulmonary lesions.
CD4+ T lymphocytes are critical in the control of rhodococcal infection, but CD8+ T lymphocytes contribute as well. These CD8+ cells probably act through multiple mechanisms to decrease bacterial numbers. One of their effector functions is the ability to produce IFN-γ. Another possible important function is the recognition and lysis of R. equi–infected cells, as demonstrated for the related pathogen, Mycobacterium tuberculosis.133,134
Limited data in horses support the observations in mice that protective immunity to R. equi involves both CD4+ and CD8+ T lymphocytes. Adult horses challenged intrabronchially with virulent R. equi do not develop clinical disease and effectively clear bacteria from the lung in association with increased numbers of CD4+ and CD8+ lymphocytes at the site of infection.135 T lymphocytes from bronchoalveolar lavage (BAL) fluid of challenged horses proliferate when stimulated with R. equi antigen, and both CD4+ and CD8+ T lymphocytes from the site express IFN-γ. In addition, T lymphocytes from the blood and BAL fluid of adult immune horses have R. equi–specific cytolytic activity.136 These cytotoxic T lymphocytes (CTLs) appear to be primarily CD8+ and have the ability to kill in a major histocompatibility complex (MHC) class I, unrestricted fashion.
CLINICAL FINDINGS
Clinical disease caused by R. equi is most common in foals 3 weeks to 6 months of age, with signs most often developing before age 4 months.73,76,77,79,137 Respiratory tract disease occurs most often, although other systems may be affected as well, either independently or in conjunction with lung involvement. In a retrospective study of 61 foals seen at a referral center for rhodococcal pneumonia, the prevalence of extrapulmonary disorders was 66%, although this percentage is higher than what some clinicians recognize.138 General clinical signs often associated with rhodococcal disease, regardless of the site of infection, include fever, lethargy, and decreased appetite.
Rhodococcal infection may remain subclinical in some cases. When clinical disease does develop, it is often insidious in nature. Because of the foal’s ability to compensate and the slow spread of infection, early clinical signs are often subtle, making the disease difficult to detect. Although infection is generally chronic, clinical signs often appear acutely when the disease becomes severe, leading to the description of an “acute on chronic” disease. A small percentage of foals exhibit a subacute form of the disease, in which they may be found dead or in acute, severe respiratory distress with high fever. These foals often have a poor prognosis.91
Pulmonary Disease
The most common manifestation of R. equi infection in foals is chronic pyogranulomatous bronchopneumonia with abscessation and associated suppurative lymphadenitis.76,79,80,137 Zink et al.137 confirmed the presence of suppurative pneumonia in 115 of 131 cases of R. equi infection. In a review of 40 cases of equine lung abscesses, 32 cases were identified in foals 6 months of age or younger, with R. equi cultured from 13 of 34 cases and Streptococcus zooepidemicus cultured from 20 of 34.139 Occasionally, R. equi is cultured with other pathogens, including Pneumocystis carinii.140 Rhodococcus equi has rarely been cultured from foals with the syndrome of bronchointerstitial pneumonia and respiratory distress, but it is not believed to be the primary cause of this condition.141
Findings on auscultation of the lung are variable and often do not correlate well with the severity of pneumonia. The sensitivity of auscultation may be enhanced by inducing the foal to breathe deeply using a rebreathing bag or cupping a hand over the nostrils for a few seconds if the foal is stable enough to tolerate this procedure. Inspiratory and expiratory crackles and wheezes may be audible and are often most prominent cranioventrally. In some cases, only large airway sounds are present, suggesting consolidation. With severe consolidation or extensive peripheral abscess formation, lung sounds may be decreased. This may also indicate pleural effusion, although effusion is seen only occasionally in association with rhodococcal pneumonia.142 In one case, respiratory distress was identified in association with a focal mediastinal abscess without concurrent pulmonary involvement.143 Thoracic percussion, as well as ancillary diagnostic aids such as radiography and ultrasonography, may help detect areas of consolidation, abscessation, or pleural fluid.
Abdominal Disease
Enterocolitis, typhlitis, abdominal abscesses, peritonitis, and adhesions have been reported in association with R. equi.* In the review of 131 cases by Zink et al.,137 approximately 50% of foals with R. equi that had bronchopneumonia on necropsy also had intestinal lesions, and an additional 4% of foals had intestinal lesions without pneumonia. However, of the foals with intestinal lesions, only 38% had any history or clinical signs related to intestinal disease.
Nonseptic Polysynovitis
An immune-mediated polysynovitis has been documented in approximately one third of R. equi cases77,138 (Fig. 32-2). Although all joints may be affected, this condition appears to be most common in the tibiotarsal and stifle joints.77 Degree of joint effusion is variable, but unlike cases of septic arthritis, there is no or minimal lameness. Evaluation of synovial fluid reveals a nonseptic mononuclear pleocytosis with no bacterial growth.77,146,147 Histologic examination of the synovium reveals a lymphoplasmacytic synovitis.146,147 In horses with nonseptic arthritis, the effusion generally resolves as the rhodococcal infection clears.
It is hypothesized that the presence of immune complexes in the joint leads to an acute reactive arthritis.146 Immunoglobulin was demonstrated within the synovial membrane using fluorescein-labeled antiequine IgG in three affected foals.147 Additionally, rheumatoid factor activity, which results from antibodies directed against the Fc portion of autologous or heterologous immunoglobulin, was identified in the synovial fluid of a foal with R. equi pneumonia and reactive arthritis.146
Bone and Joint Disease
Septic arthritis and osteomyelitis may be observed either alone or with other signs of rhodococcal disease.76,137,148–151 These conditions can be distinguished from immune-mediated polysynovitis by the degree of lameness along with cytologic evaluation and culture of joint fluid or aspirates. Some foals have an associated cellulitis. Treatment includes aggressive local therapy in addition to systemic antibiotics.
Several cases of R. equi vertebral osteomyelitis have been reported, and the question has arisen whether this condition is becoming more common.152–157 In general, early signs of vertebral osteomyelitis may include a stiff gait, reluctance to move, and pain on palpation. However, the diagnosis is usually not made until the infection extends to the epidural space, causing signs of spinal cord or nerve root compression, such as paresis, ataxia, paralysis, or cauda equina syndrome. The specific signs will vary depending on the severity and site of the lesion. Although radiography may be useful in the diagnosis, in four of six cases, radiographs were normal despite the presence of extensive lesions on necropsy.154,155,157 Therefore, nuclear scintigraphy, computed tomography, or magnetic resonance imaging may be indicated.
Diseases of Other Body Systems
A variety of other conditions have been sporadically recognized in association with R. equi.66,67,76,138 These include ulcerative lymphangitis, cellulitis, subcutaneous abscesses, abscesses of the submandibular lymph nodes, nephritis, renal abscesses, and hypopyon (Fig. 32-3). Some cases of cellulitis without any internal involvement may be secondary to wounds or damage to the skin by the larvae of Strongyloides westeri.158 Hepatitis, cholangitis, and hepatoencephalopathy as well as uveitis and panophthalmitis have also been reported.76,137,138,159,160
R. equi has rarely been isolated from aborted equine fetuses and infertile mares.137,161–165 The significance of isolating the organism has been unclear in some cases, but R. equi has been confirmed as a cause of placentitis and abortion with fetal pneumonia.164,165 However, despite the frequent occurrence of R. equi in equine feces and soil samples, it is rarely recognized as a cause of equine abortion.166,167
Infection in Adult Horses
Rhodococcal disease is uncommon in adult horses because most adults are immune to infection. However, sporadic cases have been reported.137,168–173 As in foals, the most common clinical signs are related to suppurative bronchopneumonia (Fig. 32-4). Occasionally, pleuritis may be recognized. Other reported signs include intestinal disease, lymphadenitis, wound infections, and osteomyelitis. In both cases in which the organism isolated from adult horses was assessed, the virulence plasmid was present.170,171 It is thought that adult horses with R. equi infection are immunocompromised, and although the immune status has not been assessed in every case, immunodeficiency was reported in two cases.169,173
DIAGNOSIS
Cytology
The presence of intracellular gram-positive pleomorphic rods on cytologic evaluation of fluid specimens supports a diagnosis of rhodococcal infection (Fig. 32-5). However, the organisms may be present in low numbers and may be difficult to detect. Sweeney et al.77 reported that organisms were seen on cytology of tracheobronchial fluid in 61% of 48 culture-positive foals, whereas in 22% of these foals, no bacteria were seen, and in 17%, only bacteria other than R. equi were identified. An indirect fluorescent antibody (IFA) technique has been described for acetone-fixed specimens; 33 of 53 (62.3%) tracheal aspirates from foals with experimentally induced rhodococcal pneumonia had a positive IFA result.174
Culture
Culture and subsequent phenotypic analysis of the isolate by classic morphologic and biochemical tests has been the “gold standard” for the diagnosis of R. equi. Typically, the organism is cultured from a tracheobronchial aspirate, which may be obtained by a variety of techniques.174,175 When collecting the sample, the clinician must consider stress to the patient because some foals are in severe respiratory distress. Other samples, such as joint fluid or peritoneal fluid, can be cultured as appropriate based on the case.
Colonies of R. equi will usually appear on solid media within 48 hours of aerobic culture, although in some cases, longer incubation is necessary, especially for samples collected from foals that have been treated with antibiotics.3,76,174,176 Occasionally, the organism may be isolated only under anaerobic conditions after antimicrobial therapy.176 Colonies of R. equi generally appear irregularly round, smooth, semitransparent, and mucoid (Fig. 32-6). They typically have a characteristic salmon-pink color, which may not develop until 4 to 7 days in culture. Other pathogens may be isolated concurrently with R. equi.77,177
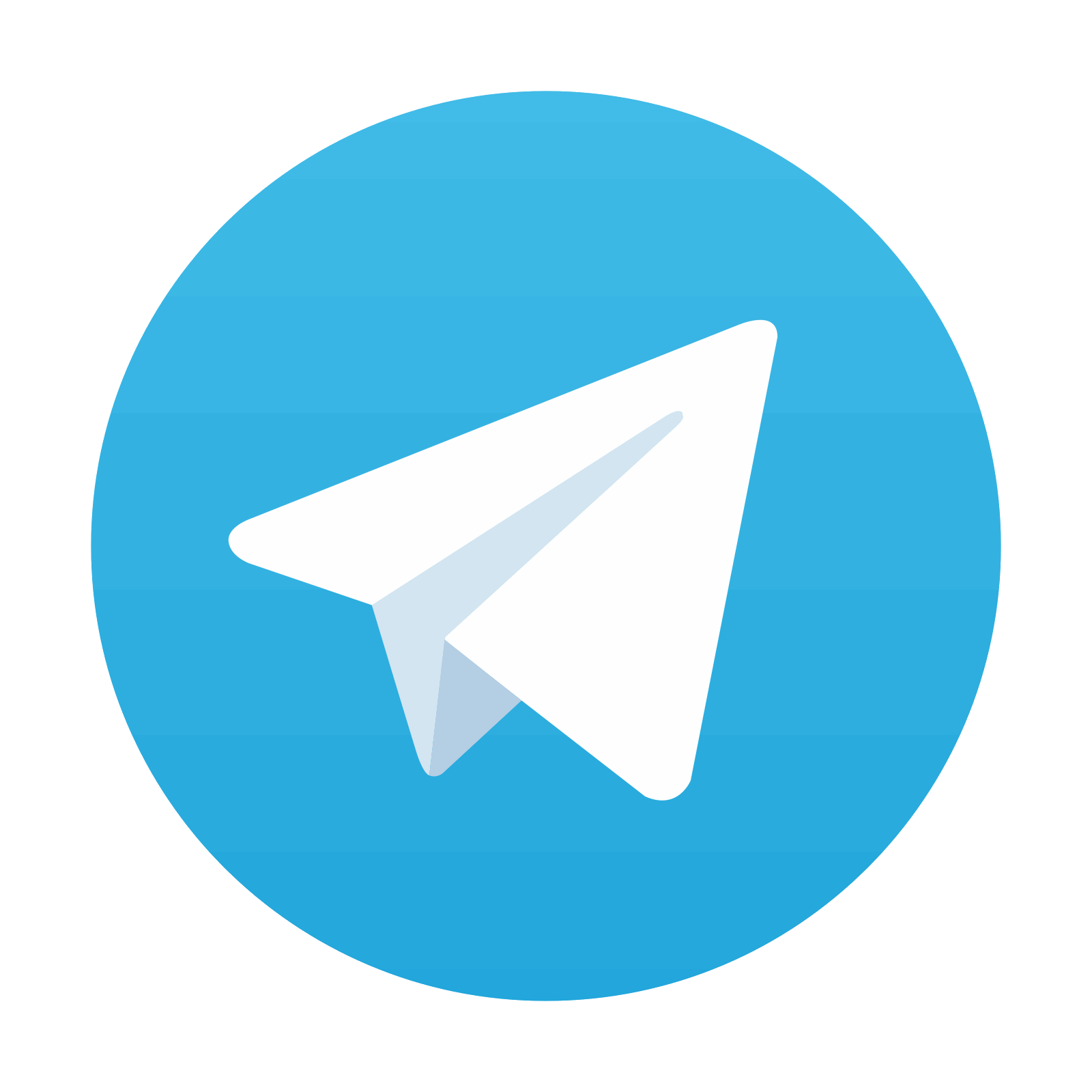
Stay updated, free articles. Join our Telegram channel

Full access? Get Clinical Tree
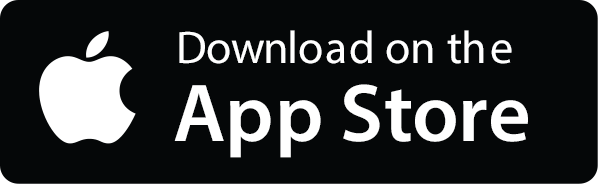
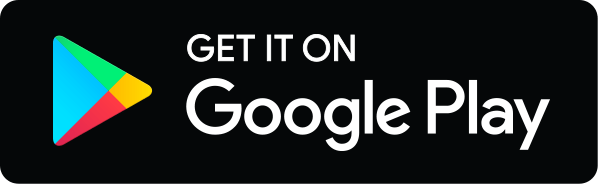